Advanced Techniques for DNA and RNA Purification
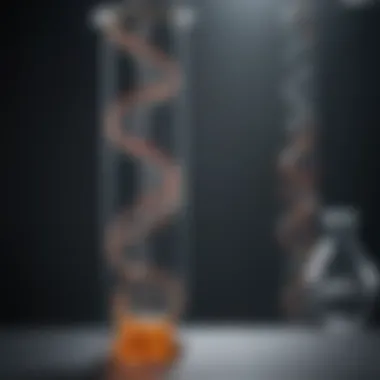
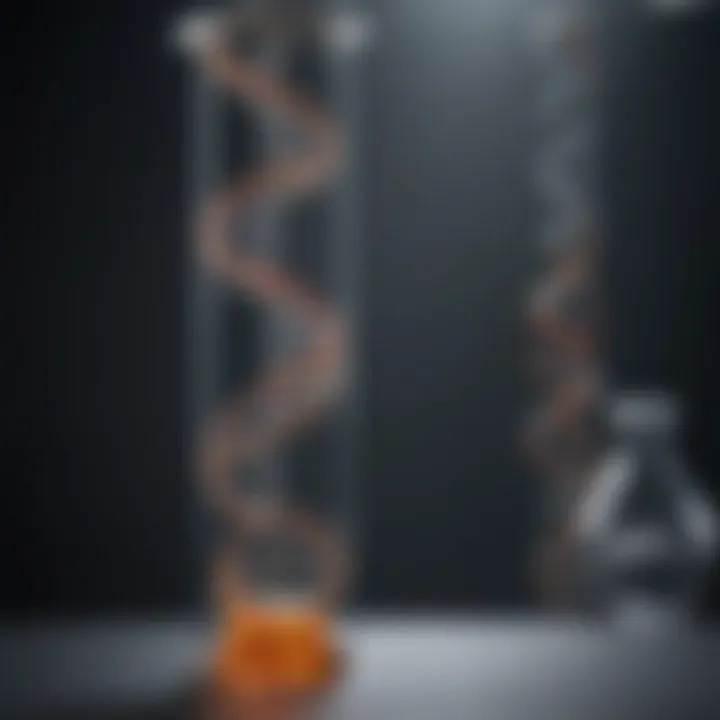
Research Highlights
Key Findings
DNA and RNA purification techniques are foundational in molecular biology. Recent studies reveal that advancements in extraction methods significantly improve nucleic acid yield and purity. Key findings indicate that using silica-based columns in combination with elution buffer optimization enhances the quality of isolated samples. Additionally, novel magnetic bead-based methods provide increased throughput and efficiency, making them more favorable in laboratory settings.
Implications and Applications
The purity and yield of extracted DNA and RNA play critical roles in genetic applications, including sequencing, cloning, and gene expression analysis. High-purity nucleic acids lead to more reliable results, thus improving the overall reliability of molecular biology research. These techniques have wide-ranging implications in medical research, biotechnology, and forensic science. By understanding the intricacies of purification methods, professionals can tailor their approach, thus obtaining better results in their experiments.
Methodology Overview
Research Design
The research design for DNA and RNA purification focuses on a systematic approach that begins with sample collection. Specific protocols are developed based on the type of sample, whether it is a biological specimen or an environmental sample. Factors such as expected yield, purity criteria, and downstream applications inform the choice of extraction method.
Experimental Procedures
- Sample Preparation: Biological samples need appropriate processing, such as homogenization or freeze-thaw cycles, to disrupt cells and release nucleic acids.
- Extraction Methods: Various methods include:
- Purity Assessment: The purity of extracted nucleic acids is assessed using spectrophotometry or gel electrophoresis. It is essential to ensure that contaminants are minimal, which can hinder downstream applications.
- Yield Measurement: Determining the concentration and yield of nucleic acids is crucial for evaluating purification success. Techniques include Qubit assays and NanoDrop measurements.
- Silica-based column extraction
- Phenol-chloroform extraction
- Magnetic bead separation
"The quality of DNA and RNA purification directly correlates with the reliability of subsequent experiments."
