Exploring Eyecell: Advances in Visual Perception
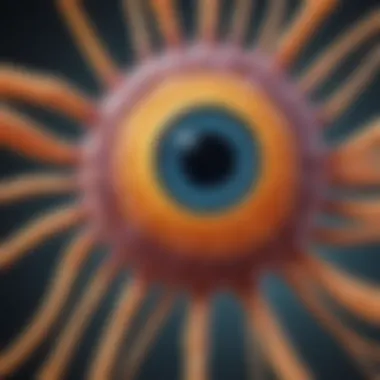
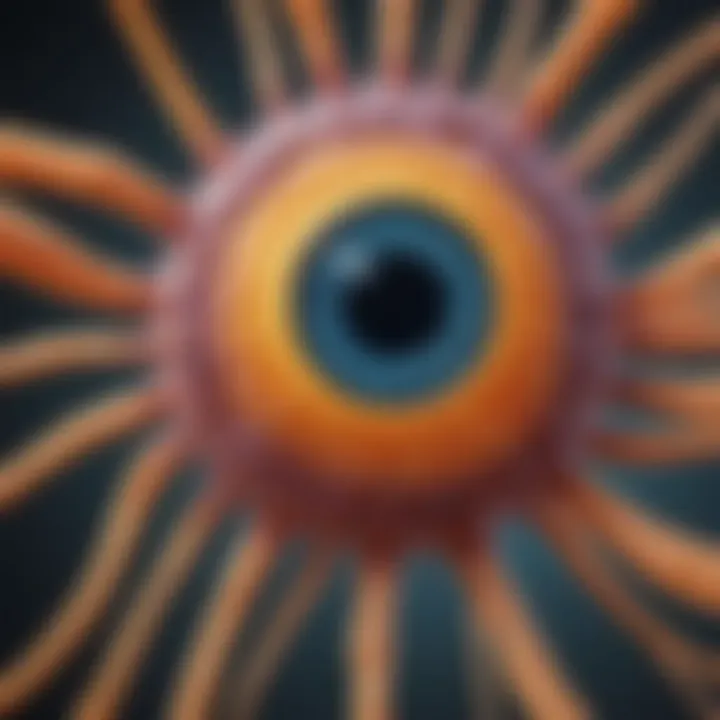
Intro
The study of visual perception is a complex field that intertwines biology, psychology, and technology. Within this domain, the concept of "eyecell" emerges as crucial. It comprises the cellular structures that facilitate our ability to see and interpret the world around us. This article intends to unravel the intricate biology of photoreceptors, which are vital for converting light signals into neural impulses. As research progresses, the implications of these cellular processes stretch far beyond basic understanding.
This exploration encompasses several facets — from the biological mechanics to the technological innovations derived from our comprehension of eyecells. Recent studies reveal both key findings and the potential applications that stem from a deeper understanding of visual processing. By dissecting current literature and ongoing research, this article bridges the divide between complex biological concepts and their practical implications.
Research Highlights
Key Findings
Research on eyecells has highlighted several significant findings:
- Photoreceptor Functionality: Photoreceptors, including rods and cones, play essential roles in capturing light and initiating the visual pathway.
- Cellular Mechanisms: Advances in understanding the molecular bases of photosensitivity have opened new avenues for studying visual impairments.
- Neural Pathways: These findings illustrate the complex pathways that visual information travels from the eye to the brain, involving numerous cellular interactions.
These insights not only deepen our scientific understanding but also hint at ways to manipulate and enhance visual perception in both healthy and compromised systems.
Implications and Applications
The implications of advancing our understanding of eyecells are vast:
- Medicine: Enhanced understanding of photoreceptive cells may lead to better treatments for visual disorders. For example, exploring gene therapy could bring solutions for diseases like retinitis pigmentosa.
- Technology: The insights gained from eyecell research can foster innovations in imaging technology, potentially improving everything from everyday cameras to advanced prosthetic devices.
"Understanding eyecells is not just about biology; it’s about paving the way for future technological advancements and medical treatments that could reshape how we perceive the world."
Methodology Overview
Research Design
Research surrounding eyecells adopts diverse methodologies. Scholars typically employ experimental designs that leverage both in vitro and in vivo approaches to uncover cellular properties and their functions.
Experimental Procedures
Investigations often include:
- Isolation of Photoreceptors: Specific techniques are used to isolate rods and cones for detailed biochemical analysis.
- Electrophysiological Measurements: Methods like patch-clamp recordings assess the electrical properties of these cells, offering insights into how phototransduction occurs.
