The Role of Asynuclein in Neurodegenerative Disorders
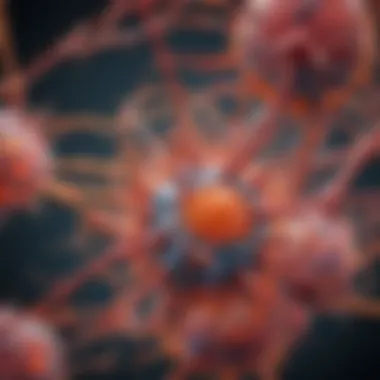
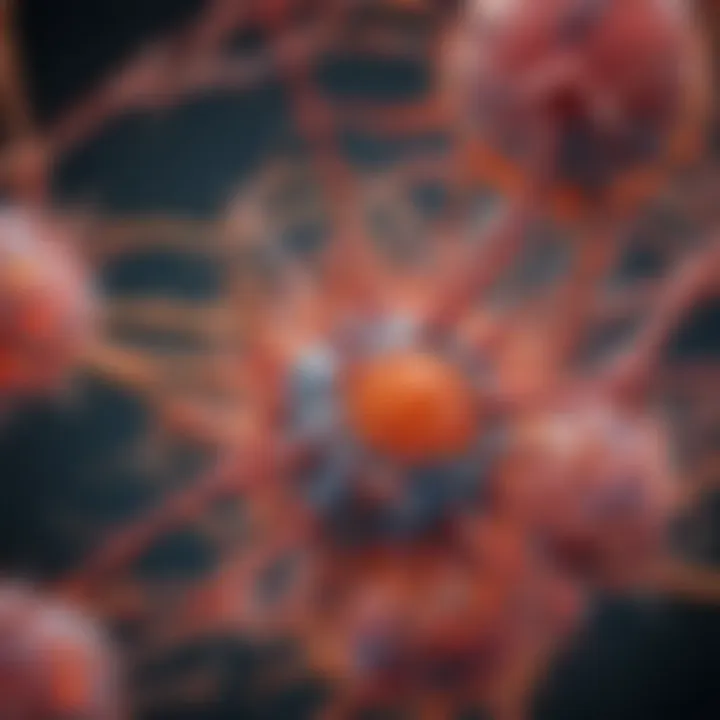
Intro
Asynuclein is a small protein but plays a significant role in neurodegenerative diseases, especially Parkinson's disease. This protein has become a focal point of research because of its association with various pathological processes. Understanding asynuclein's role can give insight into the mechanisms of neurodegeneration and pave the way for potential therapeutic interventions. This article aims to explore the structure, function, and implications of asynuclein in depth, while thoroughly examining current trends in research.
Research Highlights
Key Findings
- Structure and Form
Asynuclein exists in several forms, including monomers, oligomers, and fibrils. The misfolding of this protein leads to the formation of toxic aggregates, which are a hallmark of neurodegenerative diseases. - Function of Asynuclein
Normally, asynuclein is thought to play a role in synaptic function and neurotransmitter release. However, when it misfolds, it disrupts normal neuronal operations. - Association with Neurodegenerative Diseases
Abnormal accumulations of asynuclein are observed in conditions like Alzheimer's disease, Lewy body dementia, and multiple system atrophy, in addition to Parkinson's disease. - Research Trends
Recent studies emphasize identifying biomarkers related to asynuclein that could aid in the early diagnosis of neurodegenerative diseases. Also, therapeutic strategies targeting asynuclein aggregation show promise in early trials.
Implications and Applications
- Diagnostic Potential
Developing tests to detect asynuclein aggregates could lead to earlier interventions. Biomarkers related to this protein might reveal the onset of neurodegenerative diseases at a more treatable stage. - Therapeutic Strategies
There are ongoing clinical trials exploring the inhibition of asynuclein aggregation. Strategies like vaccination against asynuclein or using small molecules to prevent its misfolding could significantly alter treatment approaches.
"Understanding asynuclein's pathology is crucial for developing effective therapies and diagnostics in neurodegenerative diseases."
Methodology Overview
Research Design
The approach to studying asynuclein typically involves a blend of in vitro and in vivo methods. Researchers often utilize animal models to mimic disease conditions, offering insights into asynuclein's role in vivo.
Experimental Procedures
Experiments may include:
- Biophysical techniques to analyze asynuclein structure. Techniques like X-ray crystallography and NMR spectroscopy help visualize the protein under different conditions.
- Cellular studies to observe the effects of asynuclein aggregation on neuronal health. These studies often utilize culture models, where the behavior of neurons can be monitored.
- Genetic approaches involving transgenic models which express mutant forms of asynuclein. These models are vital for understanding the mechanisms of toxicity associated with this protein.
