Beams Design: Key Principles and Applications
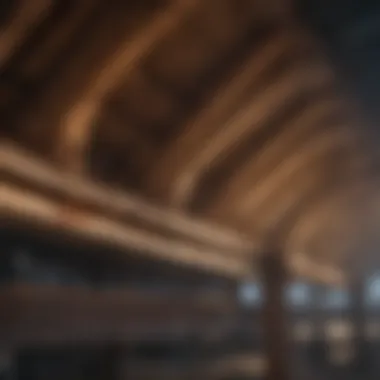
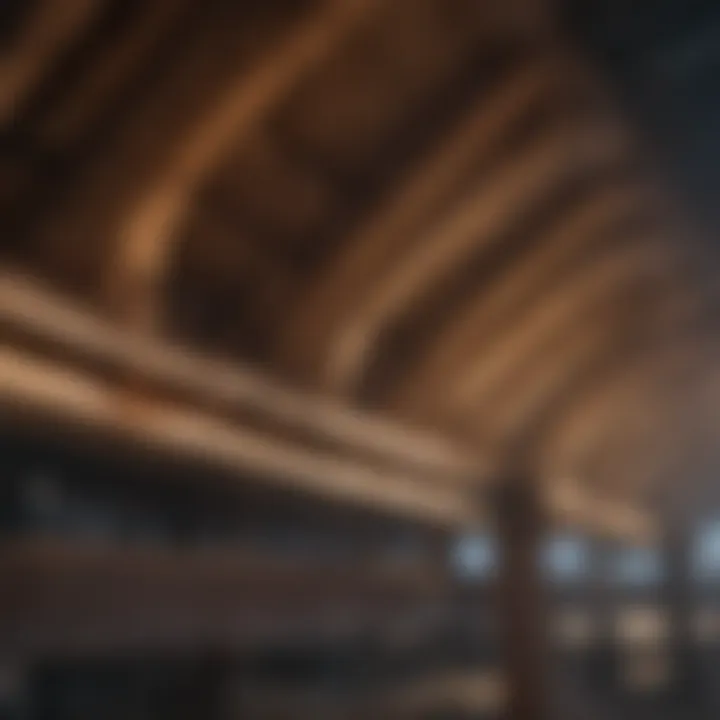
Intro
Beam design serves as a critical aspect of civil and structural engineering. It encompasses a variety of considerations that engineers must address to create safe and effective structures. Understanding these principles is essential for students, researchers, and industry professionals alike. This article will explore fundamental concepts, methodologies, and modern applications tied to beam design.
In engineering, beams are structural elements that support loads and transfer those loads to supporting structures. Common applications include bridges, buildings, and various mechanical components. The significance of analyzing different types of beams becomes apparent as engineers seek to optimize their designs for strength, stability, and functionality.
Emerging technologies, such as software modeling and advanced materials, also play a vital role in beam design. These advancements allow for more precise and efficient engineering solutions. By examining key principles and recent trends, this article aims to offer comprehensive insights into the world of beam design, facilitating a deeper understanding of its challenges and opportunities.
Research Highlights
Key Findings
To effectively implement beam design concepts, it is crucial to focus on several key areas:
- Types of Beams: Understanding the distinctions between simply supported, cantilever, and continuous beams is fundamental. Each type responds differently to loads, which directly impacts design strategies.
- Material Selection: The choice of materials significantly affects the strength and longevity of a beam. Engineers must consider factors such as durability, weight, and cost when selecting materials like steel, concrete, or composite materials.
- Load Considerations: Load analysis is a necessary step in beam design. Engineers must account for stationary loads, dynamic loads, and environmental factors like wind and seismic activity.
- Design Standards: Adhering to established engineering codes and standards is fundamental to ensuring safety and regulatory compliance.
Designing beams without a proper understanding of the principles can lead to structural failure and safety hazards.
Implications and Applications
The implications of effective beam design extend beyond immediate structural integrity. Successful applications in engineering can lead to innovations in various domains, such as:
- Sustainability: Employing advanced materials and construction techniques can result in more sustainable designs, reducing environmental impact.
- Cost Efficiency: Optimized designs can minimize material use, leading to reduced costs in both construction and maintenance.
- Safety Enhancements: An understanding of modern design methodologies can enhance safety protocols in the engineering process.
Methodology Overview
Research Design
Research in beam design often involves a mix of theoretical analysis and practical experimentation. Theoretical frameworks guide the initial design stage, while experiments provide real-world data to validate those designs. As a result, a rigorous approach is taken to ensure that various factors influencing beam performance are carefully evaluated.
Experimental Procedures
Experimental procedures in beam design typically include:
- Load Testing: Physical prototypes are subjected to controlled loading to assess performance and identify points of failure.
- Material Testing: Various materials undergo testing to evaluate their properties, such as tensile strength and stiffness.
