Exploring Automated Plasmid Purification Methods
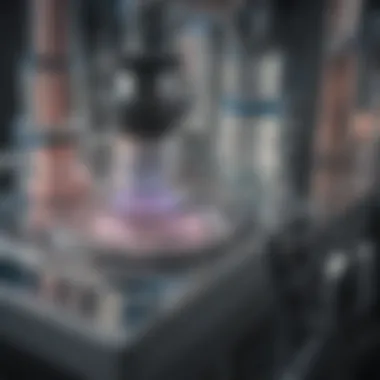
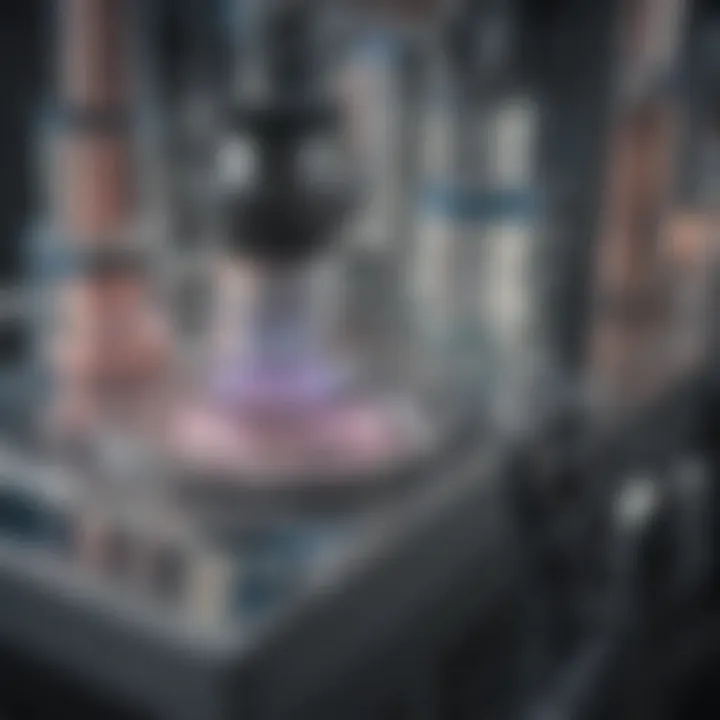
Intro
In the fast-paced realm of molecular biology, automated plasmid purification has emerged as a beacon of efficiency and reliability. With the increasing demand for high-quality plasmids for various genetic engineering applications, researchers have begun to shift from traditional manual methods to more robust automated systems. This shift is not only transformative but also crucial in optimizing laboratory workflows. What once required significant time and effort can now be accomplished with precision and minimal human intervention.
Automated plasmid purification streamlines the purification process, reducing errors and increasing throughput. By preemptively addressing common challenges associated with manual techniques, these automated solutions open up new avenues for innovation in genetic research and biotechnology. For those involved in plasmid preparation, understanding the intricacies of this technology is essential.
In this article, we will dissect the various aspects of automated plasmid purification, covering everything from underlying methodologies to practical implications and future directions.
Research Highlights
Key Findings
As the research unfolds, several key points emerge regarding automated plasmid purification.
- Efficiency: Automation has shown to significantly reduce the time required for plasmid purification from days to mere hours.
- Consistency: Repeated experiments yield comparable results, minimizing variations that can arise from manual techniques.
- Cost-Effectiveness: Though initial investments in automated systems can be steep, long-term savings related to labor and error correction offset these costs.
- Scalability: Automation allows laboratories to scale their operations without a corresponding increase in manual labor or complexity.
Implications and Applications
The implications of these findings sweep across various sectors of molecular biology. Researchers can now focus on more complex tasks without getting bogged down by labor-intensive purification processes. Applications in synthetic biology, vaccine development, and gene therapy have seen immediate benefits from these advancements. This is a real game-changer for labs aiming to keep pace with rapid scientific developments.
Methodology Overview
Research Design
The exploration of automated plasmid purification methods typically involves a combination of quantitative and qualitative research design. This approach allows for a comprehensive understanding of both the performance of automated systems and the experiences of users within various laboratory settings.
Experimental Procedures
In studying the efficacy of automated plasmid purification, researchers employ specific procedures that include:
- Comparative analysis between traditional and automated methods.
- Evaluation of different automated systems, such as MagNA Pure and QIAcube.
- Assessment of plasmid quality via restriction enzyme digestion and gel electrophoresis.
These methods help in establishing benchmarks and best practices to enhance laboratory productivity and reliability.
The findings and analyses presented here form the backbone of a more profound understanding of automated plasmid purification and its far-reaching benefits within the scientific community.
Prolusion to Automated Plasmid Purification
In the ever-evolving landscape of molecular biology, the role of plasmid purification cannot be overstated. As we explore the world of automated plasmid purification, it’s crucial to consider its implications not only for researchers but also for the wider field of genetic engineering. In this context, automated methods offer a fresh perspective, marrying efficiency with precision to streamline laboratory workflows.
Importance of Plasmid Purification
Plasmids, those small circular DNA molecules, are essential tools in cloning, gene expression, and various biotechnological applications. To successfully harness their potential, it's imperative that researchers ensure high-quality purification. This process isn’t just about isolating plasmid DNA; it impacts downstream applications, influencing the quality of results in experiments like transfections or generating recombinant proteins.
