Biochips in Humans: Implications and Future Prospects
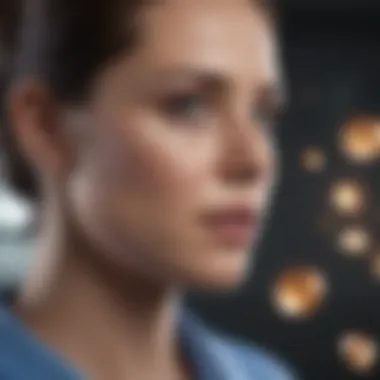
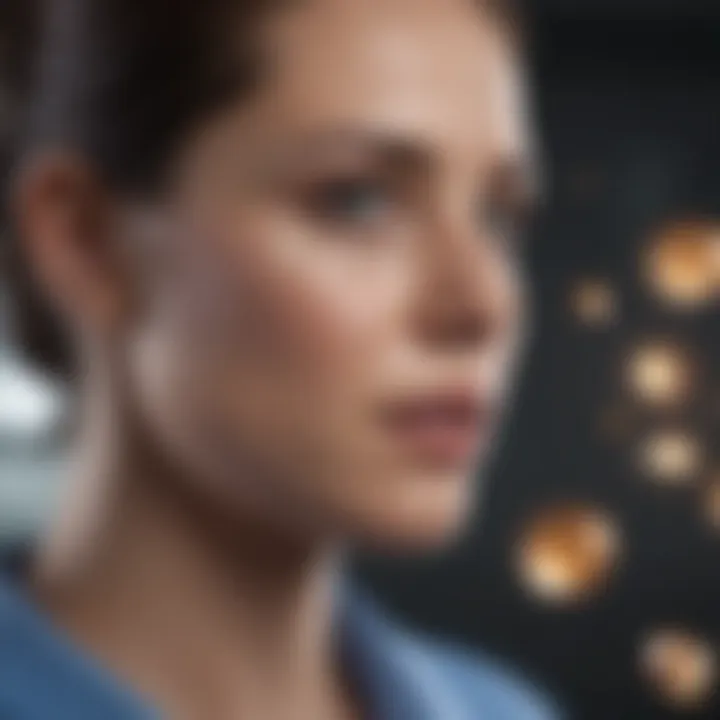
Intro
Biochips represent a significant advancement in the intersection of technology and medicine. These small devices, often made up of biocompatible materials, have the potential to transform healthcare through their ability to monitor biological processes in real time. Biochips can facilitate early diagnosis, personalized treatments, and even advancements in biotechnology. As this field develops, it is imperative to explore the design, functionality, and implications of biochips integrated into human bodies.
Research Highlights
Key Findings
Recent studies show the versatility of biochips. They can be used for various applications, such as disease detection, biomarker identification, and even drug delivery systems. Their ability to analyze vast amounts of biological data quickly is noteworthy. Furthermore, biochips can be engineered for specific tasks, enhancing their effectiveness in personalized medicine.
- Disease Detection: Detection of various diseases, including cancer, through early screening methods.
- Personalized Treatments: Tailoring medical treatments to individual genetic profiles.
- Real-Time Monitoring: Continuous assessment of health indicators, leading to proactive health management.
Implications and Applications
The implications of biochips are extensive. In the realm of healthcare, biochips can lead to significant cost reductions by minimizing the need for invasive procedures and streamlining diagnostics. This innovation can empower patients and healthcare professionals with data-driven insights, which may improve overall health outcomes.
- Patient Empowerment: Patients can access their health data and make informed decisions.
- Efficiency in Healthcare: Reducing time and resources spent on traditional diagnostic methods.
- Research Advancements: Expanding knowledge in human biology and chronic diseases.
Methodology Overview
Research Design
The exploration of biochips typically involves both qualitative and quantitative research methods. Researchers analyze the effectiveness of biochips through clinical trials and case studies. This mixed-methods approach provides a comprehensive view of their impact on patient care.
Experimental Procedures
When conducting experiments involving biochips, researchers often focus on their biocompatibility, sensitivity, and accuracy. Common procedures include:
- Prototype Development: Creating initial versions of biochips.
- Testing in vitro: Assessing functionality in a controlled environment.
- Clinical Trials: Evaluating safety and efficacy in human subjects.
Biochip technology continues to grow. Its integration into healthcare holds immense potential. Addressing ethical considerations surrounding its use is equally vital. As such, biochips may redefine how we approach healthcare and biotechnology in the future.