- Simulation and Modelling: Computer-aided design (CAD) and finite element analysis (FEA) tools allow engineers to simulate loads and assess performance virtually prior to fabrication.
In summary, the field of beam design is complex and multifaceted. By examining and understanding its principles, materials, and applications, engineers can play a pivotal role in crafting structures that meet the demands of today's fast-evolving environment.
Prolusion to Beams and Their Importance
Beams represent a crucial element in structural engineering and architecture. Their fundamental role is to bear loads and transfer them safely to vertical supports. Understanding beam design is essential for the integrity of buildings, bridges, and various infrastructures. This section aims to explore the significance of beams and how they contribute to overall structure stability.
Beams are everywhere, from residential homes to commercial skyscrapers. They allow structures to span large distances without bending or collapsing under weight. Itโs their ability to handle loads effectively that maintains safety and functionality across diverse applications.
Key Benefits of Beam Design
- Load Distribution: Beams help distribute loads evenly across a framework, preventing localized stress concentrations.
- Structural Efficiency: Well-designed beams utilize materials in an optimal way, which contributes to resource efficiency. This is vital in the modern push for sustainable engineering practices.
- Versatility in Applications: Beams can be implemented in numerous forms, such as beams in bridges, roofs, and floors. Each application requires a unique approach to design, making the foundational principles of beam design indispensable.
- Facilitating Design Innovations: Knowledge of beam principles encourages creative problem-solving in engineering. This aspect can lead to innovative designs that enhance aesthetic and functional qualities in buildings.
Considerations in Beam Design
When designing beams, numerous factors come into play. Engineers must consider the intended use, material properties, the expected load, and environmental factors. Each of these elements significantly influences the design method employed.
- Material Selection: Choice of material is critical. Different materials, such as steel, concrete, or timber, present unique advantages and limitations.
- Types of Loads: Understanding static and dynamic loads ensures that beams are adequately designed to withstand various forces encountered in real-world applications.
- Compliance with Standards: Adherence to international design codes and standards is essential for safety and efficiency. Non-compliance can lead to structural failures.
Beams are indispensable in achieving safe, functional, and sustainable structures. The implications of understanding beam design extend to all fields of engineering, making it important for students, researchers, and professionals alike.
"Effective beam design is not just about strength; it also incorporates aesthetics and environmental considerations."
Gaining insight into beam design opens the door to advanced engineering practices and innovations. By understanding the importance and principles of beams, professionals can contribute to safer and more efficient infrastructure.
Fundamental Principles of Beam Design
Understanding the fundamental principles of beam design is crucial for creating safe, functional, and sustainable structures. Beam design is not just about aesthetics. It involves a careful consideration of material strength, load distribution, and structural stability. The principles guide engineers and architects in selecting the appropriate types of beams, materials, and design methods for different applications. This understanding is essential in ensuring that beams can efficiently support various loads while minimizing material use.
Definition of a Beam
A beam is a structural element that primarily resists loads applied laterally to its axis. It carries loads by bending and is defined by its length, width, height, and cross-section. Beams can be categorized based on their support conditions, material properties, and load characteristics. Understanding what constitutes a beam allows engineers to select the appropriate design and analysis methods tailored to specific requirements. The correct definition of a beam is key, as it influences design decisions from material choice to installation methods.
Structural Behavior of Beams
The structural behavior of beams is influenced by material properties and geometrical configurations. When a load is applied, beams respond through deformation characterized by bending moment and shear force. The relationship between these forces dictates how the beam will perform under various loading conditions. Understanding beam behavior helps engineers predict failure modes, such as buckling or shear failure. This knowledge is crucial during the design phase to ensure structural integrity and safety. A thorough grasp of structural behavior allows for more innovative and effective design solutions.
Types of Forces Acting on Beams
Beams are subjected to a variety of forces, and understanding these forces is vital in the design process. The main types include:
- Point Loads: Localized loads applied at specific points on the beam, causing maximum bending moment at those locations.
- Distributed Loads: Loads spread over a length of the beam, which can create different bending and shear stress distributions compared to point loads.
- Dynamic Loads: Forces that change over time, such as moving vehicles or machinery, which require careful consideration of beam capacity under these conditions.
- Temperature Effects: Changes in temperature can create thermal stresses in beams, requiring consideration, especially for long spans.
Understanding these forces and their interactions with beam geometry is essential in creating reliable and efficient designs. Each type of force impacts the beamโs structural response differently, influencing material choice and design parameters.
Types of Beams
Understanding the different types of beams is critical in the context of structural engineering. Each beam type offers unique characteristics and advantages, influencing the decision-making process during design. Selecting the appropriate beam type can optimize performance, enhance safety, and manage costs effectively. For students, researchers, and professionals alike, grasping these distinctions aids in mastering practical applications, as well as formulating innovative designs suited to specific engineering challenges.