Overall, the systematic approach of these methodologies facilitates a comprehensive understanding of visual perception and the impact of eyecells on it.
Preamble to Eyecell
The study of eyecells is crucial for understanding how humans and animals perceive the world visually. Eyecells, specifically photoreceptors, are the first step in the complex process of visual perception. This section will provide a foundational understanding of eyecells, their definition, and their historical significance.
Definition and Significance
Eyecells refer to the cellular structures responsible for capturing light and converting it into neural signals. There are two main types of photoreceptors: rods and cones. Rods are more numerous and function well in low light conditions, while cones are responsible for color vision and operate best in bright light.
The significance of eyecells lies in their integral role in vision. They determine how we see shapes, colors, and movements. Without these cells, visual perception would be impossible. Understanding their functionality not only sheds light on basic biological processes but also has profound implications for addressing vision disorders. Research on eyecells helps in developing treatments and enhancing technologies for better visual assistance.
Historical Context
Historically, the study of eyecells can be traced back to the 19th century when scientists began to investigate the anatomy of the eye. Pioneering researchers like Johannes Kepler and Hermann von Helmholtz contributed to the understanding of how light interacts with the eye.
In the 20th century, advances in microscopy and cellular biology enabled researchers to observe photoreceptors in more detail. This allowed for breakthroughs in understanding how these cells function. Modern research continues to build on this foundation, using innovative techniques to explore the complexities of visual perception, helping to facilitate further advancements in various fields such as neuroscience and optometry.
"Eyecells are not just passive receptors of light; they are dynamic entities that play a central role in how we engage with our environment."
This historical perspective emphasizes the ongoing dialogue between different scientific disciplines in unraveling the mysteries of vision, highlighting the interdisciplinary nature of eyecell research.
Anatomy of Eyecells
The anatomy of eyecells is a crucial element in understanding visual perception. This aspect covers the different structures and types of cells responsible for translating light into signals that the brain interprets as images. Without a firm grasp of this anatomy, one cannot truly appreciate how vision works or the implications for advancements in this field. The study of eyecells reveals how intricate and specialized these structures have evolved to optimize human sight and its inherent challenges.
Types of Photoreceptor Cells
Photoreceptor cells play a pivotal role in capturing light stimuli. They are broadly classified into two categories: rods and cones, each with unique features that contribute to our visual experiences.
Rods
Rods are photoreceptor cells that are highly sensitive to light, making them essential for night and peripheral vision. A key characteristic is their ability to operate in low-light conditions which is why they are so significant when discussing visual perception in dim environments. They have a unique structure that allows them to contain a high amount of the photopigment rhodopsin, enhancing their light-capturing efficiency.
The advantage of rods is their ability to detect movement and ensure visibility in dark conditions, but they have limitations. They do not provide color information, making them inadequate for tasks requiring color differentiation. This limitation underlines the necessity of understanding both rods and cones in the larger scope of visual perception.
Cones
Cones are the second type of photoreceptor cells and are crucial for color vision and visual acuity. They are primarily active in well-lit conditions and enable humans to perceive a wide spectrum of colors. A significant feature of cones is their diverse types, which include those sensitive to short, medium, and long wavelengths corresponding to blue, green, and red colors.
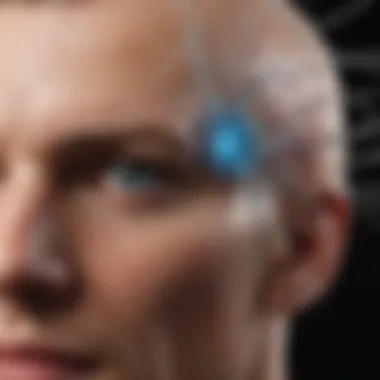
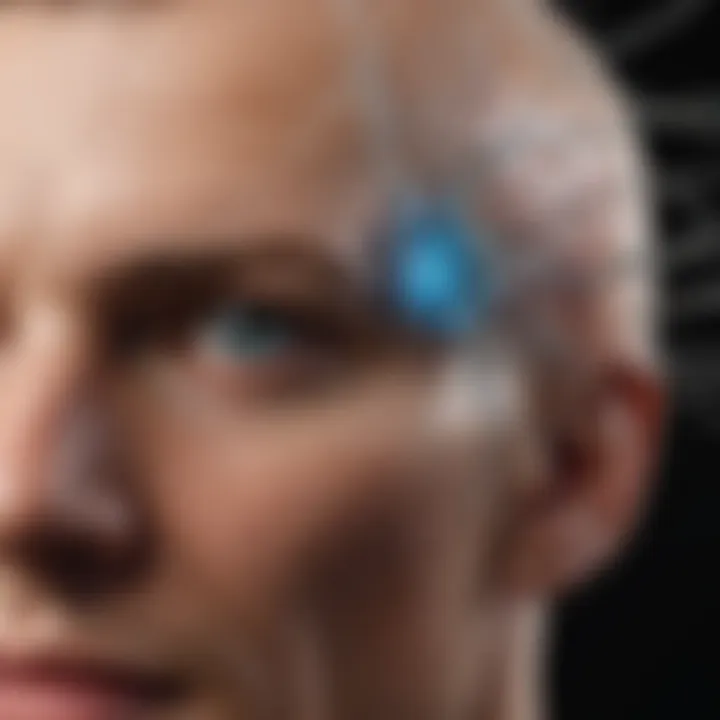
These cells excel in daylight conditions, allowing for detailed central vision. The disadvantage of cones is their lower sensitivity in low light compared to rods. This aspect emphasizes the contrast in functionalities between rods and cones, which together harmonize to provide a full visual experience.