When you think about it, plasmid purification is like polishing a diamond before showcasing it. If the base material isn’t up to par, any work that follows can quickly become difficult, if not impossible. Therefore, the significance of this purification process goes beyond technicality—it’s about achieving reliable, reproducible outcomes that can stand up to scrutiny.
Overview of Automation in Molecular Biology
With the surge in demand for higher throughput and efficiency, automation in molecular biology has become a linchpin in laboratory practices. From high-tech liquid handling robots to integrated workstations, the tools available have transformed how researchers approach mundane yet critical tasks.
Automation isn’t merely about speeding up processes; it’s about enhancing accuracy and minimizing human errors. These devices are designed to perform repetitive tasks with meticulous precision, which significantly reduces the chances of mistakes that can compromise results. Additionally, automation enables the simultaneous processing of multiple samples, making it an imperative in high-throughput environments.
Some of the key benefits of automation in molecular biology include:
- Time-saving: Reduces the duration of tedious pipetting and washing protocols.
- Consistency: Maintains uniformity across sample processing, ensuring reliable outcomes.
- Ease of use: Intuitive software allows even novice users to navigate complex procedures.
As we navigate through this overview, it will become evident each of these elements contributes to the overarching goal of advancing plasmid purification processes, ultimately propelling research forward.
Understanding Plasmids
The significance of comprehending plasmids cannot be overstated when it comes to the broader scope of automated plasmid purification. Plasmids are the unsung heroes of genetic engineering and molecular biology, serving as essential tools in various research and therapeutic applications. These small, circular DNA molecules are pivotal for cloning, sequencing, and gene expression. Knowing the ins and outs of plasmids, including their definition, composition, and roles in gene cloning, enriches our understanding of why plasmid purification is crucial and how it can be automated effectively.
Definition and Composition of Plasmids
Plasmids are essentially dinky DNA circles that dwell outside the chromosomal DNA within a cell. They are usually found in bacteria, though they can also be present in some eukaryotic cells. Generally, a plasmid comprises a few key components:
- Origin of Replication: This is the sequence that allows the plasmid to replicate independently of the chromosomal DNA, making additional copies as the host cell divides.
- Selectable Markers: These genes, such as antibiotic resistance markers, enable researchers to identify which cells have successfully taken up the plasmid.
- Multiple Cloning Sites (MCS): This region contains several unique restriction enzyme sites, allowing easy insertion of foreign DNA.
- Promoters: These sequences drive the expression of inserted genes, permitting production of required proteins.
Understanding these elements is essential because they determine how effective a plasmid will be for a specific molecular biology application. The right combination can lead to substantial improvements in research outcomes.
Role of Plasmids in Gene Cloning
When it comes to gene cloning, plasmids play a crucial role—it’s like the bread and butter of molecular techniques. The concept of gene cloning involves transferring a gene from one organism into a plasmid, which is then introduced to a host cell. This process not only facilitates the production of multiple gene copies but also enables the synthesis of proteins for various experiments. Here’s how plasmids contribute:
- Gene Insertion: By using restriction enzymes to cut the plasmid and insert the desired gene into the MCS, researchers can create a recombinant plasmid.
- Transformation: The recombinant plasmid is introduced into competent bacteria through a process known as transformation, where the bacteria absorb the plasmid.
- Amplification: As the bacteria multiply, they reproduce countless copies of the plasmid, resulting in high yields of the cloned gene.
- Expression: Finally, the inserted gene can be expressed, allowing scientists to study its function or produce the corresponding protein.
In summary, plasmids are not just crucial; they are the bedrock for many genetic engineering endeavors. Their structure and role in gene cloning highlight their importance in both laboratory settings and practical biotechnology applications. Understanding these concepts sets the stage for appreciating the intricacies of automated plasmid purification, a process that aims to optimize the outputs from these genetic wonders.
Traditional Plasmid Purification Methods
In the world of molecular biology, the reliability and quality of plasmid DNA are crucial for many applications, including research and therapeutic processes. Traditional plasmid purification methods have long been the backbone of obtaining high-quality plasmid DNA from bacterial cultures. Each technique not only serves as a foundation for automated processes but also offers benefits that can be pertinent to understanding the evolution of purification methods. The importance of these traditional methods spans across fields, providing solid insights into the nature of plasmids and their behaviors during isolation.