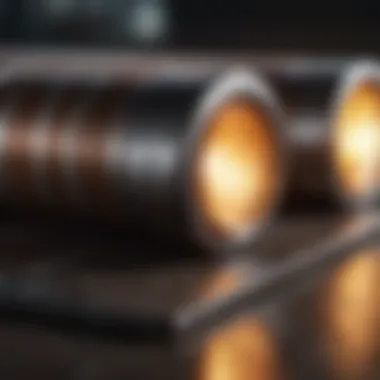
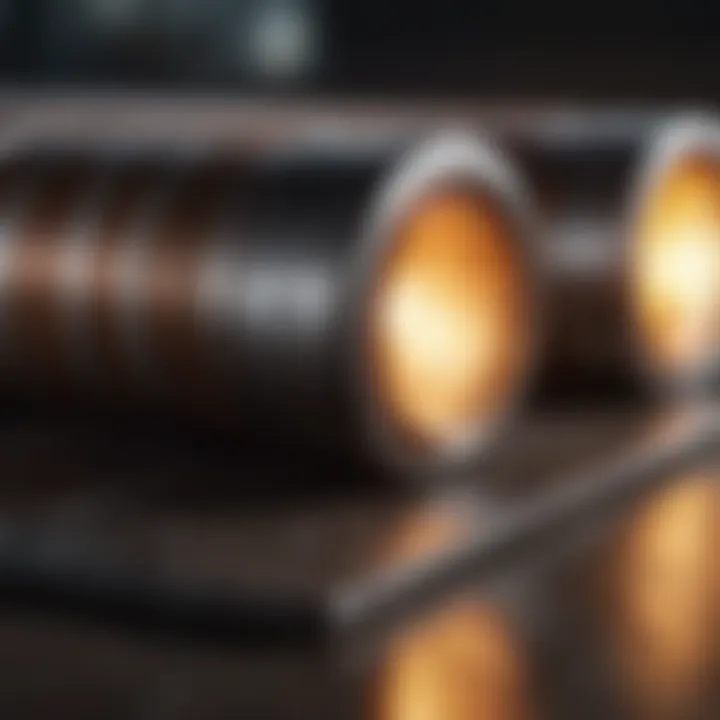
Simply Supported Beams
Simply supported beams are among the most straightforward beam configurations. They are supported at both ends without any restraint against rotation. This design allows the beam to freely deflect under applied loads. One significant benefit of simply supported beams is their simplicity in analysis and construction.
These beams typically experience bending moments, shear forces, and reactions at the supports. An example is a beam spanning between two columns in a building. Such configurations are common in residential structures due to their efficiency and ease of implementation. Generally, engineers utilize various materials for simply supported beams, including steel and reinforced concrete, depending on the project's requirements.
"Simply supported beams represent a foundational element in structural design, often serving as benchmarks for load calculations and performance assessments."
Cantilever Beams
Cantilever beams project outwards from a fixed support, with one end unsupported. This arrangement creates unique loading scenarios, often subjecting the free end to maximum bending moments. The ability to extend without additional supports allows design flexibility, particularly in situations where space is limited.
A common application of cantilever beams can be seen in balconies and bridges. Engineers must carefully calculate the necessary material strength and dimensions to prevent excessive deflection or failure. Materials like reinforced concrete and steel are often chosen based on their load-bearing capacity and durability to ensure safety and longevity in the final design.
Continuous Beams
Continuous beams extend over multiple supports instead of relying on simply supported ends. This design enables the distribution of loads across several points, enhancing stability and performance. Continuous beams show less deflection than simply supported beams, making them advantageous in large-span applications, such as bridges and large industrial buildings.
The analysis of continuous beams is more complex, requiring advanced techniques, such as the use of influence lines and moment distribution methods. These methods help engineers understand how loads affect the overall structure. Continuous beams can often utilize materials like reinforced concrete and steel to manage the increased demands placed on them by the more complex loading conditions.
Composite Beams
Composite beams incorporate two or more materials to maximize the advantages of each. Often combining steel and concrete, these beams utilize the compressive strength of concrete and the tensile strength of steel. This synergy results in a lightweight structure with enhanced load-bearing capacity.
Commonly found in modern construction, composite beams are popular due to their efficiency in high-rise buildings and bridges. Engineers focus on material compatibility and construction detailing to ensure optimal performance and durability. Special attention is also given to bond strength between materials to prevent failure.
Material Considerations in Beam Design
Material selection plays a vital role in beam design. The performance of a structure significantly depends on the materials used. Engineers must consider a variety of factors when choosing the appropriate materials for beams. These factors include strength, durability, weight, and cost. In this section, we will dive into common materials used for beams, their properties and impacts, and sustainability considerations in material choice.