Intro to Biochips
Biochips are pivotal tools that facilitate a deeper understanding of human biology and its complexities. Their design and functionality represent a significant breakthrough in technology, impacting several areas, particularly healthcare and biotechnology. The ability to analyze biological materials with high precision opens avenues for advanced diagnostics and personalized medical interventions.
The integration of biochips into human biology transcends traditional methods of testing and treatment. They enable rapid analysis of genetic material and proteins, leading to early disease detection and tailored treatment plans. Consequently, biochips hold the potential to drastically improve patient care and outcomes.
This section will present a foundational understanding of biochips, starting with a clear definition and overview. Furthermore, the historical context highlights their evolution and the technological advancements that have positioned them as essential components in contemporary biomedicine.
Definition and Overview
Biochips can be defined as miniature devices that embed biological molecules and enable simultaneous analysis of multiple biological samples. They contain an array of sensors that detect and analyze various substances like DNA, RNA, proteins, or cells, often in very small quantities. The most commonly known type of biochips is DNA microarrays, which allow researchers to study gene expression and genetic variations effectively.
These chips utilize different biochemical techniques, integrating numerous complex processes into a single platform. The ability to execute numerous tests on a single chip is both time-efficient and cost-effective, making biochips valuable in research and clinical settings. As technology evolves, the prospects for biochip applications continue to expand, integrating features such as portability and real-time data processing.
Historical Context
The concept of biochips emerged from the convergence of molecular biology and technology. Initially, the development of DNA sequencing in the 1970s laid the groundwork for biochip technology. Over the decades, researchers introduced various forms of microarrays, with the first DNA microarray reported by Pat Brown and his team in the mid-1990s. This invention heralded a new era where biological research could harness computational capabilities to analyze data on a massive scale.
In the early 2000s, advancements in fabrication techniques allowed for the creation of more sophisticated biochips. This included the incorporation of proteins and other biomolecules onto chips, broadening their applications to proteomics and diagnostics. The historical trajectory of biochips reflects an ongoing interplay between biological inquiry and technological innovation, marking them as a cornerstone in the quest to decipher human biology.
"The integration of biochips signifies not just a scientific advancement but a reinterpretation of possible interactions within biological systems."
As the field continues to evolve, it is imperative to understand both the technological milestones that led to current capabilities and their potential to reshape future biomedical practices.
Types of Biochips
Biochips are becoming increasingly important in the field of biotechnology and medicine. Their ability to analyze biological samples rapidly and accurately makes them crucial tools in research and healthcare. This section will discuss the primary types of biochips, namely DNA microarrays, protein microarrays, and lab-on-a-chip technology, each offering unique benefits and functionalities.
DNA Microarrays
DNA microarrays are a powerful tool for genetic analysis. They can simultaneously measure the expression levels of thousands of genes within a single experiment. This capability is vital for understanding complex biological processes, such as disease progression and response to treatments. In cancer research, for instance, DNA microarrays help in identifying gene expression patterns associated with specific types of tumors.
One of the main advantages of DNA microarrays is their high throughput, allowing researchers to process large amounts of data quickly. Moreover, they can facilitate personalized medicine by enabling genetic testing that identifies individual risks and responses to medications. This technology can lead to tailored treatment plans, improving patient outcomes immensely.
Protein Microarrays
Protein microarrays extend the functionalities of DNA microarrays, focusing on the expression and interaction of proteins. These biochips enable the analysis of protein-protein interactions, post-translational modifications, and the identification of biomarkers for diseases. This information is crucial to understand cellular mechanisms and develop new therapeutic strategies.
The significance of protein microarrays lies in their ability to capture the complexity of biological systems better than gene-based approaches. By profiling proteins, researchers can identify disease biomarkers and examine the effects of drugs more effectively. For example, in autoimmune diseases, protein microarrays can distinguish between different types of proteins that may trigger adverse immune responses, informing better treatment options.