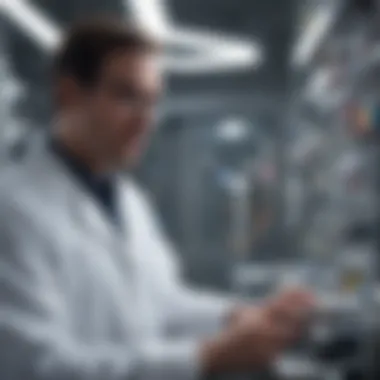
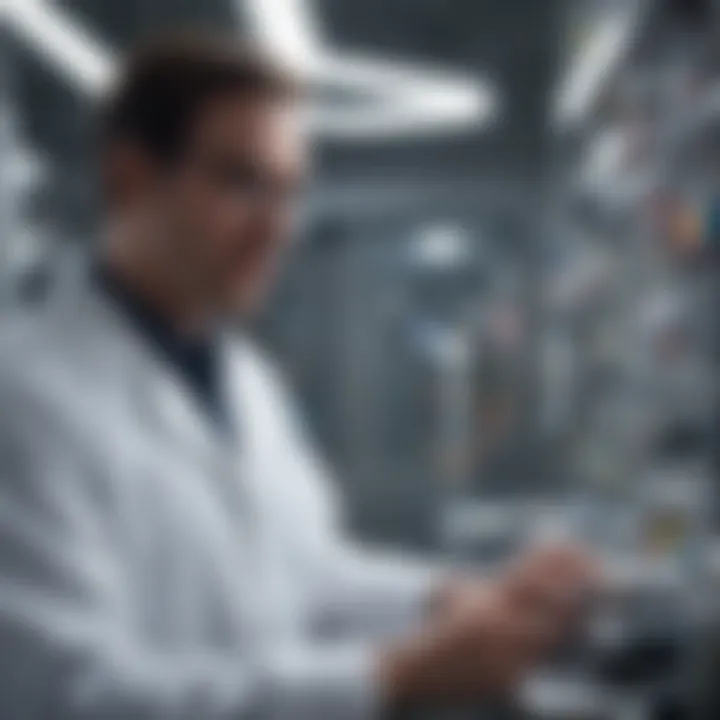
Alkaline Lysis Protocol
The alkaline lysis protocol stands as one of the most commonly employed methods for plasmid DNA extraction. It typically involves the lysis of bacterial cells using a combination of alkaline buffer solutions. The procedure is quite straightforward and typically follows these steps:
- Cell Suspension: Bacteria are harvested and resuspended in a buffer (often called resuspension buffer) that maintains a gentle environment for cell stability.
- Lysis: An alkaline solution is added to disrupt the cellular architecture, causing the contents, including the plasmid, to leak into the solution.
- Neutralization: After the lysis, a neutralization buffer is introduced. This changes the pH, allowing for the separation of plasmid DNA from cellular debris.
- Precipitation: The solution is then centrifuged to precipitate the cellular debris, and the supernatant containing plasmid DNA is carefully collected.
- Purification: Further purification steps may include ethanol precipitation or column-based methods to achieve higher purity levels.
While efficient, this method can yield variability in plasmid quality due to different factors such as cell growth conditions or lysis efficiency. Variability in plasmid isolation can lead to inconsistent results in downstream applications.
Phenol-Chloroform Extraction
Another classic method, phenol-chloroform extraction, revolves around the use of organic solvents to separate biomolecules based on their solubility properties. Here’s a simplified outline:
- Mixing: After the initial lysis of bacteria, an equal volume of phenol-chloroform is added to the lysate. This mixture selectively partitions the plasmid DNA into the aqueous phase.
- Centrifugation: When the mix is centrifuged, two distinct layers form: the lower organic phase containing proteins and cell debris, and the upper aqueous phase containing the plasmid DNA.
- Collection: The upper aqueous phase is carefully collected, often followed by a wash step using buffer solutions to remove residual phenol and chloroform.
Although effective in purifying DNA, this method is known to be labor-intensive and requires careful handling of hazardous substances, which poses risks and adds to operational complexity.
Centrifugation Techniques
Centrifugation is a staple in molecular biology, especially when it comes to the purification of plasmid DNA. This method typically comes into play after initial lysis has occurred. Given its role, understanding how centrifugation works is vital. Centrifugation utilizes centrifugal force to separate substances of different densities within a solution. Here are the key points regarding its operation in plasmid purification:
- Separation: Following cell lysis and any additional purification steps, centrifugation helps settle cellular debris while the plasmid DNA remains suspended in the supernatant.
- Concentration: By adjusting time and force, researchers can concentrate plasmid solutions, which is essential when low DNA yields are observed.
- Variable Conditions: Centrifugation methods may vary based on the type of rotor, temperature, and time settings, making it adaptable for different laboratory environments.
Nevertheless, challenges can occur with centrifugation, such as potential shearing of DNA or loss of yield during transfers between tubes. These considerations make understanding these traditional methods paramount in a research setting.
The success of downstream applications often hinges on the quality of plasmid DNA, making traditional purification methods more relevant than ever as we explore automated solutions.
Principles of Automated Plasmid Purification
Automated plasmid purification has increasingly become a cornerstone in the molecular biology lab, marrying efficiency with precision. At its core, this topic highlights the substantial implications of integrating automation technology into traditional purification practices. Automation not only streamlines the process but also enhances the quality and reliability of plasmid isolation.
Automation Technologies in Analytical Chemistry
Automation technologies have been revolutionary in the field of analytical chemistry, particularly when it comes to plasmid purification. They contribute to significant improvements in various analytical methods, primarily by enhancing throughput and accuracy. Methods such as liquid chromatography and mass spectrometry, when automated, not only reduce sample handling time but also minimize the risk of human error, thus leading to more reproducible results.
In plasmid purification, automation technologies allow for consistent results across multiple samples, making it easier to scale up experiments while maintaining reliability. These advancements are crucial, especially in high-throughput environments where time and efficiency are of the essence.
Types of Automated Systems
Automation in plasmid purification typically involves two main types of systems: liquid handling robots and integrated workstations. Each presents distinct features that cater to differing laboratory needs.