Common Materials Used for Beams
Beam designs are typically realized using several common materials. Each material has specific advantages and is used in different situations. Here are the most frequently used materials:
- Steel: Known for its high tensile strength, steel is often employed in large structures like skyscrapers and bridges. Its ductile nature allows it to withstand considerable loads.
- Concrete: Concrete beams offer excellent compressive strength and durability. They are commonly used in residential and commercial buildings.
- Wood: Typically used for smaller structures or residential settings, wood is lightweight and has good load-bearing capabilities.
- Composite Materials: Combining materials, such as glass fiber reinforced polymer, can optimally tailor properties of beams according to specific applications.
Each of these materials comes with its own set of properties, making it crucial to analyze their performance under various conditions.
Material Properties and Their Impact
Understanding the properties of materials used in beam design is essential. Key properties include:
- Yield Strength: This defines the maximum stress a material can withstand while maintaining its structure. For example, the yield strength of structural steel is significantly higher than that of normal concrete.
- Modulus of Elasticity: This metric helps in understanding how much a material will deform under stress. Materials like steel have a higher modulus compared to wood, which means they are stiffer.
- Thermal Expansion: Materials expand under heat; knowing this helps engineers design beams that can accommodate these changes without cracking.
- Durability: Buildings need materials that withstand environmental conditions. For example, treated wood is used to resist rot.
The impact of these properties on the beam's design and functionality cannot be overstated. Incorrect choices can lead to structural failures.
Sustainability and Material Choice
In recent years, sustainability has become a pressing consideration in beam design. The construction industry is under pressure to utilize materials that have reduced environmental footprints.
- Recycled Materials: Utilizing recycled content in materials can significantly lower the environmental burden. For instance, recycled steel is a popular choice in modern beam design.
- Renewable Resources: Wood from sustainably managed forests can provide a suitable alternative to carbon-heavy products like steel and concrete.
- Lifecycle Assessment: Evaluating the entire lifecycle of the materials can lead to informed decisions that prioritize economic, environmental, and social factors.
Choosing sustainable materials not only helps the environment but can also improve the public perception of the projects and companies involved.
"Materials are the building blocks of our structures. Understanding them is key to effective design and construction."
Loading Conditions and Analysis
Understanding loading conditions and analysis is quintessential in the field of beam design. This section elucidates the various types of loads affecting beams, the significance of accurate load combinations, and the methodologies used to conduct thorough load analysis. Proper analysis ensures that structures can withstand expected forces and loads throughout their lifespan, which ultimately contributes to the safety, durability, and functionality of structures.
Types of Loads Acting on Beams
Loads acting on beams can be categorized mainly into two types: static loads and dynamic loads.
- Static loads are those that are applied slowly and remain constant over time. These include dead loads, which consist of the weight of the structure itself and any permanent fixtures, and live loads that account for temporary loads like people, furniture, and equipment.
- Dynamic loads, on the other hand, vary with time. These can arise from natural forces such as wind and earthquakes or operational forces such as vehicles. Understanding these loads is crucial as they affect structural integrity differently than static loads.
Beam designers often utilize load calculations to determine the appropriate structural elements. Additionally, the distribution of these loads along the length of a beam can significantly influence its behavior. For instance, a uniformly distributed load leads to different reactions compared to a point load.
Load Combinations and Factors
Load combinations are essential to ensure that beams can handle more than one load acting simultaneously, which is more typical in real-life scenarios. The load combination factors are defined by building codes, which govern how different loads should be evaluated together to assess the overall structural performance.
- The factors typically involve combinations of:
- Dead Loads (DL)
- Live Loads (LL)
- Environmental Loads (EL) such as wind and seismic effects
For example, a common load combination might be:
- 1.2 DL + 1.6 LL + 0.5 EL
This factorization addresses various load scenarios, providing a safety margin for unexpected conditions. The use of these factors is mandatory to meet safety and performance standards that are set forth in building codes.
Methods of Load Analysis
Various methods exist for analyzing loads acting on beams, each catering to different complexities of the structure involved. The most common methods include:
- Analytical Methods: Traditional approaches rely on equations of equilibrium to compute reactions and internal forces. Techniques such as the method of sections and the moment distribution method fall into this category.
- Numerical Methods: This approach is beneficial especially when dealing with complex geometries or loading conditions. Finite Element Analysis (FEA) stands out as a prominent technique, dividing the beam into smaller elements for detailed analysis. This allows engineers to predict how beams respond under varying loads accurately.