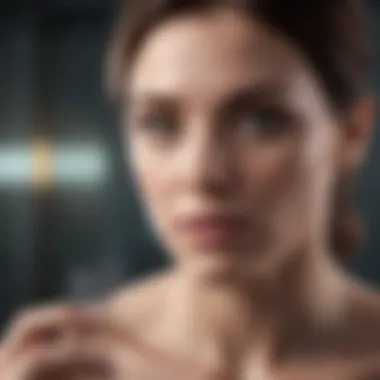
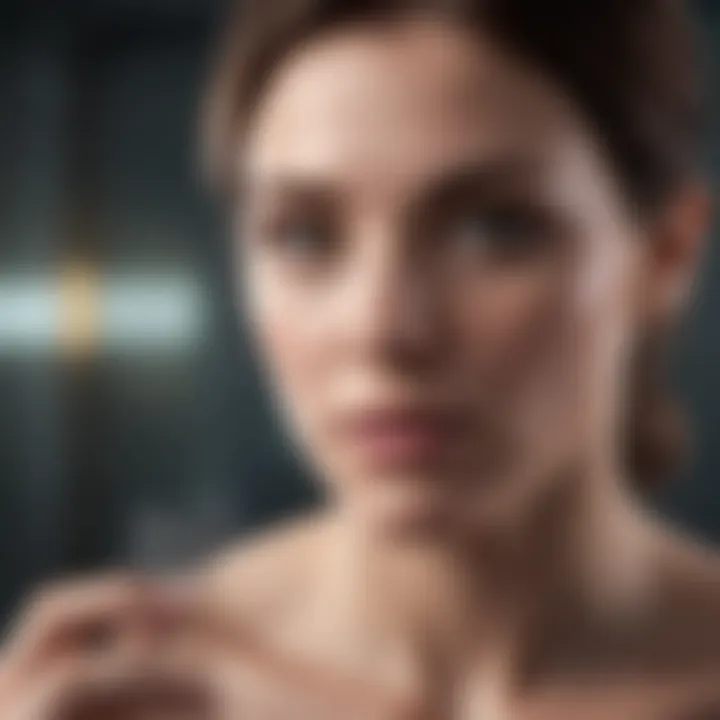
Lab-on-a-Chip Technology
Lab-on-a-chip technology integrates multiple laboratory functions onto a single chip the size of a credit card. This innovation allows for the miniaturization of processes such as sample handling, reaction, and detection within one system. The importance of lab-on-a-chip devices is their potential for point-of-care diagnostics, which can be performed outside traditional lab settings. This capability is especially valuable in remote locations or during emergencies.
Lab-on-a-chip devices offer rapid results, low sample volumes, and reduced costs compared to conventional laboratory methods. This technology can revolutionize the way clinical diagnostics are performed, making it possible to obtain results in a matter of minutes. As a result, the integration of lab-on-a-chip technology into healthcare systems can enhance patient care by enabling timely interventions.
"The future of diagnostics lies in the ability to conduct comprehensive analyses at the point of care, bringing testing directly to patients instead of waiting for laboratory results."
Biochip Design and Fabrication
Biochip design and fabrication are critical components in the effective application of biochips in human health and biotechnology. These processes not only affect the functionality of the biochips but also determine their performance in various medical applications such as diagnostics, personalized medicine, and disease monitoring. Understanding the nuances of design and fabrication allows for the development of biochips that can interact with biological systems effectively, ensuring accurate results and facilitating better healthcare outcomes.
Materials Used
In the realm of biochip fabrication, the choice of materials is paramount. Commonly used materials include polymers like poly(dimethylsiloxane) (PDMS), glass, and silicon. Each material has distinct properties which affect the biochip's performance. For example, PDMS is favored for its flexibility, biocompatibility, and ease of manufacturing. Glass provides high optical clarity essential for imaging and can also be modified to create specific surface chemistries.
The surface chemistry of the materials is significant. Modification techniques might include silanization or the attachment of biomolecules, allowing for better selective binding of target analytes. This leads to enhanced sensitivity and specificity in detection methods. Additionally, the choice of materials influences the overall cost and scalability of biochip production, which are crucial factors for widespread clinical application.
Manufacturing Techniques
The manufacturing techniques employed in biochip creation also play a pivotal role in their functionality. Standard techniques include photolithography, microcontact printing, and inkjet printing.
Photolithography, a traditional method in microelectronics, creates precisely defined patterns on a substrate. This allows for intricate designs essential for high-resolution biochips.
Microcontact printing is a newer technique that involves using an elastomeric stamp to transfer molecules onto a surface. This method enables rapid production and can be scaled up effectively.
Inkjet printing, while more recent, is notable for its ability to quickly print biomolecules, opening up new possibilities for customization. By adjusting the parameters of the inkjet process, different patterns and concentrations can be achieved, catering to specific assay needs.