Consistency across these procedures improves reproducibility in molecular biology experiments. A clear understanding of methodologies enables researchers to achieve their specific objectives effectively.
Prologue to DNA and RNA Purification
In the realm of molecular biology, the purification of nucleic acids, specifically DNA and RNA, remains a foundational process. This section elucidates the importance of mastering these purification techniques and highlights their implications across various research applications. Understanding the methods for effectively isolating DNA and RNA is crucial, as it sets the stage for subsequent analyses and experimental procedures.
The significance of purification lies not just in the extraction of nucleic acids but in the preservation of their quality and integrity. Purified DNA and RNA are central to numerous applications such as genetic engineering, forensic analysis, and forensic genetics, where the reliability of results heavily relies on nucleic acid purity. Therefore, researchers must consider factors like purity, yield, and contaminant levels during purification processes.
"The initial step in any molecular analysis is the quality of nucleic acids extracted."
Furthermore, various purification methods bring distinct advantages and limitations, emphasizing the necessity to choose the right approach based on the specific requirements of a study. Knowledge of these methods allows researchers to enhance the reproducibility and accuracy of their experiments. As the field of molecular biology evolves, the advancements in purification technologies continue to push the boundaries of what can be achieved with nucleic acids, urging researchers to stay informed about the latest techniques and protocols.
The following sections will provide a comprehensive overview of nucleic acid purification, delve into fundamental concepts, and analyze common techniques that underlie this crucial scientific practice.
Fundamental Concepts
Understanding the fundamental concepts of DNA and RNA purification is paramount for effective molecular biology research. These concepts lay the groundwork for selecting appropriate techniques and assessing their efficiency. The purity of these nucleic acids directly influences downstream applications, such as genetic analysis and sequencing. Each purification method has unique trade-offs involving cost, time, and quality, necessitating a thorough grasp of these elements before embarking on any experimental protocol.
DNA and RNA Structures
DNA and RNA are biopolymers composed of nucleotides, which are the building blocks for genetic material in all living organisms. DNA, or deoxyribonucleic acid, typically exists as a double helix with complementary strands linked by base pairing. Its structure allows for the stable storage of genetic information. On the other hand, RNA, or ribonucleic acid, is usually single-stranded and plays more dynamic roles in cellular functions, including coding, decoding, and regulation of genes.
The chemical differences between DNA and RNA influence their respective purification processes. For example, the presence of ribose in RNA makes it more susceptible to hydrolysis than DNA, which contains deoxyribose. This inherent instability necessitates different safeguards during RNA extraction, emphasizing the importance of understanding these structural differences when choosing purification techniques.
The Role of Purity and Yield
In nucleic acid purification, achieving high purity and yield is crucial. Purity refers to the absence of contaminants, while yield indicates the amount of nucleic acid recovered from the source material. Both factors significantly impact the quality of subsequent analyses, including qPCR, sequencing, and cloning.
Ensuring high purity in nucleic acid samples minimizes the risk of interference in experimental outcomes.
Inadequate purity can lead to false results, misinterpretation of data, and overall experimental failure. Conversely, low yields can restrict the usability of samples, limiting the number of experiments and potentially wasting valuable resources.
Achieving an optimal balance between purity and yield requires careful consideration of the chosen purification strategy. Some methods may prioritize yield over purity or vice versa. Therefore, researchers must consider the specific requirements of their downstream applications when selecting an extraction technique, ensuring that the purified nucleic acids meet the necessary standards.
Common Techniques for DNA Purification
The purification of DNA is a critical step in molecular biology research and applications. Various techniques have been developed over the years, each with its specific advantages and limitations. Understanding these common methods is vital for ensuring the quality and yield of purified DNA. It directly impacts downstream applications such as cloning, sequencing, and genetic analyses. Each technique presents a unique approach that meets diverse experimental needs, contributing to the reliability of molecular data.