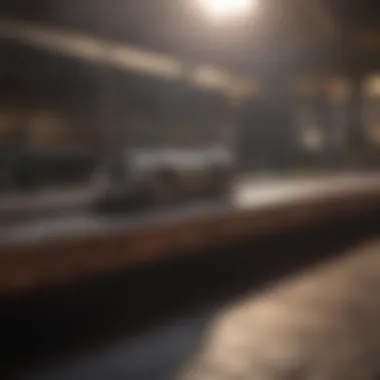
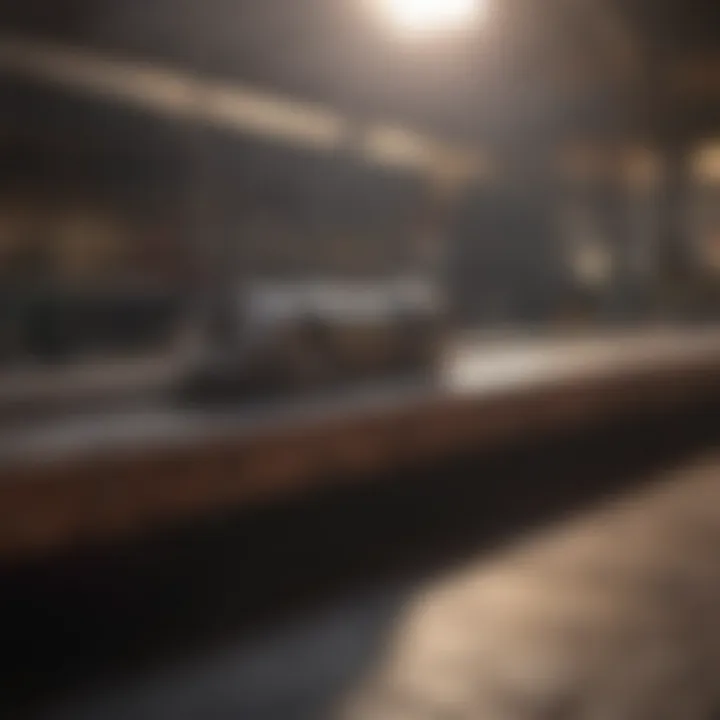
Overall, having a robust analysis of loading conditions is crucial for the design of beams. By understanding not just the types of loads, but how they interact and affect structures, engineers can ensure safe and effective designs.
"Effective load analysis is more than just a checkbox in beam design; it is the foundation on which structural integrity stands."
Ensuring your designs are grounded in empirical data and robust methodologies improves overall engineering outcomes, safeguarding both users and infrastructure.
Design Standards and Codes
Design standards and codes serve as the backbone of any engineering discipline, including beam design. These standards ensure safety, reliability, and performance across structures, which is paramount in today's engineering projects. The importance of understanding design standards cannot be overstated. They not only provide guidelines for material selection and construction methods but also help unify practices across different regions and industries.
Using standardized design codes promotes a common understanding among engineers and architects. It simplifies communication and enables effective collaboration. Furthermore, adherence to these codes fosters public trust in engineered structures. When the public sees that a bridge or building is designed according to recognized standards, confidence in the safety of that structure increases significantly.
In beam design specifically, these standards cover aspects such as load-bearing capacities, deflection limits, and material specifications. Engineers must consider the local regulations and codes, which may vary widely depending on the jurisdiction. Keeping abreast of such codes is essential for compliance and to avoid potential legal repercussions. It requires constant education and awareness of updates in standards to ensure that designs are not just effective but also legally defensible.
"Adhering to established design codes is not just a guideline; it is a commitment to the safety and sustainability of society."
Overview of Design Codes
Design codes, such as those provided by the American Institute of Steel Construction (AISC) or the American Concrete Institute (ACI), outline the requirements for designing structural elements like beams. These codes address various factors, including structural loads, material specifications, and required safety factors. The codes also highlight essential design principles such as load combinations and serviceability requirements.
There are distinct codes for different materials. For instance, when designing with steel, the AISC specification covers design methodologies specific to steel structures. In contrast, when dealing with concrete, the ACI provides detailed guidelines. Understanding the differences between these codes is important for engineers to make informed decisions according to the material they are using.
Additionally, international codes, such as the Eurocode, are becoming increasingly relevant. They offer a more global context and help engineers adopt a standard approach irrespective of geographical boundaries.
Importance of Compliance
Compliance with design standards and codes serves several critical functions within the engineering community. First, it ensures that structures can withstand required loads under given conditions. Compliance helps address performance and safety issues during the lifecycle of a structure.
Not only does compliance safeguard against failures, but it also minimizes the risk of costly legal actions that could arise from code violations. Furthermore, it fosters innovation by encouraging engineers to explore new solutions within the framework of established safety norms.
Moreover, organizations like the National Fire Protection Association (NFPA) or Occupational Safety and Health Administration (OSHA) often incorporate various design codes as essential components of their regulations. Therefore, understanding and following these codes can significantly impact project approvals, construction timelines, and budget management.