Mechanism of Action
Understanding the mechanism of action of biochips is essential to unlock their potential in various fields, especially in medicine and biology. The way biochips interact with biological systems and process information illustrates their relevance in real-world applications. Biochips work by harnessing biochemical reactions to detect specific biomolecules, enabling efficient data collection and analysis.
One significant aspect of this mechanism is signal detection. Signal detection serves as the foundation for the biochip's capability to identify and quantify biological materials. Sensors within biochips recognize specific targets, such as DNA or proteins, by binding to them. This binding interaction generates a signal, which is often measured in various ways. The strength of the signal correlates with the presence and quantity of the target molecule. Improved precision in signal detection enhances the reliability of diagnostics and disease monitoring.
A robust signal detection system not only improves sensitivity but also specificity. Distinguishing between similar biomolecules is crucial, especially in applications such as genetic testing. The effectiveness of signal detection hinges on the design of the biochip and the materials used in its construction. Enhanced materials and fabrication methods contribute to better performance in terms of lower detection limits and improved response times.
The mechanism of action plays a pivotal role in determining the usability and effectiveness of biochips in practical applications.
Signal Detection
Signal detection in biochips encompasses various techniques aimed at identifying biochemical interactions. One common approach is fluorescence-based detection. This method relies on fluorescent tags attached to biomolecules, emitting light upon excitation. The emitted light is measured to assess the target's concentration. This approach benefits from high sensitivity, making it valuable for low-abundance biomolecules.
Another technique involves electrochemical detection, where changes in electrical signals are used to infer biomolecule concentrations. This method can be highly effective due to real-time measurement capabilities and lower costs compared to optical methods.
In certain cases, surface plasmon resonance (SPR) can be utilized to monitor biomolecular interactions in real-time. SPR detects changes in the refractive index near the sensor surface as molecules bind, offering a label-free method that can provide quantitative results without requiring additional labels.
Data Analysis Techniques
Once signals are detected, data analysis techniques play a vital role in interpreting the results. Biochips generate vast amounts of data, requiring robust analytical methods to extract meaningful information. Algorithms and biosystems leverage machine learning and artificial intelligence to analyze complex datasets effectively.
Common techniques include statistical analysis and pattern recognition. Statistical methods help to determine the significance of the results, often guiding decisions in clinical diagnostics. Pattern recognition identifies specific traits in data, facilitating early disease detection or monitoring treatment responses.
Moreover, software tools are essential to visualize the data. Visualization aids in contextualizing results, making it easier for researchers and clinicians to draw conclusions based on the evidence presented. Seamless integration of data analysis enables biochips to transition from research tools to standard practices in clinical settings.
Applications in Medicine
The integration of biochips in medicine is vital for enhancing healthcare delivery. These tiny devices enable precise diagnostics and treatments that are tailored to individual needs. Their importance also lies in real-time monitoring, which allows for rapid responses to changing health conditions. The benefits of biochips in medicine go beyond efficiency; they ultimately hold the promise of improving outcomes for patients.
Diagnostics
Early Disease Detection
Early disease detection is a crucial aspect of diagnostics. Biochips allow for the identification of diseases at their onset, before severe symptoms manifest. This proactive approach is increasingly recognized as a key characteristic of modern healthcare solutions. The capability to detect subtle changes in biomarkers enables healthcare providers to intervene sooner.
The unique feature of early disease detection through biochips is the ability to analyze multiple markers simultaneously in a single test. This characteristic not only streamlines the diagnostic process but also enhances accuracy. One significant advantage is the potential for preventive care. With early information, patients can adopt lifestyle changes or receive early treatment, effectively slowing disease progression.
However, one must consider the disadvantages, such as potential false positives, which might lead to unnecessary anxiety or treatment. Thus, while promising, cautious interpretation of results is essential.
Genetic Testing
Genetic testing represents another fundamental application of biochips in diagnostics. It allows for the understanding of inherited diseases and predispositions. The key characteristic of genetic testing is its ability to provide insights into a person’s genetic makeup, identifying mutations or anomalies. This feature is particularly beneficial in the context of hereditary conditions, paving the way for informed decision-making in health matters.