In summary, exploring asynuclein's role in neurodegenerative diseases involves a multifaceted approach. Research continues to reveal the complexities surrounding this protein, presenting opportunities for diagnostic and therapeutic advancements. As ongoing studies unfold, further understanding of asynuclein can enhance treatment strategies and improve patient outcomes in neurodegenerative disorders.
Preface to Asynuclein
Asynuclein is a key protein in the study of neurodegenerative diseases. Understanding its role within the brain allows researchers to explore potential pathways for treatment and intervention. Asynuclein's significance is particularly evident in conditions like Parkinson's disease, where it contributes to pathological changes. Specifying how this protein functions and interacts with cellular mechanisms is essential for anyone interested in neuroscience.
Definition and Context
Asynuclein is a small, soluble protein predominantly found in the presynaptic terminals of neurons. It is coded by the SNCA gene, and its function has been associated with synaptic vesicle regulation and neurotransmitter release. The protein can exist in several forms, including monomers, oligomers, and fibrils. In a healthy state, asynuclein seems to play a role in synaptic function; however, its aggregation leads to neurotoxicity.
The pathological aggregation of asynuclein is a hallmark of several neurodegenerative disorders. Parkinson’s disease is the most studied context where this aggregation manifests in the formation of Lewy bodies, which are intracellular inclusions mainly composed of misfolded asynuclein. Beyond Parkinson's, abnormal asynuclein accumulation is observed in other diseases such as Lewy Body Dementia and Multiple System Atrophy, which also highlight the importance of understanding this protein's behavior and implications in neurodegeneration.
Historical Background
The discovery of asynuclein dates back to the late 1990s. Researchers first identified it as a component of Lewy bodies in patients with Parkinson’s disease. Interest in asynuclein surged after evidence suggested its role in the pathogenesis of various neurodegenerative conditions. Early studies revealed that mutations and gene multiplications of the SNCA gene could lead to familial forms of Parkinson’s disease.
Subsequent research has expanded the understanding of asynuclein’s functions and implications. In 2004, a landmark discovery published in Nature outlined the structure of asynuclein, shedding light on its propensity to aggregate. This research opened a pathway for exploring therapeutic targets focused on inhibiting asynuclein aggregation. The integration of genetic, biochemical, and neuroimaging techniques continues to refine the profile of asynuclein in modern neuroscience.
Asynuclein Structure
Understanding the structure of asynuclein is crucial to comprehend its role in neurodegenerative diseases. Asynuclein, predominantly known for its aggregation in conditions such as Parkinson's disease, has a unique structure that influences its function and behavior in neural environments.
Amino Acid Composition
The amino acid composition of asynuclein is vital for its biological activity. This protein comprises 140 amino acids and is classified as an intrinsically disordered protein. The presence of specific amino acids, such as valine, serine, and glycine, plays a role in the protein's ability to adopt various conformations. Notably, the high density of proline residues at positions 11 and 12 can impact the folding and stability of the protein.
An interesting aspect of the amino acid profile is the hydrophobic regions that facilitate interaction with lipid membranes. Such interactions are essential for synaptic function and may highlight potential mechanisms for its involvement in neurodegeneration. By understanding the amino acid make-up, researchers can better anticipate how mutations or alterations might lead to pathological aggregates.
Protein Folding and Confirmation
Protein folding is another critical element in the study of asynuclein. Initially, asynuclein adopts a random coil conformation in solution. However, upon interacting with lipid membranes or cellular components, it may transition to an alpha-helical structure. This structural versatility permits asynuclein to fulfill multiple roles in neuronal function, including neurotransmitter release and synaptic regulation.
When asynuclein misfolds, it can form oligomers and fibrils. These aggregates are characteristic of several neurodegenerative diseases, leading to cytotoxic effects. Understanding the folding pathways helps in identifying potential interventions that can prevent misfolding. It also raises questions on how certain environmental or genetic factors may predispose individuals to these pathological changes.
Role of Post-Translational Modifications
Post-translational modifications (PTMs) of asynuclein significantly influence its physiological and pathological properties. These modifications include phosphorylation, ubiquitination, and nitration, among others. Each modification has the potential to alter the stability, aggregation propensity, and function of the protein.
For instance, phosphorylation at specific serine residues can promote or inhibit aggregation, while ubiquitination may target asynuclein for degradation. Such modifications provide insights into the protein's dynamic regulation, offering potential biomarkers for disease progression. Understanding PTMs helps in comprehending the complex network of cellular signaling pathways that interact with asynuclein, further underlining its importance in neurodegenerative disorders.
"The structure of asynuclein is not just a detail; it is integral to understanding its role in health and disease."