Structure and Function
Understanding the structure and function of eyecells helps in deciphering their operational mechanics in visual perception. Each component of the eyecell plays a role in processing visual signals.
Outer Segment
The outer segment of photoreceptor cells contains disc membranes packed with photopigments. This structure is critical for capturing light photons, initiating the process of visual signal transduction.
A key characteristic of the outer segment is its layered arrangement, maximizing surface area for light absorption. This unique feature is beneficial because it enhances the photoreceptor’s ability to respond to a wide range of light intensities. However, the outer segment is also prone to degeneration, which can impact vision adversely.
Inner Segment
The inner segment is where essential cellular processes occur. It houses organelles like mitochondria that provide energy for phototransduction. The inner segment is vital for maintaining the health and functionality of photoreceptors.
Its significance lies in providing the necessary resources for the phototransduction cascade, translating light into electrical signals. The disadvantage here is that any dysfunction can lead to issues in the conversion process, affecting overall vision.
Synaptic Terminal
The synaptic terminal connects the photoreceptors to bipolar cells, playing a major role in the transmission of visual signals to the brain. It releases neurotransmitters that signal the presence or absence of light to subsequent cells in the visual pathway.
A key characteristic of the synaptic terminal is its ability to modulate signal strength depending on the light intensity. This adaptability is crucial for enhancing visual contrast. However, the synaptic terminal is susceptible to various diseases, complicating visual transmission and processing.
Mechanism of Visual Perception
The mechanism of visual perception is central to understanding how humans and many animals interact with their environment. This section unpacks the intricate processes involved in converting light into a visual experience. By breaking down the mechanisms at play, we can appreciate the delicate balance of biological systems that allows sight. Visual perception encompasses more than just the act of seeing; it includes interpreting and responding to visual stimuli. Hence, understanding this topic helps bridge the gap between biological functions and practical applications in various fields, from medicine to technology.
Light Transduction
Light transduction is the process where photoreceptor cells in the retina convert light into electrical signals. This is an essential step in visual perception because without it, we could not process visual information. The initial interaction occurs when photons, or light particles, strike the photoreceptors, primarily rods and cones.
Within these cells, a series of biochemical reactions are triggered, leading to a change in membrane potential. Rods are highly sensitive to light and primarily enable night vision, while cones function in bright light and enable color perception. Each type of photoreceptor utilizes a different visual pigment. For instance, rods contain rhodopsin, while cones contain opsins. This difference underlies their distinct roles in vision.
Importance of Light Transduction
- Enables contrast sensitivity and adaptation to varying light conditions.
- Facilitates color differentiation through cones.
- Allows for quick responses to visual stimuli, crucial for survival.
- > Without efficient light transduction, visual perception would break down, impairing daily functions and biological interactions.
Role of Retinal
The retinal is a vital component in the visual transduction pathway. This molecule binds with opsins in the photoreceptors, initiating the process of converting light signals into electrical impulses. Retinal undergoes isomerization when it absorbs photons, changing from its 11-cis form to all-trans form. This conformational change starts a cascade of events within the photoreceptor cells that lead to hyperpolarization, ultimately resulting in neurotransmitter release.
The retinal exists in two major forms, both of which play crucial roles in different types of photoreceptors. For example, in the rods, it is fundamental for low-light vision. Conversely, in cones, retinal contributes to color vision, as the different types of cones each have specific opsins that respond to various wavelengths of light.
Significance of Retinal
- Central to the phototransduction cascade, facilitating vision.
- Allows adaptation to changes in light intensity.
