CRISPR-Cas9 Innovations in Gene Editing and Ethics
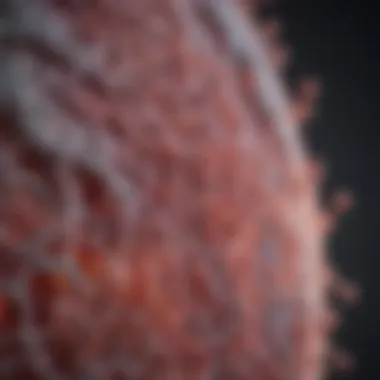
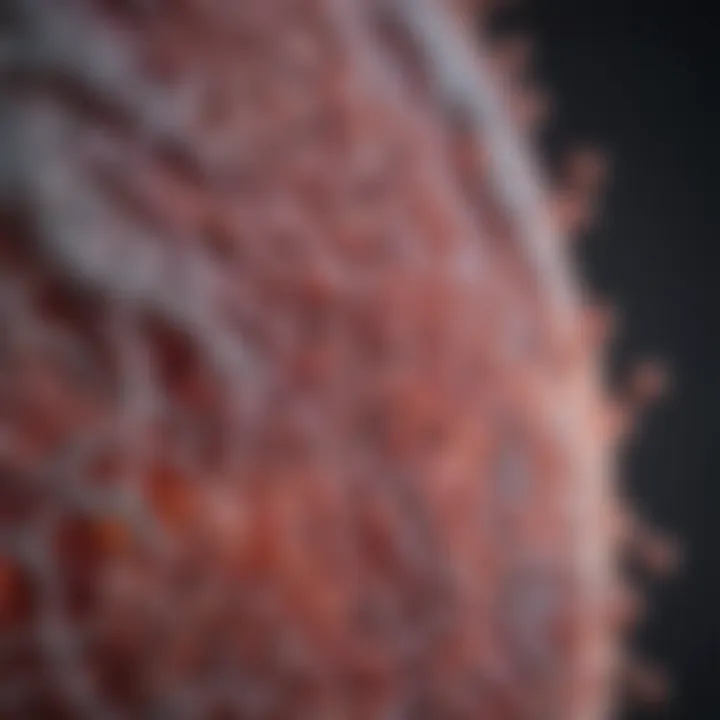
Intro
CRISPR-Cas9 technology has revolutionized the field of molecular genetics, emerging from the fascinating intersection of bacterial immunity and genetic engineering. The name CRISPR stands for clustered regularly interspaced short palindromic repeats, which are part of the adaptive immune system in bacteria. This natural mechanism allows bacteria to defend themselves against viral attacks by storing snippets of viral DNA and using them to recognize and destroy subsequent infections. The adaptation of this bacterial defense mechanism into a tool for gene editing represents a monumental advancement in biotechnology.
Understanding CRISPR-Cas9 involves not only grasping its origins but also appreciating its applications in various fields, including medicine, agriculture, and environmental science. The technology allows scientists to edit genes with unprecedented precision and efficacy, leading to potential cures for genetic diseases, enhanced crop resilience, and novel methods for disease control.
This article leads readers through the intricacies of CRISPR-Cas9, examining how it works, its origins in bacteria, and the ethical considerations that accompany its use. With an intention to provide comprehensive insight into this revolutionary technology, we aim to discuss both the significant benefits and the potential risks associated with gene editing.
Through this exploration, a clearer picture will emerge regarding CRISPR-Cas9's transformative impact on science and society.
Foreword to CRISPR Technology
CRISPR technology has fundamentally changed the landscape of genetic research and manipulation. Understanding its origins and mechanisms will shed light on how this tool became a cornerstone in biotechnology and genetics. This section serves as a gateway into the world of CRISPR-Cas9, illustrating its significance and implications.
Origins of CRISPR in Bacteria
CRISPR systems originate from bacteria, where they function as adaptive immune responses. They provide a defense mechanism against phages, which are viruses that infect bacteria. This system was first recognized in Escherichia coli in the 1980s but gained scientific attention in the early 2000s. The term CRISPR stands for "Clustered Regularly Interspaced Short Palindromic Repeats." These sequences serve as a genetic memory of past viral infections, allowing bacteria to recognize and combat subsequent challenges from the same viruses.
When a bacterium survives a viral invasion, it incorporates a segment of the viral DNA into its own genome. This segment is stored as a CRISPR array. If the bacterium encounters the virus again, it uses this information to produce RNA that matches the viral DNA, guiding CRISPR-associated proteins to the target. This complex ultimately cleaves the viral genetic material, effectively neutralizing the threat. The understanding of these natural mechanisms has led researchers to repurpose CRISPR for gene editing in other organisms, making it a revolutionary tool in biotechnology.