Liquid Handling Robots
Liquid handling robots stand out for their capability to perform precise and repetitive tasks—qualities that are invaluable in plasmid purification. Their key characteristic lies in their ability to manage liquid transfers with exceptional accuracy. This minimizes the variability that often comes with manual pipetting, thus ensuring more reliable outcomes. Their high throughput capacity allows for the simultaneous processing of numerous samples, making them a popular choice in busy or growing labs.
However, these robots do have a few disadvantages. For instance, they can be somewhat expensive, and the initial setup may require specific programming skills that not all lab personnel possess. Nonetheless, the investment often pays off, considering the efficiency and reliability gains achieved.
Integrated Workstations
On the other hand, integrated workstations combine various automated processes into a single system, offering a holistic approach to laboratory workflows. The main allure of integrated workstations is that they allow for comprehensive automation—from sample preparation to purification—within one platform. This integration simplifies the workflow, reduces the chances of cross-contamination, and saves precious lab time.
"Integrated workstations bring together multiple tasks into one seamless operation, enhancing efficiency and accuracy."
However, the drawback might be the initial cost, which is typically higher than standalone systems, as well as the complexity that comes with managing an all-in-one unit. Despite those concerns, their user-friendliness and simplified operations often make them an attractive choice for researchers looking to maximize their lab's efficiency.
The examination of these automation systems provides a clearer understanding of their roles in enhancing plasmid purification techniques. Each system, whether a liquid handling robot or an integrated workstation, embodies unique strengths and considerations, all of which contribute to the overarching goal of improving laboratory productivity and reliability.
Methodologies in Automated Plasmid Purification
In the realm of molecular biology, the methodologies employed in automated plasmid purification stand as pivotal pillars. These techniques not only define the efficiency of plasmid yield but also the purity levels that researchers can achieve. With the growing demand for high-throughput genetic analyses, understanding these methodologies is essential for any lab aiming for excellence. The right technique can vastly lower contamination risks and improve overall performance in experimental tasks.
Implementing advanced methodologies translates into substantial benefits:
- Consistency in Results: Automated systems minimize human variability, resulting in more reproducible and reliable outcomes.
- Efficiency: Automation streamlines complex processes, shortening execution time from hours to mere minutes.
- High Throughput: Laboratories can process a greater number of samples simultaneously, paving the way for larger studies.
These methodologies are not a one-size-fits-all. Different approaches cater to various requirements depending on the specific application or plasmid type. Let's delve into the three primary methodologies utilized in automated plasmid purification.
Silica-based Columns
Silica-based column purification is a widely embraced methodology within the automated plasmid purification landscape. This technique exploits silicon dioxide's unique properties to adhere to plasmid DNA while allowing impurities to be washed away. The process is quite straightforward and involves several essential steps:
- Lysis of Cells: Bacterial cells containing the plasmid are lysed using alkaline solutions to release the DNA into solution.
- Binding: The lysate is processed through a silica column where plasmid DNA binds to the silica, effectively separating it from contaminants like proteins and RNA.
- Washing: Contaminants are rinsed away using a wash buffer, ensuring that only pure plasmid DNA remains.
- Elution: Finally, the purified plasmid DNA is eluted from the silica using an elution buffer.
This methodology is favored due to its simplicity and effectiveness. It allows researchers to get highly purified plasmids quickly, making it appropriate for downstream applications such as cloning and sequencing.
Magnetic Bead-based Methods
Magnetic bead-based purification methods have grown increasingly popular for their versatility and efficiency. This approach involves the use of magnetic beads coated with materials that preferentially bind to nucleic acids. Researchers can simply add the beads to a lysate, mix them thoroughly, and apply a magnetic field to facilitate the separation of the bound plasmid from the liquid debris.
Here’s a closer look at how it works:
- Lysis: Similar to the earlier methodology, bacterial cells are lysed to free the plasmid DNA.
- Binding: Once the magnetic beads are introduced, they interact with plasmid DNA.
- Magnetic Separation: Using a magnet, researchers can easily pull the beads—and thus the bound DNA—out of the solution, leaving impurities behind.
- Washing and Elution: The beads are washed to remove contaminants before eluting the purified plasmid DNA.
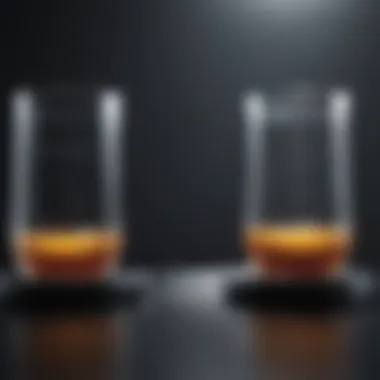
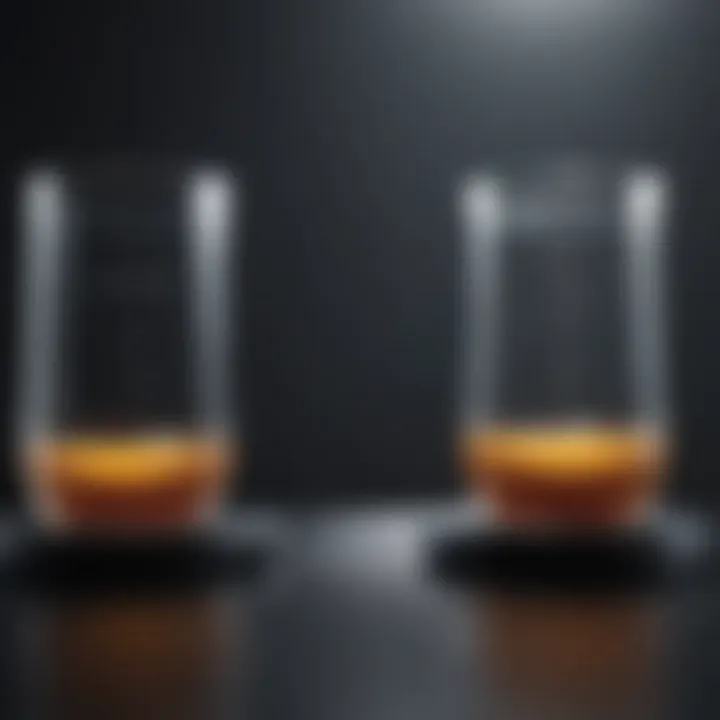
This method boasts several advantages, including reduced handling time and fewer overall steps compared to silica-based methods. Its flexibility makes it suitable for different scales of purification, from mini-preps to larger processes.