- Plays a role in both night and color vision, contributing to the overall visual experience.
Understanding the roles of light transduction and retinal is critical in exploring the broader topic of visual perception. These mechanisms not only underlie how we see but also influence technology and clinical practices aimed at treating vision-related issues.
Eyecell and Neural Pathways
Understanding the relationship between eyecells and neural pathways is crucial for fully grasping the complex nature of visual perception. Eyecells, specifically photoreceptors, initiate the process of vision by converting light into electrical signals. These signals then travel along neural pathways to various parts of the brain. This journey plays a significant role in how we perceive and interpret visual information.
The interactions between eyecells and neural pathways form the groundwork for numerous aspects of our ability to see. It is not merely the presence of light that matters but how that light information is processed in the brain. Here, we will explore two main elements: the transmission of visual signals and the integration of these signals within the brain.
Transmission of Visual Signals
Visual signal transmission begins in the retina, where photoreceptors transform light into electrical impulses. Rods and cones play distinct yet complementary roles in this process.
- Rods are sensitive to low light and enable night vision. They gather dim light and send signals to the bipolar cells, which then relay the information to the ganglion cells.
- Cones, in contrast, function best in bright light conditions and are essential for color vision. They send similar signals through a comparable pathway.
The movement of signals from the rods and cones to the brain happens via the optic nerve. This nerve carries the aggregated signals from approximately one million ganglion cells. As it reaches the brain, the information is not yet fully processed; it is merely a raw data set of visual impulses, requiring further interpretation.
Despite the apparent efficiency of this process, complexities arise. For instance, if the signals are interrupted, as can be seen in various eye disorders, visual perception dramatically declines. It shows the importance of uninterrupted transmission for maintaining the quality of vision.
Integration in the Brain
Once visual signals reach the brain, they must be integrated into meaningful perceptions. The primary area responsible for processing visual information is the visual cortex located in the occipital lobe. Here, the brain decodes what has been transmitted by the eyecells.
- The integration process involves several steps, including depth perception, motion detection, and color differentiation. This allows us to create vivid representations of nuestro entorno, enabling response to it.
Moreover, various regions of the brain communicate extensively during this integration. Signals from visual fields interact not only with visual processing centers but also with areas related to memory and emotions. This interplay facilitates responses based on past experiences and current emotions associated with visual stimuli.
"The visual cortex does not just receive data; it constructs our reality from it, linking raw signals with memories, expectations, and emotions."
The sophisticated nature of visual integration reflects how deeply intelligent our visual system is. Disruptions in this process can occur due to various neurological disorders, emphasizing how vital smooth integration is for functional vision.
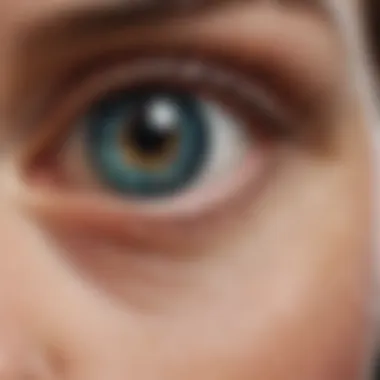
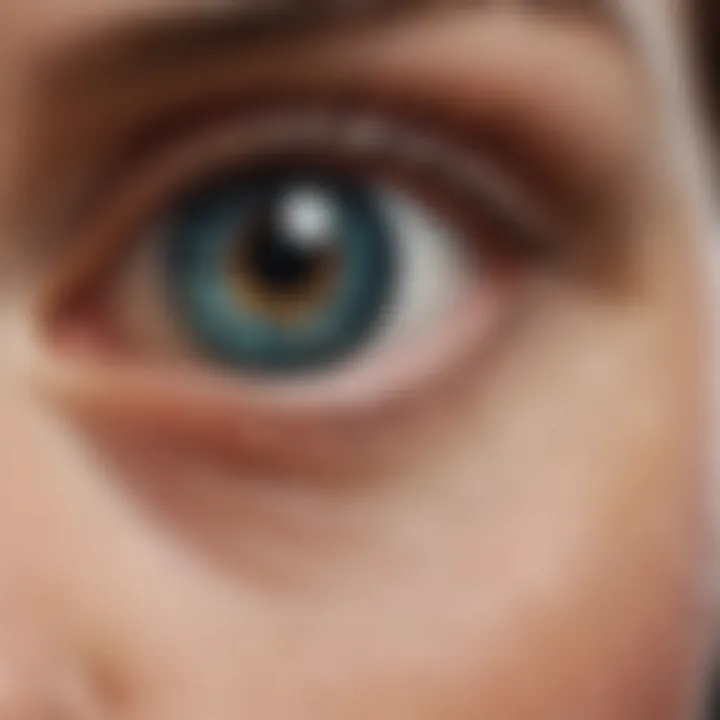
The field of research is moving continuously, examining how neural pathways interact with eyecells at more granular levels. Understanding these mechanisms opens doors for innovations in therapies and technologies that could enhance or restore vision for those with impairments.