Phenol-Chloroform Extraction
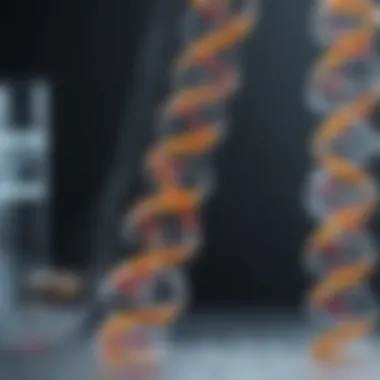
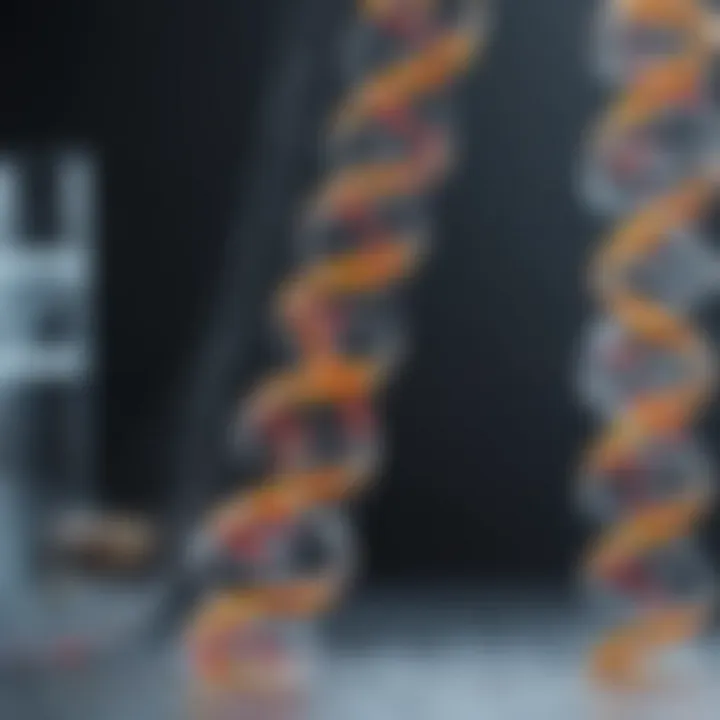
Phenol-chloroform extraction is a traditional and widely utilized method for DNA purification. This technique involves the use of organic solvents, typically a mixture of phenol and chloroform, to separate nucleic acids from proteins and other contaminants. The DNA is extracted into the aqueous phase, while proteins and lipids typically remain in the organic phase. The main advantage of this method is its ability to effectively remove proteins and some contaminants, yielding high-purity DNA suitable for various analyses.
However, this method is not without its drawbacks. The process involves hazardous chemical reagents that require careful handling and appropriate safety measures. Moreover, efficiency can be variable, depending on sample types and the exact protocol used. While phenol-chloroform extraction remains a gold standard, it is often paired with further purification steps to enhance yield and quality.
Silica-Based Methods
Silica-based methods have gained popularity for their simplicity and effectiveness in DNA purification. These methods exploit the property of silica to bind DNA in the presence of high salt concentrations. Once bound, contaminants can be washed away using an ethanol solution, followed by elution of the DNA in a low-salt buffer or water.
The advantages of silica-based methods include their speed and scalability, making them well-suited for high-throughput applications. Furthermore, they typically provide good yields and purity. These methods are common in commercial kits, which often standardize protocols for ease of use. However, slight variations in protocols can lead to different outcomes, so careful adherence to the guidelines is essential.
Colony PCR and its Applications
Colony PCR is a technique that allows rapid screening of cloned DNA in bacterial colonies. It is a powerful method for verifying the presence of a specific DNA fragment inserted into a plasmid. The process is straightforward; it involves picking a colony and using it directly in a PCR reaction without the need for prior purification. This efficiency makes colony PCR an attractive choice for molecular cloning workflows.
While this method is beneficial for quick assessments, it does come with some limitations. The success of colony PCR relies heavily on the quality of the colony itself and the efficiency of the PCR reaction. Additionally, results can sometimes yield false positives, necessitating further validation through sequencing or additional assays. Nevertheless, the ability to quickly analyze multiple colonies increases throughput and reduces assay time, proving valuable for many researchers.
"Efficiency in DNA purification can streamline your research process, making techniques like phenol-chloroform extraction, silica-based methods, and colony PCR indispensable tools for molecular biologists."