Anion Exchange Chromatography
Anion exchange chromatography is a more specialized technique that utilizes the charge properties of DNA for purification. This method is predicated on the differential binding affinities of plasmid DNA to an anion exchange resin, depending on the pH of the elution buffer. In practice, it often involves:
- Sample Application: The lysate containing plasmid DNA is loaded onto a positively charged column.
- Binding Depiction: Plasmid DNA, which carries a negative charge, binds to the column while other impurities remain unbound.
- Gradual Elution: By gradually altering the salt concentration in the buffer, researchers can selectively elute plasmid DNA while retaining unwanted contaminants.
This methodology allows for exceptionally high purity levels, making it ideal for sensitive applications like large-scale productions or when the DNA needs to be used in high-precision techniques such as transfection.
"Different methodologies offer unique advantages and cater to various research needs. Understanding these differences is vital for selecting the right technique for your applications."
In sum, the methodologies employed in automated plasmid purification are critical to achieving desired research outcomes. By tailoring the choice of method to specific experimental needs, researchers can enhance not only their workflow efficiency but also the quality of their results.
Advantages of Automation in Plasmid Purification
The rise of automation in molecular biology is not just a trend; it represents a transformative shift in how researchers approach plasmid purification. Automated systemshave quickly become essential tools in laboratories, bringing a host of advantages that streamline workflows and elevate the quality of scientific outcomes. In this section, we will explore key benefits: increased throughput, enhanced consistency and reproducibility, and a significant reduction in human error.
Increased Throughput
One of the most compelling reasons to adopt automated plasmid purification is the boost in throughput. Manual methods can be slow and labor-intensive, limiting the number of samples that can be processed concurrently. In contrast, automated systems can handle multiple samples with minimal intervention. This capability translates into a considerable time savings, enabling labs to pivot from processing a handful of samples to tackling dozens, or even hundreds, simultaneously.
This higher capacity does more than just speed things up; it also means that researchers can conduct more experiments in a given timeframe. Moreover, the efficiency gained allows for faster validation of results, which is essential in fast-paced research environments.
"In laboratories where time is of the essence, automation acts like a breath of fresh air, allowing researchers to focus on the science rather than the mundane operations."
Improved Consistency and Reproducibility
When it comes to scientific research, consistency is king. Automated systems excel in this area by eliminating the variability often associated with manual techniques. Each cycle of purification can be standardized, ensuring that the same protocols are followed every time. This leads to outputs that are not only reliable but also highly reproducible across different experiments and laboratory settings.
For instance, the precision of liquid handling robots is unparalleled; they can dispense reagents in minute, accurate volumes, reducing the likelihood of discrepancies that can skew results. With automation, researchers gain the confidence that their plasmid yields will meet expectations, regardless of when or who performs the experiment.
Reduction of Human Error
Human error is a persistent challenge in laboratory settings. From inconsistent pipetting to mislabeling samples, the potential for mistakes can compromise entire projects. Automation addresses this concern directly. With automated systems, the risk of human error diminishes significantly because many of the laborious steps are executed by machines programmed to follow precise protocols.
This is especially critical in high-stakes environments where even a small mistake can derail long-term experiments or skew results. By automating the process, researchers can alleviate stress and focus on more complex aspects of their work, knowing that the procedural elements are in capable hands.
In summary, the advantages of automation in plasmid purification are not merely incremental; they represent a paradigm shift in how laboratories function. As the field continues to evolve, embracing these technologies opens up possibilities for innovation and improved outcomes.
Challenges in Automated Plasmid Purification
As the field of molecular biology steadily integrates automation into various procedures, the journey of plasmid purification using automated systems is not without its bumps. While automation presents significant advancements in efficiency and reliability, it also brings along its unique set of challenges. Understanding these challenges is paramount as it allows researchers and professionals in the field to navigate the complexities involved with automated systems, ensuring the highest quality of outcomes in their experiments.
Equipment Limitations
One of the foremost challenges in automated plasmid purification arises from the limitations in the equipment used for these processes. Many purification systems are designed with specific protocols in mind, which may not accommodate variations in sample types or impurities present in the plasmid preparations. For instance, while some machines may excel at handling basic bacterial lysates, they might falter when faced with samples containing residual proteins or cellular debris that could impede purification efficacy.
Furthermore, issues such as incompatibility with certain reagents or the need for regular calibration and maintenance can lead to unexpected downtime.