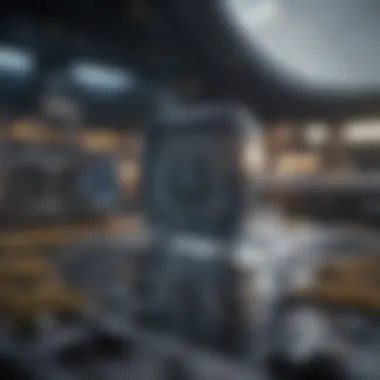
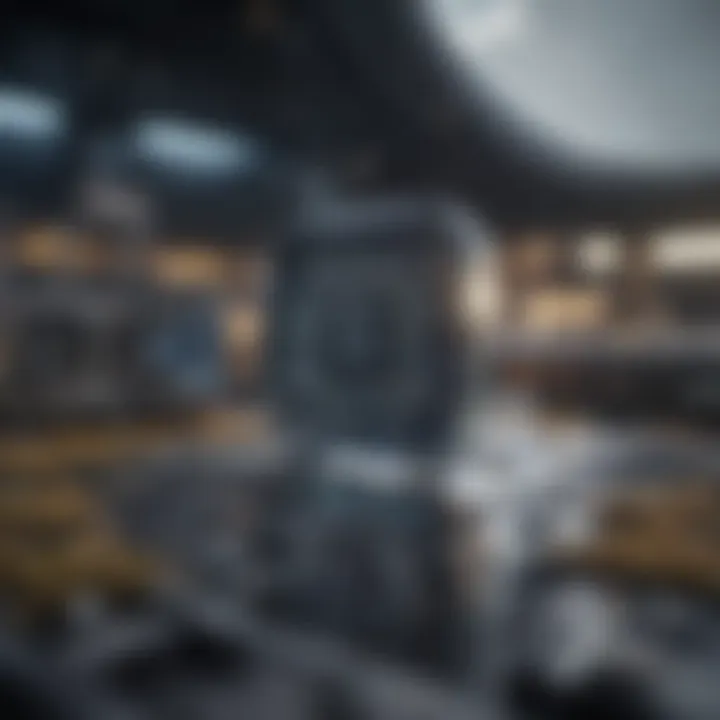
Genetic testing's unique advantage lies in its precision; it directs medical professionals towards targeted therapies that align with an individual’s genetic profile. By personalizing approaches to care, it stands out as a powerful tool for both prevention and treatment.
On the downside, ethical concerns can arise, such as issues regarding privacy and the potential for discrimination based on genetic information. Managing these concerns is crucial to maintaining trust in genetic testing and its applications.
Personalized Medicine
Tailored Treatment Plans
Tailored treatment plans are a defining element of personalized medicine that leverage the capabilities of biochips. These plans consider individual variances, including genetic factors, lifestyle, and environmental influences, to optimize therapy. The importance of this approach lies in its ability to enhance treatment efficacy while minimizing adverse effects.
A vital characterisic of tailored treatment plans is their responsiveness to individual needs. By using biochips that enable detailed patient profiling, healthcare practitioners can design interventions that directly address the unique aspects of each patient’s condition.
Moreover, the unique feature of continuous data acquisition from biochips facilitates adjustments to treatment over time. This adaptability is a significant advantage, particularly for chronic conditions that demand ongoing management.
Nonetheless, the costs associated with implementing highly personalized treatment plans could be prohibitive. Hence, accessibility remains a challenge that must be addressed for widespread adoption.
Pharmacogenomics
Pharmacogenomics is the study of how genes affect a person's response to drugs. Biochips play a pivotal role in this field by enabling the analysis of genetic variations that influence drug metabolism. The key characteristic of pharmacogenomics is its capacity to inform prescribing decisions based on genetic information.
This application is beneficial because it aims to eliminate the trial-and-error approach often seen with medication. Instead, it allows healthcare providers to select the most suitable drugs and dosages for patients.
The unique benefit of pharmacogenomics is its potential to improve safety and efficacy outcomes, thereby reducing adverse drug reactions. However, similar to genetic testing, issues of privacy and the need for informed consent are paramount considerations.
Monitoring Disease Progression
Monitoring disease progression is another critical application of biochips in medicine. These devices facilitate continuous observation of biomarkers associated with specific diseases. The ongoing tracking of these markers provides vital information about how a disease is evolving over time.
Such monitoring enables healthcare professionals to assess the effectiveness of treatments, make necessary adjustments, and predict potential complications. The real-time data provided by biochips can lead to timely interventions, possibly improving patient outcomes.
Yet, there are challenges, including data overload and the potential for misinterpreting signals. As with other applications, the integration of biochips into routine clinical practice requires careful management of data to maximize benefits while mitigating risks.