In an era where sustainable practices are becoming crucial, adherence to these design codes also aligns with wider environmental goals. Many codes now incorporate sustainability practices, which force engineers to consider energy efficiency and material lifecycle impacts in their designs.
Overall, compliance with design codes is not just a bureaucratic requirement; it is an ethical responsibility that engineers must uphold to ensure the safety and welfare of the public.
Advanced Beam Design Techniques
Advanced beam design techniques are pivotal in modern engineering. As structures grow more complex, the demand for precision and efficiency increases. These techniques streamline the design process, ensuring that beams meet both safety and aesthetic exigences.
Finite Element Analysis for Beam Design
Finite Element Analysis (FEA) is a numerical method that allows for a detailed examination of various phenomena within beams. It provides insight into stress distribution, deflections, and vibrational behavior. FEA divides a structure into smaller, manageable sections, known as elements, which can then be analyzed independently.
The benefits of FEA in beam design include:
- In-depth analysis: Engineers can predict how beams will behave under different conditions, which helps in identifying potential weaknesses.
- Optimization: FEA enables the refinement of the design by testing various materials and configurations without the need for physical prototypes.
- Cost efficiency: By reducing the need for extensive physical testing, FEA saves both time and resources.
Using FEA can result in the creation of more efficient beams, ultimately leading to safer and more sustainable structures.
Optimization Techniques in Beam Design
Optimization in beam design refers to the process of making a beam as effective and efficient as possible while satisfying all constraints, including material limits and load requirements. Various techniques can be used to achieve this, including:
- Topology Optimization: This method determines the best material layout within a given design space, subject to various constraints. It aims to maximize performance while minimizing weight.
- Shape Optimization: By altering the profile of a beam, engineers can enhance its performance characteristics, reducing stress concentrations and improving load-bearing capacities.
- Multi-objective Optimization: This technique balances competing design goals, such as weight reduction versus cost or strength versus flexibility.
Effective optimization techniques lead to beams that not only perform better but also use materials more sustainably, aligning with modern engineering principles aimed at reducing environmental impact.
To sum up, advanced beam design techniques such as FEA and optimization are critical to the future of structural engineering. They enhance the safety, efficiency, and sustainability of beam designs.
Case Studies in Beam Design
Case studies in beam design provide a practical perspective on the application of theoretical principles within real-world contexts. They serve as essential tools for engineers and architects as they illustrate successful implementations and innovative solutions in various structural challenges. Not only do these case studies highlight effective designs, but they also expose potential pitfalls and the ways these can be navigated.
Understanding past projects fosters a deeper insight into what works effectively. Case studies also demonstrate the integration of new technologies and materials in beam design, showcasing the adaptability of professionals in this field. Additionally, they promote the sharing of knowledge, allowing future designers to build upon previous successes and mistakes.
"Learning from others' experiences in beam design can significantly enhance project outcomes and reduce the risk of failure."
Successful Beam Designs in Architecture
Successful beam designs in architecture often showcase a marriage of aesthetics and functionality. Iconic structures like the Sydney Opera House or the Centre Pompidou in Paris exhibit not only engineering marvels but also artistic vision. For instance, the design of the roof structure of the Sydney Opera House employs pre-cast concrete shells that efficiently distribute loads while also creating a distinctive visual profile. This not only meets the structural requirements but also enhances the building's iconic status.
In many cases, the choice of material plays a critical role. Steel is frequently used in high-rise buildings due to its high strength-to-weight ratio, allowing for longer spans and open spaces without obstructing views. Additionally, innovations such as ultra-high performance concrete provide enhanced durability, reducing the need for maintenance.
Successful designs evoke emotions and create functional spaces, while adhering to strict structural design principles.
Innovative Solutions in Engineering Challenges
Innovative solutions in beam design arise when faced with complex engineering challenges. One notable example is the use of composite materials in the construction of bridges. In the case of the San Francisco-Oakland Bay Bridge, engineers encountered the need for both flexibility and strength to withstand seismic activities. The incorporation of fiberglass reinforced polymer beams has allowed for lighter structures that can endure stress without sacrificing performance.
Additionally, the introduction of digital tools and finite element analysis has revolutionized beam design approaches. Engineers can now simulate structural behaviors under various load conditions before actual construction begins. This reduces risks while optimizing material usage.
The ability to adapt and innovate in response to challenges is a hallmark of effective beam design. Examples abound where creative thinking has led to more efficient, sustainable, and aesthetically pleasing solutions in architecture and engineering.