- Frequent calibration: Ensuring that the equipment operates within specification can require consistent recalibrations, diverting valuable lab resources.
- High maintenance costs: Instruments can demand regular servicing, contributing to overhead.
- Limited throughput: Some systems may not handle high volumes efficiently, creating bottlenecks during critical research timelines.
Developers of these systems must continuously refine their technology to enhance adaptability to varied sample conditions, thus ensuring the efficacy of automated plasmid purification methods.
Cost Considerations
Automated systems can carry a hefty price tag, which often raises eyebrows among budget-sensitive labs. The initial investment required for high-quality equipment can be intimidating, especially for smaller institutions or startups that may not have the financial backing of larger organizations. This leads many to weigh the benefits against the costs meticulously.
- Equipment Purchase Costs: High-end automated systems can range widely in pricing; however, investing in the latest technology might seem unfeasible for smaller labs.
- Operational Costs: In addition to the initial outlay, recurring expenses for consumables and specialized reagents can escalate quickly, further burdening the budget.
- Risk vs. Reward: The potential for increased efficiency must be matched against these initial and ongoing costs, requiring savvy decision-making regarding resource allocation.
Though there are long-term savings associated with reduced labor and increased throughput, it’s often a hard pill to swallow upfront. As a result, a thorough cost-benefit analysis becomes essential to make informed decisions about integrating automated solutions into plasmid purification protocols.
Technical Expertise Required
Last but not least, diving into automated plasmid purification is not a walk in the park when it comes to the technical expertise that is often a prerequisite.
Many systems demand a certain level of proficiency in both the operation of the machinery and the underlying biochemical principles. As modern equipment becomes more sophisticated, so does the knowledge required to troubleshoot complex issues that may arise during procedures.
- Understanding Complex Protocols: Operators must grasp detailed protocols and system operations to prevent errors that can compromise results.
- Continuous Learning: With technological advancements, continuous education and training become necessary to keep up with emerging techniques and software updates.
- Collaboration and Team Dynamics: Laboratories may find that not all personnel have equal skill sets regarding these new technologies, creating potential discrepancies in workflow if training is not uniformly applied.
Building a skilled workforce is pivotal; this can involve workshops, training sessions, or even partnerships with vendors to ensure everyone is on the same page.
"Efficient technology requires equally competent minds behind it. Advanced machinery is only as good as those who operate it."
Addressing these challenges head-on forms the cornerstone of successfully integrating automation in plasmid purification. By recognizing equipment limitations, carefully analyzing costs, and investing in the necessary technical expertise, laboratories can better harness the promise that automated systems deliver, leading to improved outcomes in genetic engineering endeavors.
Troubleshooting Common Issues
When dealing with automated plasmid purification, one can't ignore the real-world challenges that researchers face. Addressing these problematic situations is not just about saving time; it’s also about ensuring that the results are reliable and valid in experiments. Therefore, this section is dedicated to troubleshooting common issues that may arise during the purification process. Understanding these hiccups helps researchers navigate their workflows smoothly, reduces frustrations, and ultimately leads to better outcomes.
Dealing with Contamination
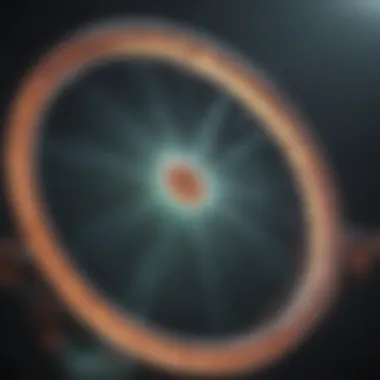
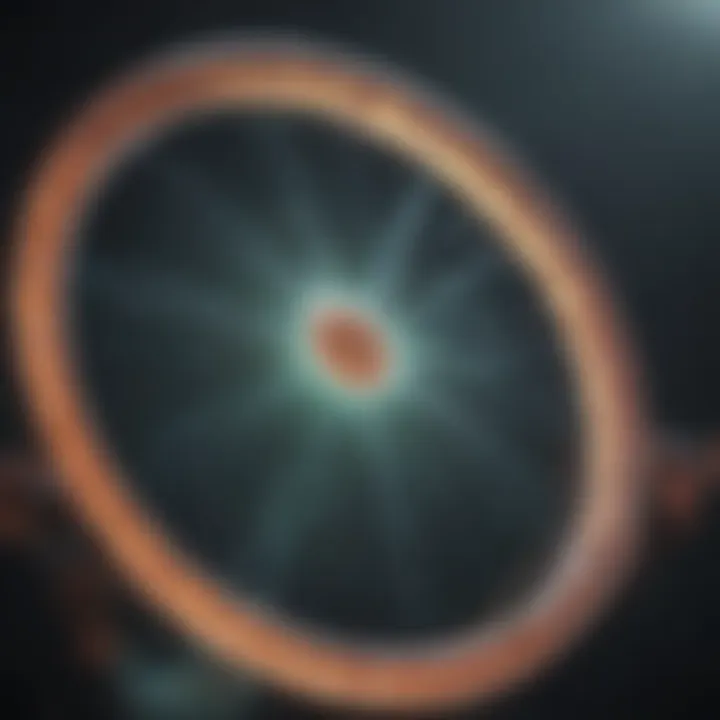
Contamination can be a pervasive problem when purifying plasmids. It arises when unwanted nucleic acids or cellular components inadvertently mix with the plasmid DNA during the purification process. This can stem from various sources, from inadequately prepped materials to cross-contamination between samples.