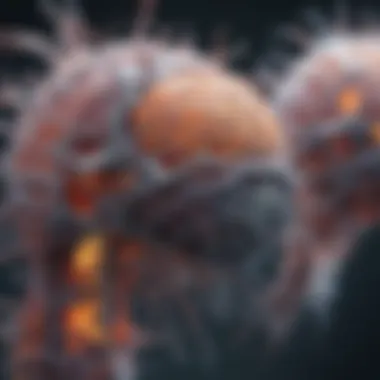
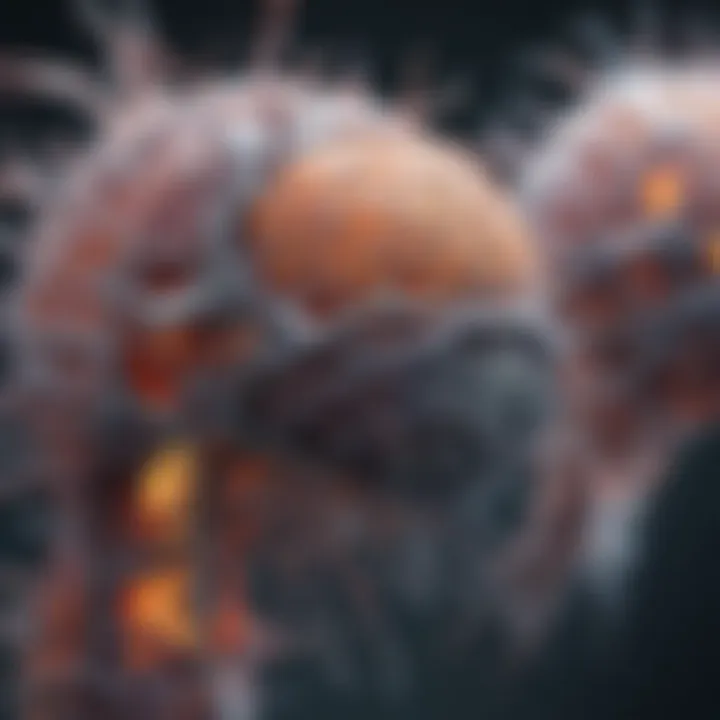
Overall, the structural characteristics of asynuclein are foundational. They provide a platform upon which its involvement in neurodegeneration is built. With ongoing research, the hope is to decipher the complexities surrounding this remarkable protein.
Function of Asynuclein
Asynuclein serves a crucial role in various neuronal processes, and understanding its functions is essential for grasping its implications in neurodegenerative diseases. This section focuses on how asynuclein contributes to synaptic function, neurotransmitter release, and neuronal survival. Each aspect interlinks, showcasing how disruptions in asynuclein's normal functioning may lead to neuronal degeneration and related disorders.
Involvement in Synaptic Function
Asynuclein is integral to the maintenance of synaptic structures. It mostly resides in presynaptic terminals, where it interacts with synaptic vesicles. The protein's presence facilitates the formation and maintenance of these key cellular structures.
When synaptic function is compromised, it can affect communication between neurons. Asynuclein influences the recycling of synaptic vesicles, which are essential for neurotransmitter release. A proper balance of asynuclein in the synapse enables efficient neurotransmission. Disruption or aggregation of asynuclein can lead to synaptic dysfunction, ultimately contributing to neurodegeneration.
Role in Neurotransmitter Release
The process of neurotransmitter release is a finely tuned mechanism requiring the coordination of multiple proteins. Asynuclein plays a vital role in this. It modulates the interaction between synaptic vesicles and the presynaptic membrane. Through its action, asynuclein can influence the timing and the quantity of neurotransmitter release, ensuring timely communication between neurons.
Excessive asynuclein aggregation can hinder this release. Studies have shown that increased asynuclein levels can lead to impaired vesicle trafficking and defective neurotransmission. This dysfunction can be seen prominently in Parkinson's disease, where abnormal neurotransmitter levels significantly affect movement and cognition.
Influence on Neuronal Survival
Neuronal survival is tightly linked to the health of synaptic functions, and asynuclein has a significant impact here as well. It appears to protect neurons from stress and promote overall cellular health. Typically, asynuclein supports crucial pathways related to neuronal survival, including mitochondrial function and cellular responses to stress.
When asynuclein misfolds or aggregates, it loses its protective capability. This change can trigger various pathways leading to cell death. Both loss of function and toxic gain of function are associated with pathological conditions. Protecting neuronal survival in the presence of asynuclein anomalies is essential for preventing neurodegenerative diseases.
"Understanding how asynuclein regulates synaptic function, neurotransmitter release, and neuronal survival is critical for developing targeted therapies."
In summary, the function of asynuclein in these processes highlights its dual nature as both a facilitator and a potential hazard for neurons. Disruption in its normal functioning can pave the way for neurodegenerative diseases, necessitating further research into its roles and interactions.