Recent Advances in Eyecell Research
Recent advances in eyecell research have significantly impacted our understanding of visual perception. These developments illustrate the intersection of technology and biology, leading to fresh insights and potential applications. As we advance, it becomes crucial to explore innovative techniques that researchers are utilizing to uncover new layers surrounding eyecells. The advancements also highlight some existing considerations, such as ethical implications and practical applications. Overall, these breakthroughs are instrumental for students, researchers, educators, and professionals seeking to expand knowledge in the field.
Innovative Techniques
Optogenetics
Optogenetics is a modern technique that allows precise control of neuronal activity using light. This method plays a crucial role in studying eyecells by enabling researchers to manipulate photoreceptor response actively. One key characteristic is its ability to selectively target specific cell types, making it a beneficial tool in understanding the functionality of various eyecells in the visual pathway.
The unique feature of optogenetics is the incorporation of light-sensitive proteins, which can be expressed in targeted neuronal populations. This allows researchers to turn cells on or off with light pulses, providing insight into their roles in perception.
While optogenetics offers several advantages, such as high spatial and temporal resolution, it is not without drawbacks. The requirement for genetic modification may pose challenges in certain applications. Additionally, not all targeted cells respond uniformly to light, which can complicate the interpretation of results.
Imaging Technologies
Imaging technologies, particularly advancements in microscopy, are another critical area in eyecell research. Techniques like two-photon microscopy provide detailed views of the microstructure within the eye, enabling deeper insights into how eyecells interact and function. These technologies contribute to visualizing the anatomy and physiology of eyecells in real time, which is essential for understanding visual perception.
One prominent characteristic of imaging technologies is their ability to capture dynamic processes at cellular resolutions. This characteristic makes these tools a popular choice for researchers studying eyecells and related pathways.
The unique advantage of imaging technologies is their non-invasive nature. Researchers can obtain high-resolution images without disrupting the biological processes they study. However, limitations exist, such as the technical complexity and the need for specialized equipment.
Recent Findings
Recent findings in eyecell research have been groundbreaking. Studies have shown how specific eyecell structures adapt in response to light exposure and how disruption at the cellular level can lead to visual impairments. Understanding these complex interactions provides potential pathways for developing treatments for various vision disorders.
Clinical Implications of Eyecell Studies
Understanding eyecells and their functions has significant medical relevance. Research in this field is influencing how vision disorders are treated. Advances provide insights that can ameliorate conditions like retinitis pigmentosa and age-related macular degeneration. These insights can guide the development of new therapies and rehabilitation strategies.
This section explores two critical areas: vision disorders and their treatments, alongside future prospects in gene therapy. Both of these aspects highlight the transformative potential of eyecell research in clinical practice.
Vision Disorders and Treatments
Vision disorders affect millions of people globally. Conditions stemming from eyecell dysfunction are often challenging to treat. However, increased understanding of how photoreceptors operate offers new paths for mitigating these issues.
For instance, retinitis pigmentosa is a genetic disorder that leads to progressive vision loss. Current treatments focus on managing symptoms, but ongoing research aims to restore function to damaged eyecells. Some therapies are now being tailored to target specific mutations that underlie this condition.
In addition, treatments for diabetic retinopathy are evolving. New clinical trials investigate medications that can prevent or slow the progression of this disease. Learning how eyecells degenerate in response to hyperglycemia helps researchers identify effective intervention strategies.
"The integration of eyecell biology in treatment protocols marks a substantial shift towards precision medicine."
Moreover, regenerative medicine is another exciting area influenced by eyecell research. Stem cell therapies are being explored to replace damaged photoreceptors, offering hope for restoring vision in individuals with severe retinal conditions.
Gene Therapy Prospects
Gene therapy stands at the forefront of eyecell-related interventions. This approach focuses on directly altering the genes responsible for vision disorders. Progress in identifying the genes linked to specific eye diseases has laid the groundwork for effective gene therapies.
One notable therapy is Luxturna, approved for treating specific forms of retinitis pigmentosa. This therapy successfully delivers a normal copy of the RPE65 gene into the retinal cells. Clinical trials reveal promise for further gene-based treatments, potentially for a broader range of vision disorders.