These common techniques for DNA purification provide a foundation for researchers aiming to achieve high-quality DNA suitable for various applications. Understanding the nuances of each method allows for informed choices, optimizing both purity and yield.
Common Techniques for RNA Purification
The purification of RNA is a critical step in many molecular biology applications. The effectiveness of RNA purification directly influences the quality and reliability of downstream experiments. Purified RNA is essential for various purposes, including gene expression analysis, sequencing, and functional studies. High-quality RNA must be free of contaminants that could interfere with such processes. Therefore, it is important to employ effective techniques that ensure maximum purity and yield.
Guanidinium Thiocyanate Method
The Guanidinium Thiocyanate method is one of the most widely used techniques for RNA purification. This method utilizes the chaotropic property of guanidinium thiocyanate, which disrupts hydrogen bonds in nucleic acids and proteins, thereby denaturing them. The primary advantage of this method is its ability to effectively extract RNA from various biological samples, including tissues, cells, and bacterial cultures.
In practice, samples are lysed in a solution containing guanidinium thiocyanate. This step is followed by an organic extraction or precipitation process involving alcohol, such as isopropanol or ethanol. The separation of RNA from DNA and proteins occurs during the centrifugation step, resulting in a clear, purified RNA solution. Several important considerations with this method include:
- Compatibility: It works well with a broad range of sample types.
- Ease of Use: The protocol is straightforward, making it accessible for laboratories with varying levels of expertise.
- Efficiency: It can yield high-quality RNA suitable for sensitive applications.
Despite its advantages, the Guanidinium Thiocyanate method can introduce some challenges, particularly regarding the handling of toxic reagents. Proper safety measures and disposal procedures are essential in any laboratory performing this technique.
Silica Column Methods for RNA
Silica column methods exploit the tendency of RNA to bind to silica in the presence of a specific salt concentration. These methods are known for their efficiency and ease of use, making them a preferred choice for many researchers. After cell lysis, the resultant mixture is applied to a silica-based column, allowing RNA to bind while impurities are washed away.
A critical advantage of silica column methods is the rapidity of purification. The entire process can often be completed in under an hour, which is beneficial in high-throughput settings. Key aspects of this method include:
- Selective Binding: Enhanced purity due to selective binding and washing processes.
- Scalability: Easily adaptable for various sample sizes and types.
- Cost-Effectiveness: Generally cost-efficient, with commercially available kits that simplify the process.
However, it is essential to carefully follow manufacturer instructions to ensure optimal performance. Overloading the column can result in low yield or contamination, which could compromise subsequent applications. Silica column methods stand as a reliable option in RNA purification, contributing significantly to research accuracy and efficiency.
Research Implication: "Using dependable purification techniques is fundamental to successful RNA-based analyses."
In summary, understanding various techniques such as the Guanidinium Thiocyanate method and silica column methods provides invaluable insights for effective RNA purification. Researchers must weigh the pros and cons of each to select the best approach for their specific experiments.
Quality Control in Nucleic Acid Purification
Quality control is a critical aspect in the context of nucleic acid purification. The quality and integrity of DNA and RNA are paramount for the success of subsequent analyses and applications. In research labs, where precision is essential, ensuring high purity and correct concentration can significantly influence results. Key elements of quality control include evaluating the purity of nucleic acids, determining their concentration, and identifying common contaminants. Each of these aspects plays an integral role in maintaining the reliability of molecular biology experiments.
Evaluating Purity and Concentration
Measuring the purity and concentration of nucleic acids is a foundational step in quality control. This process often involves spectrophotometric analysis. Using a spectrophotometer, researchers can assess the absorbance of samples at specific wavelengths, typically 260 nm and 280 nm. The ratio of these measurements can indicate nucleic acid purity. A ratio of 1.8 is generally sought for pure DNA, while a ratio of approximately 2.0 is preferred for RNA.
Key points in evaluating purity include:
- Spectrophotometry: Useful for quick assessments.
- Agarose Gel Electrophoresis: Can visualize nucleic acid integrity.
- Fluorometric Methods: More sensitive for low-concentration samples.
Accurately determining concentration also helps when preparing nucleic acids for downstream applications like cloning or sequencing. A standard practice is to convey concentration in ng/µl or µM, depending on the experimental requirements.