Asynuclein and Neurodegenerative Diseases
Asynuclein's role in neurodegenerative diseases is critical and multifaceted. Understanding this relationship helps to clarify the underlying processes that lead to conditions like Parkinson's disease, Lewy body dementia, and multiple system atrophy. The protein asynuclein accumulates abnormally in these diseases, indicating it may be a key player in their development and progression. Studying asynuclein is not only vital for unlocking the mechanisms of these diseases but also for improving diagnostic and therapeutic approaches.
The significance of asynuclein in the brain's cellular functions is well documented. Its aggregation in neurons leads to significant cellular dysfunction. The pathologies associated with asynuclein can reflect the broader implications in the field of neurobiology and even the potential for targeted interventions.
One important element in understanding asynuclein is its influence on neuroinflammation, which is increasing seen as a contributor to neurodegenerative diseases.
This understanding helps to highlight several key aspects:
- Pathology Understanding: Understanding how asynuclein aggregates informs researchers about disease mechanisms.
- Biomarkers: Asynuclein levels can serve as potential biomarkers for early diagnosis, aiding in prompt interventions.
- Therapeutic Targeting: Knowing how asynuclein dysfunction contributes to disease may lead to novel therapeutic strategies.
Parkinson's Disease: A Focus
Parkinson's disease (PD) notably showcases the role of asynuclein. It is characterized by the presence of Lewy bodies formed from aggregated asynuclein. The pathology of PD reveals how asynuclein influences dopaminergic neuron loss. Patients with Parkinson's often present motor symptoms such as tremor, rigidity, and bradykinesia, which correlate with the extent of neuron loss associated with asynuclein aggregation. Research has shown that reducing asynuclein levels can alleviate some symptoms in animal models, emphasizing the potential for targeted therapies that inhibit asynuclein aggregation.
Lewy Body Dementia
Lewy body dementia (LBD), another condition linked to asynuclein, provides insight into both cognitive and motor impairments. LBD is significant because it presents unique clinical features such as visual hallucinations alongside the motor symptoms similar to Parkinson's. The presence of asynuclein aggregates in the brain of LBD patients parallels findings in Parkinson's disease, reinforcing the concept that asynuclein dysregulation is a common pathological theme in synucleinopathies. Furthermore, targeting asynuclein may improve both cognitive and motor symptoms, positioning this as a critical area for future interventions.
Multiple System Atrophy
Multiple system atrophy (MSA) also aligns with asynuclein-related pathology. MSA involves the progressive degeneration of multiple systems within the brain and has distinct clinical manifestations such as autonomic dysfunction, ataxia, and parkinsonism. The aggregation of asynuclein in MSA differs from that in Parkinson's and LBD, presenting itself in glial cells. This difference highlights diverse pathogenic mechanisms while still implicating asynuclein as a fundamental component. Understanding the nuances of asynuclein's role across these diseases can lead to tailored treatments, taking into account the specific pathways involved in each neurodegenerative disorder.
Examining asynuclein's involvement in these three major neurodegenerative diseases underlines its importance and the necessity for further research into its pathological mechanisms. Uncovering the specifics may not only help us understand these diseases better but also open doors to innovative therapeutic avenues.
Pathological Mechanisms of Asynuclein
Understanding the pathological mechanisms of asynuclein is crucial for grasping its role in neurodegenerative diseases. This knowledge is essential in identifying targets for therapy and developing diagnostic methods. Asynuclein’s involvement in diseases such as Parkinson's disease, Lewy body dementia, and others hinges on its propensity for misfolding and aggregation. Each of these processes contributes differently to neuronal dysfunction and ultimately cell death.
Aggregation and Toxicity
Asynuclein aggregation forms insoluble fibrils, which are characteristic features of various neurodegenerative disorders. The aggregation process starts with the misfolding of the monomeric form of asynuclein. This misfolded protein can then aggregate into oligomers and fibrils. These aggregates are toxic to neurons.
The toxicity arises through various mechanisms. Oligomeric forms of asynuclein interfere with cellular functions, including mitochondrial activity, leading to increased oxidative stress. Mitochondria are critical for energy production in cells, and their dysfunction can drive the process of neurodegeneration. Thus, the aggregation of asynuclein poses a significant threat to neuronal health and integrity.
"The misfolding and subsequent aggregation of asynuclein are among the most critical steps in the pathogenesis of neurodegenerative diseases."
Impaired Protein Degradation Systems
The presence of aggregated asynuclein disrupts normal protein homeostasis within the neuron. Cells possess mechanisms like the proteasome and autophagy to degrade misfolded proteins. However, asynuclein aggregates can overwhelm these systems, leading to further accumulation of cellular waste and toxic proteins.
Impairment of these degradation pathways can result in a feedback loop. As the aggregates accumulate, they inhibit the proteasome function and autophagic processes, exacerbating the situation. This impaired degradation not only increases the levels of toxic aggregates but also contributes to overall cellular stress.