Ethical and Social Considerations
The discussion around biochips encompasses not only their scientific and technological implications but also their ethical and social dimensions. As the integration of biochips in humans becomes more prevalent, understanding these dimensions is crucial. The technology poses various benefits, yet it also raises significant ethical challenges. This section delves into privacy concerns, informed consent, and equity in access, all critical to shaping a responsible approach to biochip implementation.
Privacy Concerns
Biochips have the potential to store vast amounts of personal data, including genetic information and health records. This capability raises serious privacy concerns. If data is not adequately protected, there is a risk of unauthorized access. Hackers or malicious actors could exploit sensitive information, potentially leading to identity theft or discrimination. Furthermore, there is the possibility of data misuse by governments or corporations, creating a surveillance landscape where personal freedoms are compromised.
"The ethical implications of biochip technology require vigilant oversight and strong data protection measures."
Hence, establishing stringent regulations on data usage is essential. Regulatory bodies must create clear guidelines surrounding data ownership, sharing, and storage to safeguard individuals' privacy. Individuals equipped with biochips should have the right to control how their information is used and shared.
Informed Consent
Informed consent stands at the forefront of ethical biochip integration. The concept implies that individuals should fully understand the implications of having a biochip implanted. This includes comprehension of potential risks, benefits, and the nature of the data being collected.
Many individuals may not possess the technical knowledge required to understand complex biochip functionalities fully. Therefore, it is crucial that healthcare providers present information in a manner that is accessible to diverse audiences. Prior to any implantation, a patient should be equipped with information to make an educated choice. This is particularly important given the sensitivity of genetic and health-related data.
To ensure informed consent, healthcare professionals may need to implement standardized education protocols that clearly outline what a biochip entails and the implications of its usage. Such resources should be developed in partnership with bioethicists to maintain high ethical standards.
Equity in Access
The introduction of biochip technology raises questions about equity in access. As with many technological advancements in healthcare, biochips may not be uniformly accessible to all populations. Socioeconomic status can greatly influence whether or not an individual has access to this technology.
Issues related to health disparities may exacerbate divisions among different demographics. Wealthier individuals may gain access to cutting-edge biochip technology, while marginalized communities may be left behind. This disparity can further entrench existing societal inequities.
Efforts must be made to ensure that biochip technology is accessible, including reducing costs and providing education about the benefits it presents. Policymakers should advocate for inclusive initiatives, ensuring that advancements in biochips contribute to health equality.
In summary, ethical and social considerations play an integral role in the discussion surrounding biochips. Addressing privacy concerns, ensuring informed consent, and increasing equity in access are essential steps to navigate the landscape of biochip technology responsibly.
Regulatory Landscape
Understanding the regulatory landscape surrounding biochips is critical for their adoption in clinical settings. Regulations govern not only the safety and efficacy of biochips but also their ethical use within healthcare. This regulatory framework ensures that innovations in biochip technology align with medical standards and serve public health interests. Additionally, various stakeholders, including manufacturers, healthcare providers, and patients, are influenced by these regulations, making it a multidimensional aspect of biochip integration in human applications.
Current Regulations
Presently, biochips are subject to a range of regulations that differ by region. For instance, in the United States, the Food and Drug Administration (FDA) plays a pivotal role in the approval and oversight of biochip technologies. The FDA requires thorough validation processes before a biochip can be marketed. These processes include premarket notification under 510(k) or approval via a premarket approval application (PMA). The objective is to confirm that biochips meet the necessary standards for safety and effectiveness, particularly since they can influence clinical decisions.
In the European Union, biochips are regulated under the In Vitro Diagnostic Regulation (IVDR). This regulation categorizes biochips based on risk, requiring varying levels of assessment based on their intended use. Both regulatory frameworks are designed to establish a balance between fostering innovation while protecting and ensuring patient safety.