Mechanisms of CRISPR-Cas9
The CRISPR-Cas9 mechanism involves multiple components that work in synergy to edit genetic material. At its core, Cas9 is an endonuclease that creates double-strand breaks in DNA at specific locations. This precision is facilitated by guide RNA (gRNA), which is designed to match the target DNA sequence. The role of the gRNA is crucial, as it determines the specificity of the Cas9 binding.
Upon introduction into a cell along with the gRNA, the Cas9 protein searches the genome for the complementary sequence. Once identified, it binds to the DNA and introduces the cut, ensuing a repair process. Cells typically mend breaks through two main pathways: non-homologous end joining or homology-directed repair. Researchers exploit these pathways to either insert new genetic material or knock out genes, enabling a variety of genetic modifications.
"CRISPR technology harnesses the natural defense mechanisms of bacteria and translates them into practical applications in gene editing."
This capability makes CRISPR-Cas9 a powerful tool across various fields, including agriculture, medicine, and genetic research. Its simplicity in design and ease of use has opened many doors to precise genetic modifications that were once deemed complex or unfeasible. Understanding these mechanisms is essential for comprehending the broader applications and limitations of CRISPR technology.
The Bacterial Immune System
The concept of the bacterial immune system is significant in understanding CRISPR-Cas9 technology. Bacteria are exposed to a wide range of viruses, particularly bacteriophages, which pose threats to their survival. In response to these threats, bacteria developed sophisticated defense mechanisms, which are critical both for their existence and for the advancement of gene editing technologies.
Bacterial immune systems are not only fascinating due to their complexity but also because they serve as the foundation of CRISPR technology. This system allows bacteria to store segments of viral DNA, providing a form of adaptive immunity. When a bacterium encounters a virus, the immune system can recognize and combat it based on this stored memory.
Understanding these mechanisms reveals how they can be harnessed in gene editing. The mechanisms of the CRISPR system are intricately linked to bacterial survival strategies. By studying these systems, scientists have been able to develop tools for precise genome editing, which has far-reaching implications in various fields.
Understanding Bacterial Defense Mechanisms
Bacterial defense mechanisms cooperate to protect against genetic invaders. These mechanisms include the restriction-modification system, which cuts foreign DNA, and the CRISPR system, which records past infections. The CRISPR system can be divided into three stages: acquisition, expression, and interference.
- Acquisition: The bacterium integrates a segment of viral DNA, called a spacer, into its own genome.
- Expression: This spacer is transcribed into RNA, which is then processed into small CRISPR RNAs (crRNAs).
- Interference: The crRNA guides a complex that targets and cleaves the foreign DNA upon subsequent infections.
This adaptive approach significantly enhances the ability of bacteria to survive evolving threats.
Role of Cas Proteins
Cas proteins, or CRISPR-associated proteins, are crucial for the function of the CRISPR system. They are responsible for the recognition and cleavage of target DNA sequences. Cas9, the most widely studied of these proteins, is particularly important in gene editing applications. It is guided by the crRNA to the specific location in the DNA.
- Functionality: Cas proteins are nucleases, meaning they can cut DNA. This capability is harnessed to edit genes in various organisms, including plants and animals.
- Specificity: Cas proteins can be engineered to increase their ability to target specific DNA sequences, reducing off-target effects.
- Versatility: Beyond cutting DNA, some Cas proteins have been modified for applications such as gene activation or repression, broadening the scope of gene editing tools.
"Understanding the mechanisms and roles of Cas proteins is pivotal for advancing CRISPR technology and its applications."
In summary, the bacterial immune system provides a unique blueprint for innovation in molecular biology. By delving into the defense mechanisms and the role of Cas proteins, we grasp the profound implications of CRISPR-Cas9 technology for modern science. This knowledge not only informs the applications of gene editing but also opens the door to ongoing research and development in the field.
CRISPR-Cas9 Mechanism Explained
The CRISPR-Cas9 mechanism stands as a cornerstone in the realm of molecular genetics and biotechnology. Its significance in gene editing cannot be overstated. This technology offers unprecedented precision in modifying DNA, enabling researchers to manipulate genes with a level of accuracy that was previously unimaginable. Understanding how this mechanism works is essential for grasping its applications and the underlying science that tether CRISPR technology to bacterial immunity.
At its core, the CRISPR-Cas9 system is akin to a programmable pair of molecular scissors. It allows scientists to target specific sequences in the genome and induce modifications. This ability to edit genes has far-reaching implications, from agricultural improvements to potential cures for genetic disorders. The next sections delve deeper into the two fundamental components of CRISPR-Cas9: guide RNA and DNA targeting.