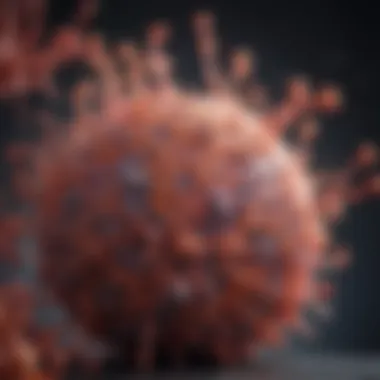
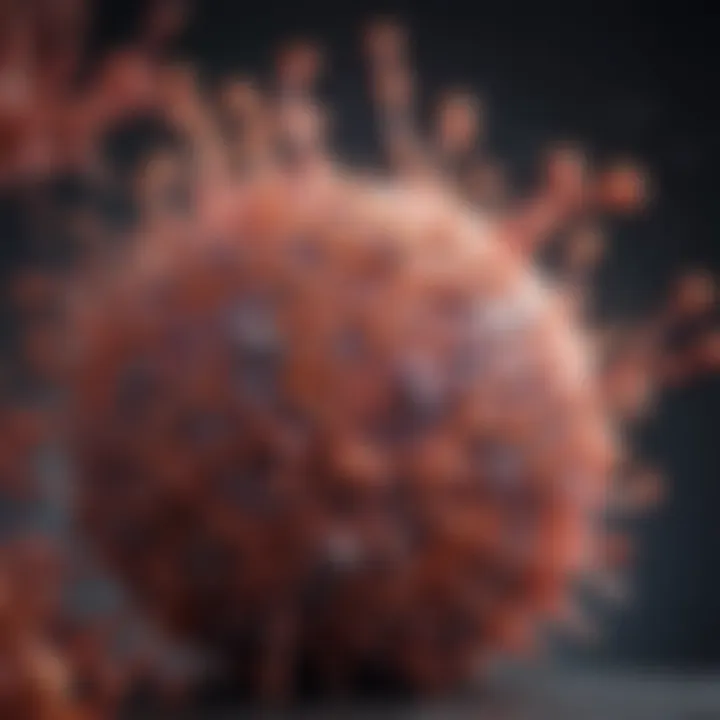
Mitochondrial Dysfunction
As mentioned, mitochondrial dysfunction is a significant aspect of asynuclein pathology. Aggregated asynuclein can localize to mitochondria, disrupting their function. This interaction can lead to decreased ATP production, increased generation of reactive oxygen species, and release of pro-apoptotic factors. The consequence is not limited to energy depletion; it can also trigger cell death pathways.
Moreover, studies show that mitochondrial health is essential for maintaining neuronal function. Thus, understanding how asynuclein impairs these organelles provides insights into potential therapeutic avenues. By addressing mitochondrial dysfunction, researchers aim to mitigate some of the detrimental effects caused by asynuclein pathology.
Current Research on Asynuclein
Research on asynuclein is crucial for understanding its varied roles in neurodegenerative diseases. Continuous studies provide insights not only into the fundamental biology of asynuclein but also into its implications in diagnosing and treating conditions like Parkinson's disease, Lewy Body Dementia, and others. As new findings emerge, they contribute to the development of potential therapeutic strategies and diagnostic tools that could help manage these serious ailments more effectively.
Novel Imaging Techniques
Advancements in imaging techniques have revolutionized the study of asynuclein. Techniques such as positron emission tomography (PET) enable researchers to visualize the accumulation of asynuclein in live subjects. With the help of specific radioligands, researchers can quantify the density of neurodegenerative markers, allowing for earlier diagnosis and better patient monitoring. These imaging methods facilitate the understanding of how asynuclein pathology progresses in the brain.
Genetic Studies and Asynuclein Variants
The exploration of genetic factors influencing asynuclein function has gained momentum in recent years. Genetic studies identify variants of the SNCA gene, which encodes asynuclein. These variants can affect protein aggregation and influence an individual’s susceptibility to neurodegenerative diseases. Understanding how different genetic backgrounds contribute to asynucleinopathies can pave the way for personalized medicine approaches, where treatment is tailored to the specific genetic abnormalities present in a patient.
Animal Models of Asynucleinopathies
Animal models are vital for studying the pathology of asynuclein. By using transgenic mice that overexpress asynuclein, researchers can observe the effects of this protein in real time. Such models help in understanding the progression of diseases and in testing potential therapies. Researchers are continuously refining these models to mimic human diseases more closely, thus providing a better platform for evaluating treatment strategies.
Further studies are needed to ensure that findings in animal models translate effectively into human cases. The gap between preclinical and clinical research is a significant consideration.
In summary, current research on asynuclein encompasses various innovative techniques and approaches. These range from novel imaging strategies to genetic explorations and animal models, all of which contribute to a deeper understanding of this complex protein's implications in neurodegenerative diseases.