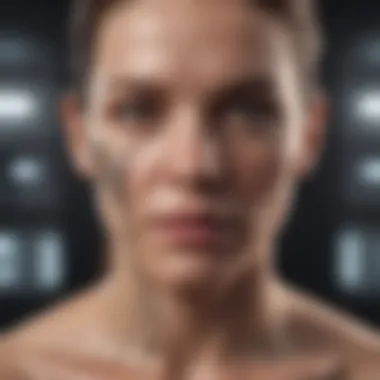
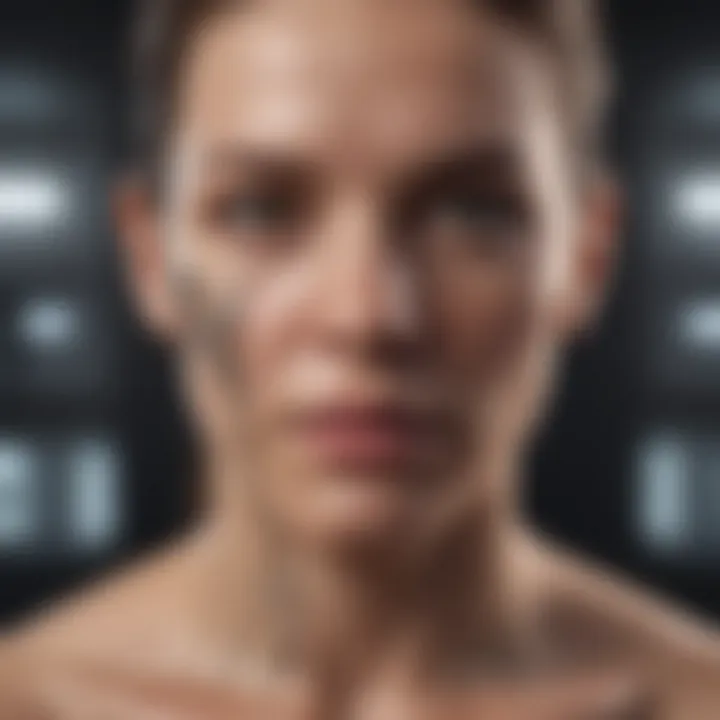
"Ensuring that biochips are subject to strict regulatory compliance is essential for maintaining public trust in their applications within healthcare."
Future Regulatory Challenges
As the biochip industry continues to evolve, future regulatory challenges are anticipated. One significant concern is the growing complexity of biochip technologies, including their integration with artificial intelligence and machine learning for data analysis. This increased sophistication raises questions about the adequacy of existing regulations to keep pace with rapid advancements.
Another challenge involves global standardization. There is inconsistency in regulations across countries. This hampers international collaboration and market access for biochip manufacturers. Harmonizing regulations could simplify compliance for companies operating in multiple jurisdictions, facilitating more effective deployment of biochip technologies.
Lastly, the implications of personalized medicine will also require regulatory evolution. Biochips that provide tailored treatment options may demand new regulatory pathways to ensure they are not only effective but also equitable in accessibility across diverse populations. Addressing these challenges will be necessary for the responsible development of biochips in healthcare.
Challenges and Limitations
Understanding the challenges and limitations associated with biochips is critical to grasping their role in human health and biotechnology. These devices hold great promise but also face significant technological and economic barriers. Identifying these issues can refine future research and application strategies.
Technical Limitations
One core aspect of challenges faced by biochips is the technical limitations inherent in their design and function. Despite ongoing advancements, many biochips exhibit precision and sensitivity constraints. For instance, the accuracy of reading biological signals can vary significantly among biochip types. Factors such as fluid dynamics, surface coating, and sensor degradation can all impact the reliability of results.
Another issue is the capacity of current biochips to analyze multiple biomarkers simultaneously. While advancements in multiplexing technologies are notable, the practical application can often fall short due to complications in data interpretation and potential signal interference. This not only complicates diagnostics but can also lead to misdiagnosis or delayed treatment.
"Technical limitations in biochips hinder their adoption in routine clinical practice, necessitating continued innovation and improvement."
Moreover, the standardization of biochip processes has not yet been universally achieved. Variabilities in manufacturing techniques and protocols can cause inconsistencies in results across different platforms, making comparisons challenging. This lack of standardization can pose hurdles in regulatory approvals as well. Thus, more robust frameworks for biochip evaluations are needed.
Cost-Effectiveness
The cost-effectiveness of biochips is another major consideration. Although these devices can potentially decrease overall healthcare costs through early detection and precise diagnostics, the initial investment required for development and manufacturing is substantial. This financial burden can inhibit wider adoption among healthcare providers, especially in regions with limited resources.
Production costs must be weighed against the benefits biochips offer in terms of personalized medicine and targeted therapies. For example, while a biochip might lead to a more accurate treatment plan, its high price could limit its use to well-funded healthcare systems or research institutes.
In many cases, it is necessary to provide a clear return on investment for healthcare facilities that implement these technologies. Current economic models must be adapted to demonstrate the long-term savings associated with the early detection capabilities of biochips. Thus, a balance between upfront costs and potential savings needs to be established.