The application of CRISPR technology in modifying genes offers additional prospects for future therapies. Scientists aim to correct genetic defects that lead to eye diseases at the molecular level. Research continues to explore how to safely apply these techniques in clinical settings, focusing on long-term outcomes for patients.
In summary, the clinical implications of eyecell studies are profound. Vision disorders can profoundly impact quality of life, yet advances in understanding these mechanisms present promising avenues for treatment. Studying eyecells opens new doors for innovative therapies that can change lives.
Technological Applications Arising from Eyecell Research
The study of eyecells has opened up a range of technological applications that hold promise for various fields, especially in medicine and visual technology. Understanding how these cells function can lead to significant benefits, including improvements in eye-related treatments and enhancements in visual devices. The advancements that arise from eyecell research can potentially revolutionize how we approach visual disorders and create new pathways for artificial vision.
Advancements in Visual Technology
In recent years, the exploration of eyecells has led to remarkable advancements in visual technology. One key area of focus has been the development of imaging techniques. Techniques such as adaptive optics allow for real-time imaging of the retina with extraordinary precision. This technology is crucial for diagnosing ailments like macular degeneration and diabetic retinopathy, enabling interventions before permanent damage occurs.
Moreover, there are innovative augmented reality solutions that rely on the understanding of photoreceptors. By mimicking the natural processing of visual information done by eyecells, augmented reality devices can provide more accurate and immersive experiences. This enhances training programs in various fields such as medicine and engineering, allowing for practical applications of complex concepts.
Artificial Vision Systems
Artificial vision systems are another significant byproduct of eyecell research. These systems aim to compensate for the loss of sight by directly interfacing with the neural pathways activated by eyecells. One of the most notable advancements is the development of retinal implants. These devices convert camera images into electrical impulses, which stimulate surviving retinal neurons, thus restoring some vision to individuals with severe vision loss.
Additionally, researchers are exploring the use of bio-inspired algorithms, which aim to replicate how eyecells process visual information. This could lead to the creation of more sophisticated artificial vision systems that offer better image recognition and processing capabilities.
"As we push the boundaries of eyecell research, the potential for developing next-generation visual technologies seems limitless."
In summary, the technological applications arising from eyecell research are not merely theoretical. They have practical implications that touch on both direct medical applications and enhancement of visual technologies. As research continues, we can expect further breakthroughs that will benefit individuals with vision impairments while also advancing general visual technology.
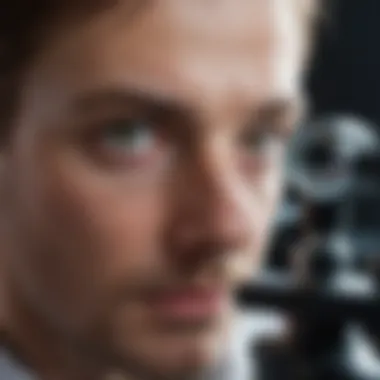
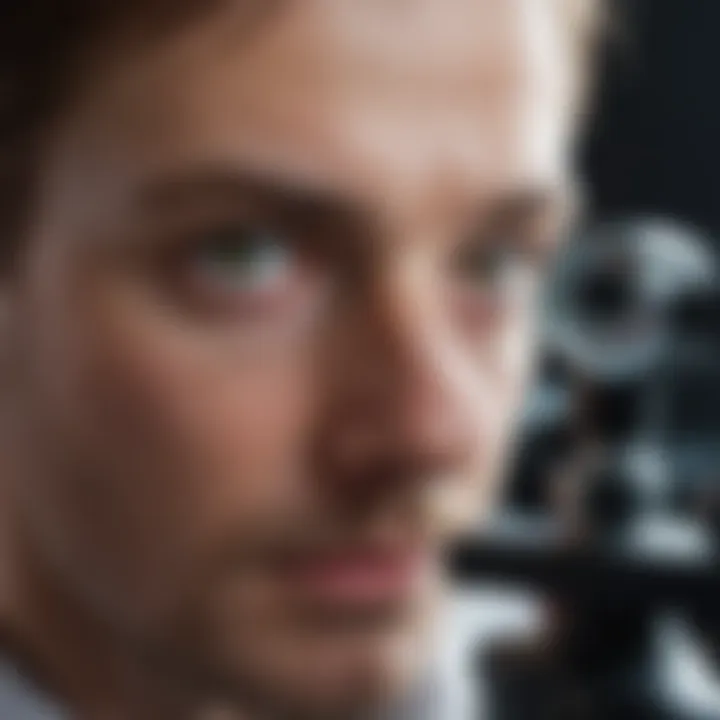
Eyecells in Evolutionary Biology
The study of eyecells within the context of evolutionary biology offers significant insights into how visual systems have adapted over time. This understanding can elucidate the mechanisms behind species survival, development of complex visual tasks, and the evolutionary pressures that have shaped the diversity of visual perception among different organisms. Exploring eyecells through this lens reveals more than just their anatomical structures; it engages with the adaptive functions that these cells serve in a broad range of environments. This section will address comparative anatomy and the role of eyecells in adaptation and survival, forming a comprehensive picture of their evolutionary trajectory.