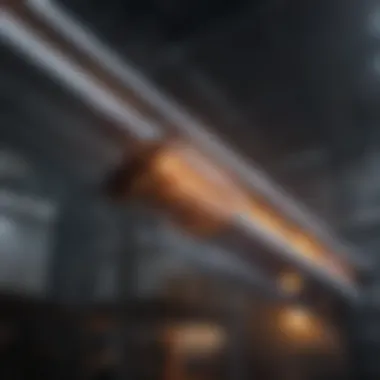
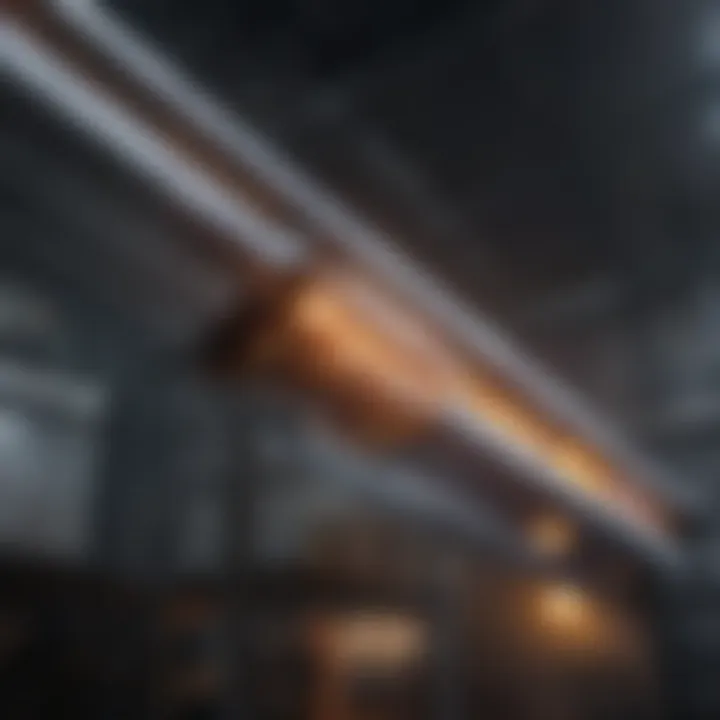
Emerging Technologies in Beam Design
The landscape of beam design has witnessed substantial evolution due to the advancement of emerging technologies. This section elucidates the significance of these technologies within the context of structural engineering and how they fundamentally reshape design methodologies. Understanding these innovations is essential for educators, students, and industry professionals alike.
Role of Computational Tools
Computational tools play a critical role in modern beam design. Software such as AutoCAD, Revit, and ANSYS provides engineers with the ability to perform complex calculations efficiently. These tools enable designers to create detailed models that simulate the behavior of beams under various conditions. As a result, engineers can evaluate different design alternatives through simulations before committing to any physical construction.
Additionally, computational capabilities facilitate faster decision-making processes. Designers can assess parameters such as load distributions, material stress, and environmental factors with precision. This level of analysis not only improves the quality of designs but also ensures safety and compliance with industry standards.
Benefits of Computational Tools
- Speed: Rapid design iterations lead to improved productivity.
- Accuracy: Minimized human errors through automated calculations.
- Simulation: Ability to test real-world scenarios within a virtual environment.
Adoption of these computational tools is paramount in achieving innovative designs that push the boundaries of what is possible in beam construction and structural analysis.
Impact of Smart Materials
Smart materials represent a game-changing trend in beam design. These materials respond dynamically to changes in their environment, offering potential benefits that traditional materials cannot match. For instance, materials like shape memory alloys and piezoelectric materials have the ability to alter their properties in response to stress or temperature variations, effectively enhancing the performance of beams under varying loads.
The integration of smart materials can lead to more resilient structures capable of adapting to unforeseen circumstances, such as seismic activities or extreme weather conditions. Furthermore, they contribute to energy efficiency goals, as some smart materials can harness energy from their environment.
Considerations for Smart Materials
- Cost: While the performance benefits are significant, the initial cost can be higher than traditional materials.
- Complexity: Understanding the properties and behavior of smart materials requires specialized knowledge.
- Lifecycle: Evaluation of long-term durability and maintenance as a function of material properties is crucial.
"The future of beam design will likely hinge on our ability to harness both computational tools and smart materials, leading to safer and more efficient structures."
Future Trends in Beam Design
The field of beam design is undergoing significant transformations due to technological advancements and increased emphasis on sustainability. Understanding the future trends is crucial not only for engineers but also for architects and project managers who are involved in designing safe and efficient structures. As construction methods continue to evolve, integrating these trends can lead to improved performance, reduced costs, and environmental benefits.
Integration of AI in Design Processes
Artificial Intelligence (AI) is emerging as a powerful tool in beam design. The integration of AI can enhance various aspects of the design process. For instance, AI algorithms can analyze large datasets, evaluating different configurations and materials much faster than traditional methods. This can help in optimizing beam shapes and sizes based on specific loads and environmental conditions.
Benefits of AI Integration:
- Efficiency: Reduces the time spent on design iterations.
- Error Reduction: AI systems can identify potential design flaws early, decreasing the risk of costly changes later.