Common Contaminants and Their Effects
Despite the best purification methods, contaminants can still affect nucleic acid quality. Common contaminants include phenol, enzymes, salts, and residual proteins. These substances can hinder enzymatic reactions and interfere with assays. For example, phenol contamination might lead to DNA degradation, while high salt concentrations can precipitate the nucleic acids, impacting downstream applications.
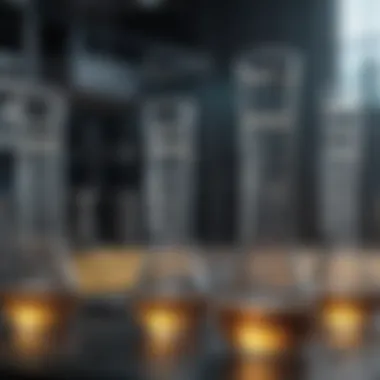
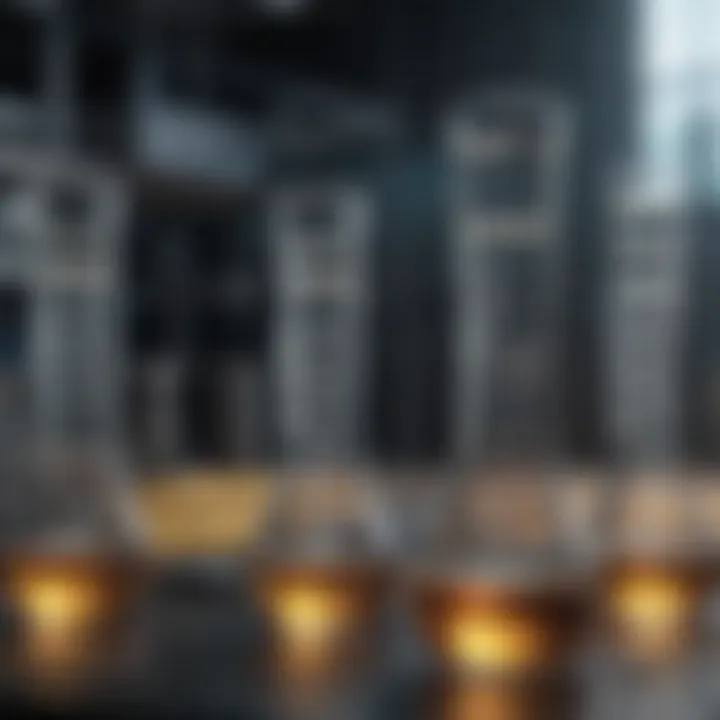
Recognizing contaminants can involve:
- Assessing the A260/A280 ratio: Ratios outside the standard thresholds may indicate contamination.
- PCR Performance: Poor amplification can suggest the presence of inhibitors.
Researchers need to remain vigilant about potential contaminants to ensure the success of their experiments. Knowing the specific effects of various contaminants can guide choosing methods and best practices in nucleic acid purification.
"Quality control is not just a good practice; it is a necessity for reliable and replicable scientific results."
In summary, integrating structured quality control measures in nucleic acid purification can vastly improve experimental outcomes. As the field of molecular biology evolves, these practices will remain central to producing high-quality DNA and RNA for various applications.
Advancements in Purification Technologies
Advancements in purification technologies play a crucial role in enhancing the efficiency and effectiveness of DNA and RNA purification processes. With continuous developments in methodologies, researchers can achieve higher purity levels and yields in their nucleic acid extractions. Trends show an increasing shift toward automation and innovative materials, which help reduce time and resource expenditure in laboratories. The changes are not just incremental but reflect a significant leap forward for molecular biology applications. This section will delve into the key advancements, exploring both magnetic bead-based systems and automated extraction techniques.
Magnetic Bead-Based Systems
Magnetic bead-based systems have emerged as a cutting-edge method for nucleic acid purification. These systems utilize magnetic particles that can bind selectively to nucleic acids, which facilitates easy separation from contaminants. The primary benefit of this approach lies in its efficiency and ability to process multiple samples simultaneously. Researchers often find that these systems reduce the risk of cross-contamination compared to traditional methods.