Comparative Anatomy
Comparative anatomy focuses on the similarities and differences in the structure of eyecells across various species. Analyzing these variations helps in understanding how different organisms have evolved their visual systems to meet ecological demands. For example, the eyecells of nocturnal animals like owls display adaptations that optimize for low light conditions, showing increased numbers of rods. On the other hand, creatures like mantis shrimp possess unique photoreceptors, allowing them to detect an extensive range of wavelengths, far beyond human capability.
Key points from comparative anatomy include:
- Diversity in Structure: Differences in rods and cones have evolved based on environmental needs.
- Evolutionary Pressure: Natural selection has fine-tuned these cells for specific tasks, such as detecting predators and foraging for food.
- Developmental Pathways: Understanding genetic pathways that lead to the different structures of eyecells can also provide insight into evolutionary changes over time.
Through this comparative study, researchers can further grasp the adaptations that enhance the fitness of various species. Insights from eyecell anatomy can also inform biologists regarding how visual capabilities might change in response to environmental shifts.
Adaptation and Survival
The adaptation of eyecells is central to survival in changing environments. This adaptation permits species to improve their chances of survival and reproduction under specific ecological conditions. For instance, cichlid fish in African lakes have evolved specialized photoreceptors to better navigate murky waters, where light conditions drastically differ.
Important considerations in this area include:
- Selection Pressures: Environmental factors such as light availability influence the evolution of eyecells.
- Behavioral Correlations: Adaptations in eyecells often correlate with behavioral changes, such as improved hunting techniques or mate selection.
- Impact on Ecosystems: Changes in visual perception can affect entire ecosystems, influencing predator-prey dynamics and inter-species interactions.
Understanding how eyecells adapt across species informs not only our view of evolution but also potential applications in technology and medicine.
Ethical Considerations in Eyecell Research
Ethical considerations are crucial in the field of eyecell research. As the understanding of eyecells progresses, so too does the responsibility to ensure that research is conducted in a manner that respects human dignity and promotes welfare. The implications of research expand not only in terms of scientific advancement but also in its effect on society at large. This section discusses the significance of these ethical considerations and their emerging relevance in ongoing studies involving eyecells.
Research Ethics
The ethical foundation of research into eyecells involves strict adherence to guidelines that safeguard the interests of participants. Researchers must consider the implications of experimental procedures, especially when they involve human subjects or the potential use of genetic manipulation. Central topics include informed consent, the welfare of human and animal subjects, and the potential for unintended consequences.
- Informed Consent: Participants must be fully aware of their involvement, its risks, and its benefits. Transparency fosters trust between researchers and subjects.
- Welfare of Subjects: Researchers must prioritize the physical and psychological safety of their subjects at all times. This consideration extends to the use of animal models in eyecell studies.
- Potential for Misuse: Advances in gene therapy and technology related to eyecells might lead to misuse or genetic discrimination. Ethical research should aim to minimize such risks.
These points highlight the multi-faceted ethical landscape in which eyecell research operates, emphasizing the need for careful planning and oversight.
Societal Impact
The societal impacts of eyecell research are profound and wide-reaching. As advancements in this field proliferate, they pose significant questions regarding accessibility, equity, and informed public discourse.
- Accessibility of Treatments: As new treatments based on eyecell research emerge, ensuring equitable access becomes imperative. There is a potential for disparities in how different socio-economic groups benefit from these advancements.
- Public Understanding and Engagement: Society must engage with the scientific community to understand the implications of eyecell research fully. Misinformation can hinder progress and lead to public skepticism.
- Ethical Conversations on Enhancement: With capabilities for enhancing vision on the horizon, ethical discussions must consider how these technologies are applied and who has access. There are fears that they might lead to a divide between those who can afford enhancements and those who cannot.
"The intersection between ethics and eyecell research will shape future innovations. Addressing concerns now can lead to better societal outcomes later.”