Diagnostic Implications of Asynuclein
The study of asynuclein's role in neurodegenerative diseases such as Parkinson's disease places a significant emphasis on its diagnostic implications. Understanding the various aspects of asynuclein detection can lead to earlier interventions and tailored therapeutic strategies. Early diagnosis improves the management of these diseases, potentially enhancing the quality of life for patients.
Potential Biomarkers for Early Diagnosis
Asynuclein has emerged as a promising biomarker for the early diagnosis of neurodegenerative diseases. In conditions like Parkinson’s disease, the presence of misfolded asynuclein can provide valuable insights.
- Asynuclein can be detected in bodily fluids, including cerebrospinal fluid and blood, making it accessible for diagnostic purposes.
- The detection methods for asynuclein are continually improving, which enhances sensitivity.
- Different isoforms of asynuclein offer unique diagnostic potentials, depending on their aggregation state.
The ability to identify asynuclein aggregates as biomarkers could revolutionize early diagnosis strategies, making treatments more effective before significant neuronal damage occurs.
Cerebrospinal Fluid Analysis
Cerebrospinal fluid (CSF) analysis is a critical component in diagnosing neurodegenerative diseases. In this context, asynuclein levels in the CSF can provide important clinical information.
- CSF samples show higher concentrations of asynuclein in patients with Parkinson’s disease compared to healthy individuals.
- Studies indicate that measuring the ratio of phosphorylated to total asynuclein offers insight into disease progression and severity.
- CSF analysis can also help distinguish between different types of neurodegenerative diseases.
Though CSF analysis has robust potential, it is important to note challenges such as the invasiveness of the procedure and variability in sample collection methods.
Blood-Based Biomarkers
Recent advancements have focused on blood-based biomarkers, presenting promising new directions for asynuclein research.
- Blood tests for asynuclein may become a practical and less invasive alternative to CSF analysis.
- Researchers are exploring specific assays that can detect asynuclein aggregates in plasma effectively.
- Successful identification of asynuclein in blood can lead to practical screening methods for high-risk populations.
Despite the exciting possibilities, existing studies show mixed results regarding the sensitivity and specificity of blood-based tests for asynuclein.
In summary, asynuclein presents several diagnostic implications that are rapidly evolving. The effective use of this protein as a biomarker has potential to improve the diagnostic accuracy and timing for targeted treatment in neurodegenerative diseases. Continued research will be critical in establishing standardized protocols for testing that can be universally applied.
Therapeutic Approaches Targeting Asynuclein
The pursuit of effective therapeutic strategies targeting asynuclein is crucial in mitigating the effects of neurodegenerative diseases. Due to the protein's central role in conditions like Parkinson's disease, any advancements in treatment could have significant implications for patient care. Approaches are diverse, ranging from the development of small molecule inhibitors to innovative therapies like gene therapy. Each method carries distinct characteristics and benefits that are foundational to current research.
Small Molecule Inhibitors
Small molecule inhibitors represent a promising avenue in the landscape of asynuclein-related therapies. These compounds are designed to bind to asynuclein and prevent its aggregation. The aggregation of asynuclein is a hallmark of several neurodegenerative diseases.
- Mechanism of Action: Small molecule inhibitors can disrupt the nucleation process of asynuclein, thus halting the formation of toxic aggregates. This helps to maintain neuronal health by reducing proteotoxic stress, which is detrimental to cell function.
- Examples: Certain compounds, such as CGP3466B and ANP, have been identified as potential inhibitors. These molecules have shown promise in preclinical models by enhancing cellular proteostasis.
- Challenges: Despite the potential, delivery and specificity remain considerable hurdles. Achieving adequate concentrations of these inhibitors in the brain, while minimizing side effects in other systems, is critical to their success.
Immunotherapy Strategies
Immunotherapy strategies aim to harness the body's immune system to combat the accumulation of asynuclein. This approach can take several forms, particularly monoclonal antibodies designed to target and clear misfolded asynuclein from the brain.
- Monoclonal Antibodies: Antibodies like PRX002 and ABBV-181 are being developed for their potential to bind to pathological forms of asynuclein, facilitating their clearance by immune cells.
- Benefits: This strategy not only reduces the toxic load of asynuclein but may also modulate the overall immune response, providing a dual benefit in treating neurodegeneration.
- Considerations: Challenges include ensuring specificity to avoid collateral damage to normal proteins. Furthermore, the risk of inflammatory responses is a concern that requires thorough investigation.