To effectively manage this issue, it is vital to incorporate a few best practices into the purification workflow:
- Maintain Clean Workspaces: Always work in a sterile environment to minimize the risk of contamination. Regularly clean surfaces and ensure that all equipment is properly sanitized.
- Use Filtered Tips: Employing filtered pipette tips can help in reducing aerosol contamination when transferring fluids.
- Quality Control Checks: Running control samples alongside experimental samples helps in identifying any contamination early, allowing for corrections.
- Monitor Reagent Quality: It’s important to use high-purity reagents, as lower quality materials can introduce contaminating substances into your samples.
By implementing these strategies, researchers can fortify their purification protocols against contamination, leading to cleaner, more reliable outcomes.
"An ounce of prevention is worth a pound of cure."
Addressing Low Yields
Low yields during plasmid purification can be incredibly frustrating, particularly when high concentrations of plasmid DNA are necessary for downstream applications. Several factors could contribute to this dilemma, ranging from issues with the lysis step, inefficient binding to purification columns, to poorly prepared growth media.
To effectively tackle low yield issues, researchers might consider:
- Optimizing Cell Growth Conditions: Ensuring that bacteria are cultured under optimal conditions, including the right temperature and duration, can significantly impact yields.
- Adjusting Lysis Conditions: Re-evaluating the alkaline lysis conditions may uncover suboptimal practices. For example, not lysing cells completely will obviously hinder yield.
- Increasing Binding Capacity: When using silica columns or magnetic beads, ensure that you’re allowing sufficient time for nucleic acids to bind adequately. Adjusting the volume of wash solutions can also help improve yield.
- Evaluating Elution Conditions: The choice of elution buffer and conditions can impact the final yield. Choosing a buffer that promotes better release of DNA from the matrix is crucial.
Each element in the purification process is interconnected, and pinpointing the area causing a yield drop can be complex yet critical. Frequent evaluation and fine-tuning of these steps go a long way in ensuring that you achieve the desired yields necessary for successful experimental outcomes.
By understanding the nuances of troubleshooting contamination and low yields in automated plasmid purification, researchers are better equipped to enhance their methodologies, ultimately leading to more reliable results.
Cost-Efficiency of Automated Systems
In the realm of molecular biology, where precision and reliability reign supreme, the cost-efficiency of automated systems for plasmid purification is a hot topic. Researchers are increasingly aware of the balance between initial investment and subsequent savings. While many are drawn to the shiny allure of automation, understanding its financial implications can prove crucial for making informed decisions.
Initial Investment vs. Long-term Savings
When considering automated systems for plasmid purification, the initial costs can seem daunting. Equipment like liquid handling robots and integrated workstations may require a substantial outlay. This can discourage some institutions, particularly smaller labs with tighter budgets. However, it’s important to look beyond the sticker price.
Take, for example, a typical lab that handles a large number of samples each week. Implementing a robotic system could significantly cut down on manual labor cost. Once the machinery is set up, the time saved translates into greater productivity. Researchers can focus their efforts on analysis and experimentation instead of monotonous pipetting tasks. The long-term efficiencies gained often outweigh the initial investment, making automation a worthwhile endeavor.
Additionally, automated systems reduce the risk of human error, which can lead to costly mistakes down the line. These system errors can contribute to low yields or even contamination, causing researchers to repeat experiments. The costs of reagents, equipment wear, and downtime can spiral quickly, reinforcing the necessity for an automated approach. Thus, it’s clear that when you add up all expenses, automation tends to pay for itself over time.
Cost Analysis in Research Environments
Examining the financial implications of automated systems shows that investment goes hand in hand with operational effectiveness. In many research environments, the benefits can be quantifiable. For example, let’s say a particular lab processes a hundred samples per week. With automation, they might reduce the time spent on purification from hours to mere minutes. Not only does this increase throughput, but it also allows for a more flexible schedule that can accommodate fluctuations in demand.
"Shifting to automated plasmid purification can turn a busy lab into a lean operation, maximizing output without proportionate increases in costs."
Moreover, with the increasing complexity of projects and genome manipulation, automated systems can help ensure consistency and reproducibility. This is crucial, especially in environments where regulatory compliance is paramount. The cost of potential missteps can be considerably high, far outweighing the investment in automation.
In essence, a well-planned deployment of automated purification systems can lead to significant financial benefits in research environments. The operational costs, maintenance expenses, and labor requirements must all be factored in to draft a clear picture of overall cost-efficiency. By focusing on these metrics, laboratories can not only enhance their workflows but also reassure funding bodies of their prudent financial management.
In summary, while the upfront expenses of automated systems can be a hurdle, their long-term savings reveal a compelling case for their adoption. Not only does it enhance productivity; it also safeguards against potential errors, proving that automation is not merely a luxury but a necessity in modern molecular biology.
Future Directions in Automated Plasmid Purification
The landscape of automated plasmid purification is continually evolving, and understanding these future directions is vital to keep pace with advancements in molecular biology. The emerging technologies and the integration of artificial intelligence in laboratory settings are setting the stage for a new chapter in efficiency and accuracy in plasmid purification processes. This isn't just about having the latest gadgets; it's about how these innovations can transform the way researchers work and the results they achieve.