In summary, addressing ethical considerations in eyecell research is imperative not just for compliance with regulations, but for fostering a responsible framework that acknowledges potential societal impacts. Researchers must engage in discussions that bridge science and ethics, ultimately benefitting the collective populace.
Future Directions in Eyecell Research
Research surrounding eyecells is at a pivotal point, with potential to significantly impact diverse fields such as medicine, technology, and even psychology. The future directions in eyecell research hold the promise of unraveling complex mechanisms of visual perception. Understanding these advancements can lead to better therapeutic options for vision-related disorders and enhance the development of artificial vision systems. This section outlines investigative approaches that can be pursued and highlights potential breakthroughs in how we perceive and interface with the world around us.
Investigative Approaches
Future eyecell research demands innovative investigative approaches. Initiatives are increasingly focused on interdisciplinary methods that combine biology, technology, and computational models. Key approaches include:
- Genetic Manipulation: Techniques like CRISPR-Cas9 offer the ability to edit genes in eyecells, possibly correcting hereditary vision disorders. This genetic editing can help in understanding gene functions within photoreceptors, providing insights into how defects lead to vision loss.
- 3D Imaging: Novel imaging technologies like two-photon and super-resolution microscopy allow researchers to visualize inner workings of eyecells with greater clarity. These techniques provide high-resolution images that help elucidate structural details and dynamic processes.
- Clinical Studies: Observational and interventional clinical trials can provide regarding the efficiency of new therapies and technologies on human subjects. These studies must be rigorously designed to ensure that the findings are meaningful and applicable to a wider population.
These approaches not only boost the quality of research but also help in applying findings to real-world challenges in visual perception.
Potential Breakthroughs
As the field evolves, several potential breakthroughs could redefine our understanding of vision and eyecell functions. Some of the most promising areas include:
- Restoration of Vision: Advances in gene therapy could lead to significant breakthroughs in restoring sight to individuals suffering from inherited retinal diseases. Researchers are optimistic about the safety and efficacy of gene-based treatments in eyecells to reverse conditions like retinitis pigmentosa.
- Enhanced Artificial Vision: Current artificial vision systems can be significantly improved by incorporating insights gained from eyecell functionality. Developing bio-inspired technologies might result in sophisticated visual prosthetics that interact seamlessly with human neural pathways.
- Neuroprotection Mechanisms: Identifying neuroprotective strategies could mitigate cell death in the retina, preserving the eyesight of aging populations. Research into compounds that enhance the resilience of eyecells against degenerative processes may lead to transformative treatments.
"The integration of advanced technologies and biological insights can unlock new pathways towards therapies that were solely theoretical a few years ago."
Such breakthroughs are not merely theoretical; they have the potential to impact millions affected by visual impairments and advance our understanding of human perception itself.
In summary, future directions in eyecell research open up a multitude of pathways for scientific inquiry. The combination of innovative investigative approaches and the possibility of breakthrough treatments will surely define the next era of visual science.
Culmination
The conclusion of this article brings together the various aspects discussed regarding eyecell and its significant role in visual perception. This section is essential as it distills the comprehensive information and research findings into a digestible form, allowing readers to reflect on the content and understand its implications. The insights gained from this article not only highlight the biological intricacies of photoreceptors but also emphasize the innovative approaches and technologies that stem from ongoing research. Considerations about the subject suggest that the understanding of eyecells is crucial for both scientific advancement and practical applications.
Recapitulation of Key Points
In summarizing the key elements covered in the article, a few critical points emerge:
- Understanding of Eyecells: Eyecells are fundamental for visual perception. The composition and function of photoreceptor cells show how the human eye processes visual stimuli.
- Recent Advances: Innovations in techniques such as optogenetics and imaging technologies have significantly improved research capabilities, shedding light on previously unclear aspects of visual processing.
- Clinical Relevance: The implications of eyecell research extend into the treatment of vision disorders, suggesting a transformative potential in medical practices through gene therapy and advanced visual technologies.
- Ethical Implications: Addressing the ethical considerations is essential in guiding future research. Society must reflect on the impact such advancements may have on human experience and health care.
Final Thoughts
In closing, the exploration of eyecells presents a fascinating intersection of biology, technology, and medicine. As our understanding deepens, the potential for breakthroughs increases exponentially. It is imperative for students, researchers, and professionals to remain engaged with this topic. Continued exploration may yield new discoveries, changing the future of visual technologies and treatments for vision disorders. By investing in eyecell research, we invest in a more profound understanding of human perception and the enhancement of life quality through innovative solutions. As advancements continue, a careful consideration of societal impacts will ensure that progress aligns with ethical standards, benefitting all of humanity.