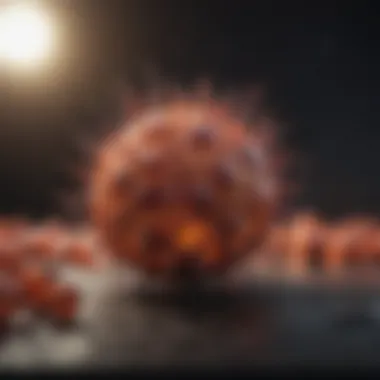
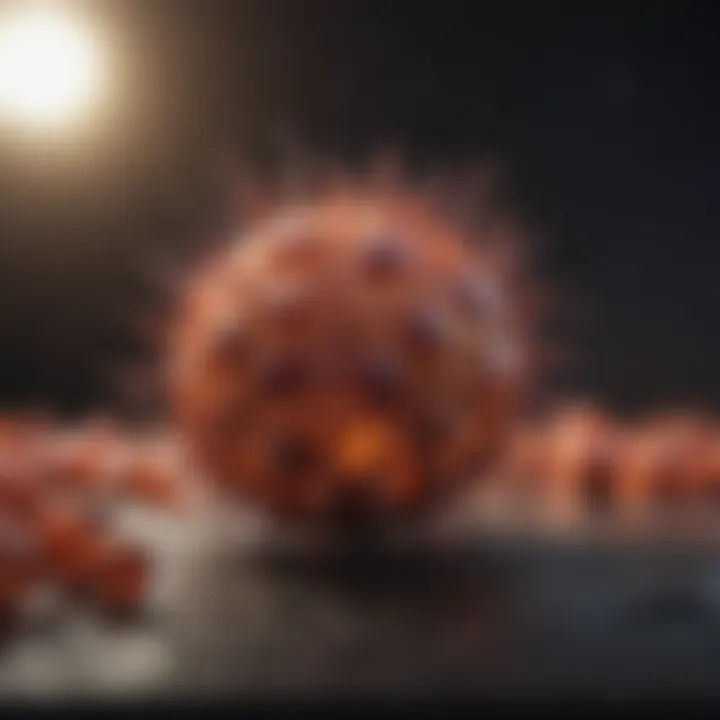
Gene Therapy Prospects
Gene therapy offers a transformative approach by altering the expression of asynuclein at the genetic level. This could involve downregulating the expression of the SNCA gene, which encodes for asynuclein, thereby reducing its production.
- Techniques: Methods such as CRISPR/Cas9 and viral vector systems are under investigation to facilitate precise gene editing or delivery, allowing targeted intervention at the source of pathology.
- Potential Outcomes: The reduction of asynuclein levels could slow disease progression, addressing one of the critical pathways of neurodegeneration.
- Challenges in Implementation: However, gene therapy is still in its infancy. Safety, ethical considerations, and regulatory approval are paramount before this can become a mainstream treatment.
"The quest for effective therapeutic approaches targeting asynuclein is not simply about treating symptoms but fundamentally altering disease progression for better patient outcomes."
Challenges in Asynuclein Research
The study of asynuclein continues to propel scientific inquiry into neurodegenerative diseases. However, this research faces several significant challenges that impede the progression toward effective therapies and better understanding of these diseases. Acknowledging these challenges is essential for researchers and clinicians alike, as it highlights areas requiring focused attention.
Understanding Protein Aggregation Dynamics
Understanding how asynuclein aggregates is crucial. It is well-known that misfolded proteins can aggregate into structures such as oligomers and fibrils. These aggregates have toxic properties that lead to neuronal death. Recent studies suggest that even small aggregates can be damaging. Researchers must identify the conditions and mechanisms facilitating aggregation. This involves not only biochemical assays but also advanced imaging techniques. Collaboration between biochemists and cell biologists may help unravel these dynamics more effectively.
Establishing Reliable Animal Models
Reliable animal models are extremely important in asynuclein research. These models should mimic human disease pathology closely. However, there is often a gap between findings in lab settings and actual human diseases. Current models like transgenic mice can replicate some features of Parkinson’s disease. Still, they do not capture the full complexity of the disease. Variations exist among human populations, complicating model creation. Hence, developing better animal models remains a priority. Progress in stem cell technology may provide new avenues to explore.
Translating Research into Clinical Practice
Translating research findings into clinical practice is one of the biggest hurdles. There are numerous promising leads from basic science that simply do not work in clinical settings. The challenge lies in ensuring that therapies targeting asynuclein aggregation are safe and effective in humans. Regulatory frameworks are stringent. They demand rigorous testing and validation, which takes time and resources. More collaboration between researchers and clinicians can enhance the swift movement of findings from bench to bedside. It could also foster integrated approaches to therapy that address multiple facets of neurodegeneration.
"Notably, the ongoing challenges in asynuclein research highlight the need for persistent efforts and innovative strategies to bridge gaps between basic and clinical science."
In summary, these challenges in asynuclein research remain critical. Addressing them could pave the way for breakthroughs that might improve the lives of those affected by neurodegenerative diseases.