Emerging Technologies
The future of automated plasmid purification will likely witness a surge in innovative technologies that enhance purification efficiency and throughput. Here are a few noteworthy emerging technologies likely to shape this future:
- Miniaturization of Processes: Smaller-scale purification techniques can save time and resources, allowing for quicker turnaround and less sample waste. Techniques such as microfluidics are gaining traction in laboratories, allowing for precision handling of sample volumes.
- Real-time Monitoring Systems: Incorporating systems that allow for the real-time assessment of purification variables can lead to immediate adjustments and optimization of protocols. This could mean monitoring parameters like temperature, pH, or even the purity of plasmid DNA on the fly.
- Integration of Flowsheet Automation: The continuous flow of processes instead of batch processing can enhance efficiency. By automating entire workflows, laboratories can reduce human error and increase consistency in their results.
- Biotechnological Advances: New methods, such as enzymatic cleavage and selective filtration, are being explored. Techniques like CRISPR have potential applications for enhanced purification processes, targeting only the desired plasmids for extraction.
These advancements point to a future where the entire purification process becomes seamless, enhancing both the accuracy and the reliability of results.
Integrating AI in Laboratory Automation
As the field of laboratory automation progresses, the infusion of artificial intelligence into plasmid purification processes stands out as a promising frontier. Here's how AI could redefine this crucial area:
- Predictive Analytics: By analyzing data from previous purification runs, AI algorithms could predict the outcomes of current experiments. This predictive capability allows scientists to tweak protocols proactively rather than reactively, vastly improving experimental outcomes.
- Decision-Making Support: AI-driven systems can assist researchers in choosing the best purification methods for specific plasmid types. By considering factors such as plasmid size, purity goals, and available materials, AI can guide users towards optimal paths, saving valuable time and resources.
- Automation of Routine Tasks: Routine tasks like sample sorting and inventory management can be automated. This frees up researchers to focus on more complex analyses rather than get bogged down with repetitive lab work.
- Machine Learning for Process Optimization: AI can continually learn from various purification runs, adjusting protocols based on data to fine-tune performance. This includes optimizing the conditions under which the purification occurs, leading to better yields and purity.
Incorporating AI into plasmid purification processes will not only maximize equipment utilization but also improve overall efficiency in research endeavors, paving the way for rapid advancements in the field of genetics and biotechnology.
In summary, the future of automated plasmid purification is bright, driven by a combination of emerging technologies and the strategic use of artificial intelligence. Each advancement promises to enhance the reliability, efficiency, and throughput of purification processes, ultimately elevating the standards of research in molecular biology.
Culmination
The conclusion holds a pivotal spot in the narrative of automated plasmid purification. It’s not merely a place to wrap up discussions but a critical moment to underscore the transformative impact that this automation has on molecular biology. A thorough understanding of this topic is essential, especially in the context of ongoing advances in biotechnology.
Recapitulation of Key Points
In summarizing the main facets of automated plasmid purification, several crucial points emerge:
- Efficiency Gains: Automation significantly boosts throughput, allowing researchers to process more samples in a shorter timeframe. By minimizing manual interventions, the likelihood of inconsistencies is greatly reduced.
- Cost Implications: While the initial setup costs can be daunting, the long-term financial benefits are clear. The reduction in labor-intensive steps and the minimization of errors lead to better budget management over time.
- Technological Advancements: The integration of cutting-edge technologies, such as AI-driven systems and highly precise robotic mechanisms, enhances accuracy and reliability in plasmid purification.
- Industry Adaptation: As more labs pivot towards automation, understanding these systems becomes increasingly relevant for researchers and educators looking to remain at the forefront of their fields.
The benefits of automation extend beyond improved laboratory metrics; they touch on the fundamental ways scientific research can evolve. The narrative clearly shows that investing in automated techniques is not just about trends but about future-proofing research capabilities.
The Role of Automation in Modern Biotechnology
Automation represents a cornerstone of modern biotechnology, influencing various aspects of research, development, and production. Its role is profoundly integral and multifaceted. Consider the following:
- Enhancing Reproducibility: With automated systems, experiments can be replicated with higher precision than manually conducted ones. This leads to results that can be trusted to comply with regulatory standards, a crucial element in fields like medicine and pharmaceuticals.
- Facilitating High-Throughput Screening: The ability to quickly screen numerous samples allows for rapid identification of potential candidates in drug discovery and genetic research, accelerating the pace of innovation.
- Reducing Skilled Labor Dependency: In a field where technical expertise is vital, automation allows researchers to focus on higher-level analysis rather than repetitive tasks. As a consequence, this can lead to a greater pool of candidates entering the industry, as reliance on specialized skill sets diminishes.
- Paving Way for Innovation: Through improved efficiency and reliability, researchers are encouraged to explore novel hypotheses and experimental designs that may have previously been too time-consuming or impractical due to resource constraints.
Automation does not replace human intellect; rather, it empowers it, providing tools that allow researchers to pursue deeper inquiry with efficient and reliable methods.
In summary, the conclusion of this article not only wraps up the various aspects of automated plasmid purification but also reaffirms its importance in shaping the future of biotechnology. Through a solid grasp of these principles, students, researchers, and professionals can better navigate the evolving landscape of molecular biology.