Key elements to consider with magnetic bead-based systems include:
- Speed: The extraction process is typically faster compared to conventional methods.
- Versatility: They can be used for a variety of sample types, including blood, tissue, and cell cultures.
- Scalability: Suitable for both small- and large-scale applications, making them adaptable for different research needs.
- User-Friendly: Many kits come with standardized protocols, making them accessible even to novices in molecular biology.
"Magnetic bead technology has revolutionized nucleic acid purification, allowing for increased automation and reproducibility."
Automated Nucleic Acid Extraction
Automated nucleic acid extraction is another notable advancement that addresses the limitations of manual techniques. Automation in purification processes minimizes the likelihood of human error. These systems often come equipped with software that standardizes extraction protocols, resulting in more consistent outcomes.
Benefits of automated nucleic acid extraction include:
- High Throughput: Automation allows for the simultaneous processing of numerous samples, significantly increasing productivity.
- Consistent Quality: Automated systems provide uniform results by reducing variability associated with manual handling.
- Labor Savings: With less hands-on time required, researchers can focus on other critical tasks in their projects.
- Comprehensive Integration: Many automated systems integrate quality control measures, ensuring that purity and yield are constantly monitored during the extraction process.
Comparative Analysis of Purification Methods
Understanding the various methods of nucleic acid purification is crucial for students, researchers, and professionals in molecular biology. The comparative analysis of purification methods allows one to evaluate their strength and weaknesses, guiding the selection of the optimal technique for specific applications. In a field where precision and reliability of results are paramount, comprehending the nuances of each method influences downstream results and the overall efficiency of research projects.
Efficiency and Cost Considerations
Efficiency speaks to how well a purification method retrieves DNA or RNA while minimizing contamination. When weighing options, it’s pivotal to consider both extraction efficiency and time investment. For instance, phenol-chloroform extraction is known for its high yield but can be time-consuming and requires handling hazardous chemicals.
Conversely, silica-based methods offer quicker protocols and are safer, as they minimize exposure to toxic solvents. However, they may sometimes yield lower amounts of nucleic acids than traditional methods. Cost is another significant factor. Some purification kits, like those from Qiagen or Thermo Fisher Scientific, can be pricey.
Thus, researchers must weigh the trade-off between cost and efficiency. For large-scale studies, opting for cost-effective methods may be more practical, while high-stakes research may justify the expense of premium kits.
- High-cost options may provide high yield but not always justify the price based on specific project needs.
- Budget-friendly methods may require optimization, such as increased reagent concentrations or additional purification steps.
Suitability for Different Sample Types
Not all purification methods are universally applicable. Each technique has advantages depending on the sample type. For example, the Guanidinium Thiocyanate Method excels in purifying RNA from tissues with high levels of secondary metabolites, while silica column methods are ideal for higher-purity nucleic acids from relatively clean sources like plasmid DNA.
Consideration of sample type dictates the logic behind selecting a purification approach. Different methods respond variably to the unique composition and structure of sample materials. Factors include:
- Source of nucleic acids: Plant tissues, animal tissues, or microbial cells.
- Quality requirements: Next-generation sequencing vs. routine PCR.
- Contaminants present: Proteins, polysaccharides, or salts that can interfere with downstream applications.
"The choice of purification method profoundly influences the reliability and reproducibility of experimental results."
In navigating these options, individuals involved in molecular biology can sharpen their techniques and methodologies, advancing their research capabilities.
Applications of Purified DNA and RNA
Purified DNA and RNA serve as the backbone of modern molecular biology. Their applications span a broad spectrum of scientific disciplines, making them indispensable for a range of studies and practices. Notably, the purity and integrity of nucleic acids directly influence their functionality in various experimental settings. In this section, we will explore key areas where purified DNA and RNA find their practical applications, emphasizing their significance.