Future Directions in Asynuclein Research
The future of asynuclein research plays a crucial role in understanding neurodegenerative diseases. Continued exploration is imperative to grasp the multifaceted nature of this protein and its implications in various conditions, especially Parkinson's disease. As scientific inquiry progresses, several key elements will shape the trajectory of research.
Emerging Technologies
Advancements in technology will significantly enhance our understanding of asynuclein. Novel imaging techniques, such as positron emission tomography (PET), provide real-time visualization of protein aggregation in living organisms. These methods allow scientists to track asynuclein's pathological changes in the brain, offering insights into the progression of diseases.
Moreover, single-molecule imaging techniques are becoming more prominent. They enable the observation of asynuclein at the molecular level, revealing dynamics that were previously obscured. By understanding the interactions and conformations of asynuclein, researchers can better comprehend its role in neuron degeneration.
Collaborative Research Initiatives
The complexity of asynuclein-associated diseases necessitates collaboration across scientific disciplines. Partnerships between universities, research institutions, and pharmaceutical companies are vital. Collaborative platforms foster sharing of data, methodologies, and resources, driving innovation in treatment development and diagnostics.
For example, ongoing initiatives may include consortia focusing on specific asynuclein variants and their implications. By pooling expertise, these collaborations can accelerate research efforts, leading to a holistic approach in addressing the challenges presented by neurodegenerative diseases.
Long-Term Research Goals
Setting precise long-term research goals is essential. A primary aim should be to elucidate the pathway by which asynuclein aggregates contribute to neurodegeneration. Understanding these mechanisms can pinpoint therapeutic targets for intervention.
Additionally, investigations into prevention strategies involving asynuclein are critical. Research should focus on how lifestyle choices or dietary factors may influence asynuclein metabolism and aggregation. Another goal should involve the development of effective biomarkers for early detection. Early diagnosis can significantly alter disease management, improving patient outcomes.
"Understanding asynuclein is not just about tackling existing diseases but also about preventing future occurrences."
By concentrating on these areas, researchers can forge a path toward breakthroughs that may reshape the landscape of treatment for neurodegenerative diseases.
Epilogue
The significance of asynuclein's role in neurodegenerative diseases is evident throughout the exploration in this article. Neurodegenerative diseases, particularly Parkinson's disease, showcase the complex interplay involving asynuclein, genetics, and environmental factors. Understanding these connections is not only critical for grasping the underlying mechanisms of these diseases but also essential for developing strategies to combat them. This conclusion encapsulates several key elements:
- Summary of Key Findings: The structure and functions of asynuclein have been meticulously studied. Its involvement in synaptic function, neurotransmitter release, and impact on neuronal survival elucidate the multifaceted nature of this protein. The pathological mechanisms, including aggregation, toxicity, and interactions with other cellular systems, highlight how asynuclein contributes to neuronal degeneration.
- Implications for Future Research: The ongoing studies of asynuclein set the stage for potential breakthroughs in diagnostics and therapies. Emphasizing the need for collaborative approaches in research can lead to a deeper understanding of asynucleinopathies. Moreover, advancements in technology and novel therapeutic options could redefine current treatment paradigms and offer hope for effective intervention.
Research into asynuclein will continue to evolve. As scientists reveal more about its role in neurodegeneration, both theoretical and applied aspects will be transformed.
"In studying asynuclein, we not only unlock its mysteries but also attempt to unlock a future free from the limitations of neurodegenerative diseases."
Through this article, a path forward is envisioned where the complexities of asynuclein research may lead to solutions for the challenges posed by these devastating diseases.
Key Points of References:
- Establish Credibility: Citing reputable sources such as peer-reviewed journals, established research articles, and authoritative texts enhances the article’s credibility. This aspect is especially critical when discussing complex biochemical mechanisms or neurological implications.
- Provide Context: By including references, the article places asynuclein within the wider framework of neuroscience. This helps readers grasp how this protein fits into the larger picture of neurodegenerative diseases.
- Discover Related Research: References guide the audience to additional literature. This can stimulate further exploration into aspects of asynuclein that may not be covered comprehensively within the article.
Types of References Used:
- Peer-Reviewed Journal Articles: These are vital as they offer empirical data and findings that support the discussions made.
- Books and Textbooks: They provide foundational knowledge and historical perspectives on asynuclein research and neurodegenerative diseases.
- From Reputable Websites: Information from platforms like Wikipedia and Britannica can offer supplemental details and accessible overviews.
To effectively understand asynuclein’s role in neurodegeneration, reviewing both current and historical research through cited references is imperative.
In essence, the references section is not just a list but a critical element that enriches the readers’ experience. It allows for an informed analysis of the content and signifies the depth of research involved in the article. By leveraging references properly, the narrative on asynuclein becomes more robust, informative, and interconnected with existing knowledge.