In summary, the challenges and limitations surrounding biochips play a crucial role in their acceptance and integration into healthcare. Addressing the technical obstacles and ensuring cost-effectiveness will be crucial in realizing the full potential of biochips as transformative tools in medicine.
Future Prospects
The future prospects of biochips signify a pivotal point in medicine and technology. As biochips evolve, their integration into healthcare could revolutionize diagnostics, treatment, and patient monitoring. These advancements will lead to more personalized healthcare solutions. An emphasis on emerging technologies and potential applications is crucial for understanding how biochips might impact human biology and medical practices in the coming years.
Emerging Technologies
Several emerging technologies signal the next wave of biochip innovation. One notable area is the development of miniaturized sensors that can perform real-time monitoring of biomarkers in bodily fluids. Such sensors could enable continuous health monitoring, translating to quicker responses to health issues. Additionally, advancements in nanotechnology are boosting the sensitivity and functionality of biochips. These nanostructures can enhance biochip efficiency, allowing for more accurate results from smaller sample sizes.
Another promising avenue of development is the integration of artificial intelligence with biochips. AI algorithms can analyze vast amounts of data generated by biochips, making it possible to detect patterns that may escape human analysis. This synergy may lead to breakthroughs in disease prediction and management, with the potential to foresee health conditions before symptoms arise.
Potential Applications
The potential applications of biochips span various fields, primarily focused on medicine. One of the most significant areas is in disease management. Biochips could facilitate rapid diagnosis of infectious diseases, thereby curbing outbreaks and improving public health responses. For example, the capacity to quickly identify viral load through simple biosensor tests could vastly improve treatment protocols.
Furthermore, biochips hold immense promise in personalized medicine. By analyzing genetic information, healthcare providers can design tailored treatment plans. This will enhance the efficacy of therapies by aligning them more closely with individual patient needs. Pharmacogenomics, which studies how genes affect a person's response to drugs, will benefit from biochip applications, ensuring better outcomes and fewer adverse effects.
"Biochips are not just a technological curiosity but a transformative force within modern medicine."
In addition to diagnostics and personalized treatment, biochips can enhance clinical research. They may allow researchers to conduct high-throughput screenings of drug candidates, aiding in the rapid development of new therapies. This could shorten the time from initial discovery to clinical application, which is a considerable hurdle in drug development.
Overall, the trajectory of biochips in future healthcare systems is promising. As their capabilities expand, they will likely become integral tools not only for diagnosis and treatment but also for preventive health strategies. Their integration into everyday medical practices could lead to healthier populations and more efficient healthcare systems.
End
The conclusion of this article synthesizes the critical findings on biochips and their implications in human biology and healthcare. Biochips stand out as significant instruments, offering various benefits that pave the way for innovative applications in medicine, diagnostics, and personalized therapies.
In recent years, the integration of biochips in clinical settings has demonstrated enhanced efficiency in disease detection and management. The ability to rapidly analyze genetic information or protein expressions can lead to earlier interventions. Moreover, the ongoing development of lab-on-a-chip technologies illustrates the shift toward decentralized and accessible healthcare solutions. These advancements can minimize traditional laboratory constraints, making advanced diagnostics available to a wider population.
However, ethical considerations cannot be overlooked. Concerns regarding privacy, informed consent, and equitable access to technology must be addressed as biochips evolve. Awareness and regulation are necessary to prevent misuse and ensure fair distributions of benefits.
Thus, the importance of understanding biochips extends beyond their technical prowess. It invites a comprehensive discourse around the implications they carry. Stakeholders must remain vigilant about ethical practices, innovative applications, and the nurturing of an inclusive technological landscape in the future.
Summary of Findings
The investigation into biochips has revealed several key points:
- Diversity of Applications: Biochips facilitate diagnostics, treatment customization, and monitoring of health conditions.
- Ethical Challenges: Privacy, consent, and access equity are pressing social considerations.
- Technological Advancement: Features like miniaturization and increased efficiency are pushing the boundaries of medical capabilities.
- Future Trajectory: Emerging technologies show promise for broader usage, but regulatory frameworks will need adaptation.
As biochips gain traction, their influence will significantly shape healthcare's future, further emphasizing the need for both innovation and responsibility.
Final Thoughts
Looking ahead, the role of biochips in human health warrants ongoing examination. Their integration into everyday medical practices embodies a transformative potential that can personalize medicine and enhance diagnostic accuracy. However, the excitement surrounding these innovations must be tempered with critical evaluation of ethical procedures and societal impacts.