Genetic Analysis
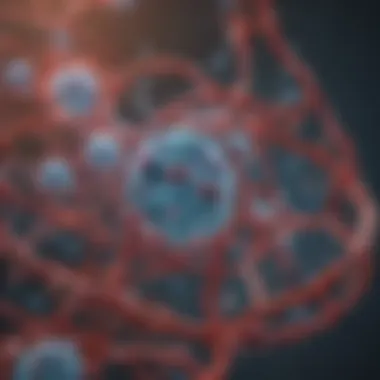
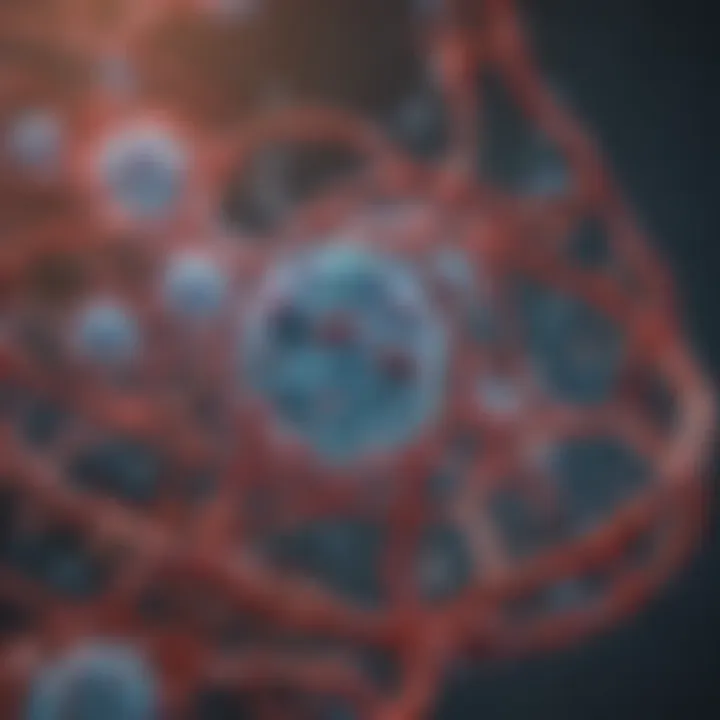
Genetic analysis relies heavily on the quality of purified DNA. Techniques such as polymerase chain reaction (PCR) and restriction fragment length polymorphism (RFLP) are common in genetic studies.
- Applications in forensics: In forensic science, purified DNA allows for reliable identification of individuals. This is essential in criminal investigations and paternity testing.
- Disease diagnosis: Genetic analysis of samples from patients can help identify mutations leading to genetic disorders. This aids in earlier diagnosis and treatment decisions.
- Ancestry studies: Companies offering DNA testing for ancestry purposes rely on high-quality purified DNA to provide accurate lineage information.
The aforementioned applications illustrate the critical role that purified DNA plays in advancing our understanding of genetics and improving healthcare outcomes.
Next-Generation Sequencing
Next-generation sequencing (NGS) provides a comprehensive view of genomes, transcriptomes, and epigenomes. Purified nucleic acids are essential for ensuring the accuracy and reliability of sequencing results.
- High throughput: NGS enables the sequencing of millions of fragments simultaneously, which produces vast amounts of data. Quality purification maximizes the yield of usable sequences, minimizing waste and enhancing data analysis.
- Research discovery: This technology is pivotal in research fields like cancer genomics, where it can reveal mutations specific to individual tumors. This information is crucial for developing targeted therapies.
- Metagenomics: NGS allows for the analysis of genetic material from environmental samples. This has applications in ecological studies, biodiversity assessments, and understanding microbiomes.
Thus, the integrity of purified DNA or RNA critically determines the success of these advanced sequencing applications.
Gene Expression Studies
Gene expression studies focus on understanding how genes are expressed and regulated. Purified RNA, in particular, is vital for such investigations.
- Quantitative PCR: This method requires well-purified RNA to accurately measure the expression levels of specific genes in different biological samples.
- Microarrays: Utilized for monitoring the expression of thousands of genes at once, microarrays depend on high-quality RNA to minimize experimental variability.
- Functional studies: RNA interference techniques, such as siRNA, rely on purified RNA to selectively silence gene expression. This can confirm the role of specific genes in various biological pathways.
By ensuring high purity of RNA, researchers gain valid insights into gene regulation and expression changes, aiding in developmental and disease-related studies.