- Customization: Facilitates the design of unique structures tailored to specific requirements.
- Predictive Analysis: AI can predict how a beam will perform under various conditions, enhancing safety.
The use of AI supports engineers in making data-driven decisions. Consequently, project timelines become shorter and cost overruns can be minimized.
Sustainability Considerations for the Future
Sustainability is a growing concern in beam design. As the world grapples with climate change, the construction industry must seek methods that reduce environmental impact. The future of beam design will heavily focus on selecting materials and methods that align with sustainable practices.
Some important considerations include:
- Material Selection: The trend is shifting towards using recycled or renewable materials, such as bamboo or engineered wood, reducing carbon footprints.
- Energy Efficiency: Designs that enhance energy efficiency in buildings can also apply to beam construction. This includes ensuring proper insulation and using materials that reduce heat loss.
- Life Cycle Assessment: Future designs will involve more comprehensive assessments of the environmental impact of beams from production to demolition.
- Circular Economy: Emphasizing the reuse and recycling of materials contributes to less waste in construction sites.
Sustainable design is not just an option; it is becoming a necessity to adapt to the challenges of climate change.
By understanding and adopting these future trends in beam design, the construction industry can create structures that are not only safe and functional but also environmentally responsible.
Challenges in Beam Design
Beam design embodies a core facet of structural engineering, requiring a careful melding of theory, practicality, and creativity. The importance of this topic in the broader discussion of beams cannot be overstated. Understanding the challenges involved in beam design is pivotal for ensuring that structures are safe, efficient, and resilient.
The challenges in beam design may involve several specific elements such as the identification of risks, balancing costs, and ensuring performance. These factors not only affect the immediate construction process but influence long-term sustainability and reliability of structures. Each project necessitates a tailored approach to effectively navigate these challenges, making expertise in this area crucial for students, researchers, educators, and engineering professionals alike.
Identifying and Mitigating Risks
In beam design, identifying risks is essential to effective planning. Risks can range from material failures, structural instability, to unexpected loads. Engineers must perform thorough analyses during the design phase, including risk assessments that consider environmental impacts, intended use, and potential loading scenarios. Assessing these factors allows for the design of beams capable of handling expected stresses and strains. Specific methods to mitigate risks include:
- Conducting sensitivity analyses to understand which variables have the greatest impact on performance.
- Utilizing advanced modeling tools to simulate real-world conditions and address possible failure points preemptively.
- Incorporating redundancy into beam designs, so even if one component fails, the overall structure remains intact.
Ultimately, a proactive stance on risk management enables engineers to deliver safe and reliable structures while minimizing the chances of costly modifications or failures during and after construction.
Balancing Cost and Performance
A crucial aspect of beam design involves balancing cost with performance. On one hand, reduced costs can lead to the selection of inferior materials or designs that may compromise structural integrity. On the other hand, prioritizing performance can escalate overall project expenses, potentially rendering the project unfeasible.
Key considerations when tackling this balance include:
- Material Selection: Choosing materials like steel, concrete, or advanced composites significantly influences both cost and performance outcomes.
- Design Efficiency: Employing optimized shapes and sizes for beams can reduce material usage while maintaining performance, ultimately enhancing cost-efficiency.
- Lifecycle Costs: Assessing the long-term benefits of a given design can aid in making informed choices that may cost more initially, but pay off over time in reduced maintenance and repairs.
By understanding the intricate relationship between cost and performance, engineers can make informed decisions that fulfill both budgetary constraints and performance expectations.
"Successful beam design is not solely about material and load; it is a sophisticated balance of multiple factors that ensures the viability of a structure over its intended lifespan."
In summary, the challenges in beam design require nuanced understanding and strategic planning. Identifying and mitigating risks alongside balancing cost and performance are cornerstones of effective beam design. This knowledge holds immense value for professionals within the field, informing better design practices and ultimately leading to safer, more sustainable structures.
Closure
The conclusion of this article captures the essence of beam design, reflecting on its foundational principles and practical applications. Understanding the intricacies involved in beam design is vital for engineering students, researchers, and professionals. It is more than just a technical process; it affects safety, functionality, and resource efficiency in real-world applications.
Through this comprehensive examination, we have highlighted several key elements that encompass the concept of beam design. These include the significance of correct material selection, which can lead to stronger and more durable structures, and the importance of adhering to design standards and codes, facilitating compliance and safety in construction projects.
Moreover, we have explored current challenges faced by engineers, including balancing cost and performance. This informs the reader about the complexities in achieving sustainable and efficient design outcomes in various structural applications.
The emerging technologies mentioned demonstrate how industry practices are evolving in response to new challenges and opportunities. Tools like finite element analysis and smart materials pave the way for innovative solutions in beam design, allowing for more refined and robust structures.