"The quality of nucleic acids determines the success of downstream applications, underscoring the importance of effective purification methods."
In summary, the applications of purified DNA and RNA are diverse and critical to many aspects of biological and genetic research. From genetic analysis to next-generation sequencing and gene expression studies, the role of nucleic acids cannot be overstated. Their purity directly correlates with the reliability and accuracy of results, which ultimately influences advancements in the field.
Troubleshooting Common Issues
Troubleshooting common issues in DNA and RNA purification is crucial. It allows scientists to maintain sample integrity and improve the success of downstream applications. Understanding potential pitfalls can save time, resources and ensure reliable results. Many problems can arise at any stage of purification. Identifying and addressing these issues can effectively enhance the overall quality of nucleic acids.
Degradation of Nucleic Acids
Nucleic acids are sensitive molecules. They can undergo degradation due to various factors. This degradation can lead to compromised samples, affecting experimental outcomes. Common causes of degradation include exposure to heat, nucleases, and improper storage conditions.
To prevent degradation, consider the following factors:
- Enzyme Inhibition: Keep nucleases at bay by adding inhibitors during extraction.
- Temperature Control: Maintain samples on ice during processing.
- Proper Storage: Store purified nucleic acids at -80°C for long-term.
Furthermore, employ quality control checks post-extraction. Techniques such as gel electrophoresis can help to visualize the integrity of nucleic acids. A sharp band on a gel indicates intact nucleic acids. Conversely, smearing may signal degradation.
"Assessing degradation is an essential step to ensure the reliability of your nucleic acid results."
Low Yields and Contaminants
Low yields of purified DNA or RNA can be frustrating. Several factors contribute to low yields during purification processes. Poor sample handling, inefficient extraction techniques, and contamination are common issues.
Here are some strategies to improve yields:
- Sample Quality: Start with fresh and high-quality samples. Older samples can degrade, leading to lower yields.
- Optimizing Protocols: Tailor purification methods based on sample type. What works for one may not work for another.
- Proper Washing: Ensure thorough washing of columns or magnetic beads to eliminate contaminants that may inhibit yield.
Contaminants can be detrimental to downstream applications. They can interfere with chemical reactions in PCR or sequencing. Regularly monitor for common contaminants like proteins, organic solvents, or residual salts. Utilizing appropriate purification kits can significantly reduce the risk of contamination.
Ending and Future Perspectives
The section on Conclusion and Future Perspectives holds significant value in any discourse regarding DNA and RNA purification. Understanding the summary of findings allows researchers and practitioners to grasp the essence of the various methods available and their distinct advantages. This thorough grasp helps guide decisions in laboratory settings. Thus, it becomes evident that as molecular biology advances, the purification methods will also evolve. This continuous improvement is essential to accommodate new applications and findings in the field of genomics.
In summarizing key insights on purification techniques, one can reflect on the intricate balance between purity, yield, and efficiency. Researchers now have access to numerous techniques ranging from phenol-chloroform extraction to modern automated systems, each with its set of advantages and limitations. Recognizing these facets enables better planning and execution of experiments, ensuring optimal outcomes.
Summary of Key Findings
To reiterate the crucial findings from this article:
- Purification methods such as silica-based and magnetic bead systems have brought significant improvements in yield and quality.
- The importance of purity is underscored by its direct correlation with the reliability of molecular biology applications, such as next-generation sequencing.
- Addressing contaminants effectively is fundamental for the success of downstream applications. Techniques to assess both concentration and purity need to be integrated into routine experimental protocols.
"Quality in nucleic acid purification translates directly to effectiveness in research applications, defining the reliability of results across studies."
The Future of Nucleic Acid Research
Looking forward, the future of nucleic acid research is promising. Emerging technologies in purification are likely to refine existing methodologies further. The integration of artificial intelligence and data analytics is poised to optimize purification protocols based on the specific needs of research projects. Additionally, with the ongoing advancements in biotechnology, we may see new methodologies that significantly improve efficiency and cost-effectiveness.
Developing sustainable practices will be essential. As environmental concerns arise, methods that minimize resource consumption and chemical waste will likely gain importance. This shift may lead to the adoption of green chemistry principles within nucleic acid purification processes.