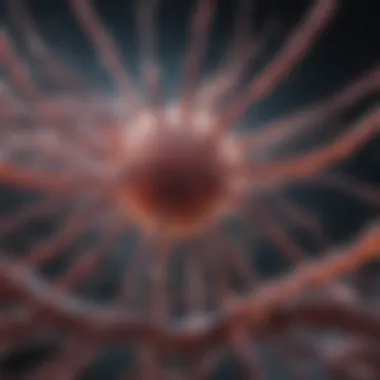
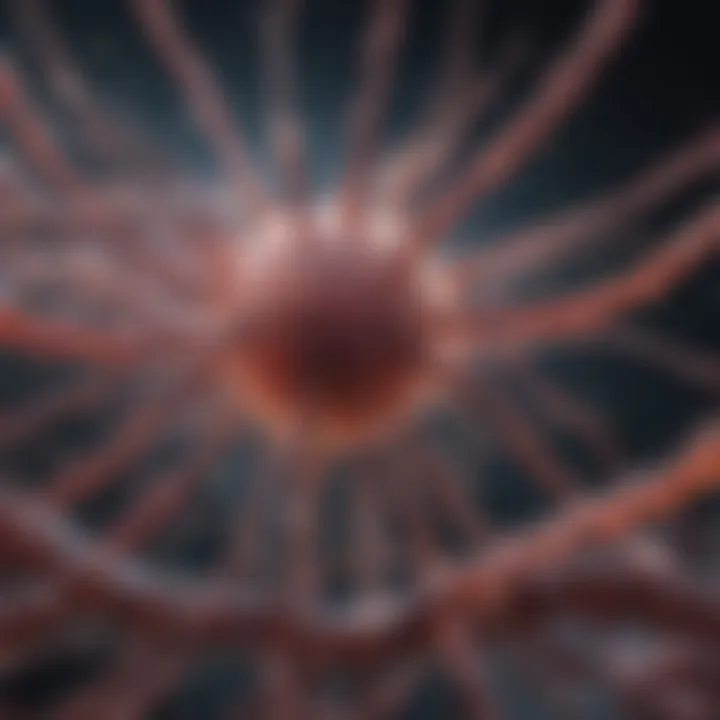
Guide RNA and Its Functions
Guide RNA, often abbreviated as gRNA, serves as a crucial element in the CRISPR-Cas9 mechanism. It is designed to align with a complementary sequence in the target DNA. By binding to the specific DNA sequence, gRNA directs the Cas9 protein to its intended location. This targeting function is what allows the system to be so versatile; researchers can create gRNAs that match almost any target sequence.
The components of gRNA include the scaffold sequence and the guide sequence. The scaffold is essential for binding to Cas9, while the guide sequence is what determines the target. This dual functionality enables the flexibility of the CRISPR-Cas9 system in gene editing.
In practical applications, the synthesis of gRNA is a pivotal step. Scientists can artificially design gRNAs to target genes responsible for diseases or undesirable traits, allowing tailored interventions at the genetic level. The ability to customize gRNA for different targets expands the potential uses of CRISPR technology across various fields.
DNA Targeting and Cleavage
Once the gRNA has bound to the target DNA sequence, the Cas9 protein performs its function of DNA cleavage. The Cas9 protein introduces double-strand breaks at specific sites in the DNA. This break in the DNA strand is a critical step for gene editing, as it triggers the cell's natural repair mechanisms.
The process of DNA cleavage by Cas9 is remarkably precise, but it is essential to ensure the correct target is chosen. Off-target effects, where the Cas9 cuts unintended sequences, pose a significant challenge. Researchers continuously explore techniques to minimize these off-target events, aiming for the highest specificity.
After the break has been introduced, the cell attempts to repair the damaged DNA. There are two major pathways for repair: non-homologous end joining (NHEJ) and homology-directed repair (HDR). NHEJ often leads to insertions or deletions, resulting in gene knockout, while HDR allows for more precise editing if a template DNA is provided. This flexibility in repair pathways is what fuels the diverse applications of CRISPR-Cas9.
The combination of gRNA's targeting ability with Cas9's cutting capability elucidates the powerful mechanism behind CRISPR-Cas9 technology. As research progresses, understanding this mechanism becomes imperative for advancing the applications and improving the efficiency of gene editing.
Applications in Gene Editing
Gene editing has made significant progress with the advent of CRISPR-Cas9 technology. This innovative tool facilitates precise modifications to DNA, enabling various applications that hold substantial implications for science and society. As gene editing becomes more prevalent, understanding its applications is crucial for appreciating its transformative impact.
Biotechnology and Agriculture
Biotechnology has embraced CRISPR-Cas9 to improve agricultural practices. This technology enables scientists to modify the genomes of crops, resulting in enhanced traits like pest resistance or drought tolerance. For example, researchers have developed CRISPR-edited rice that can thrive in saline soils. Such advancements not only help to increase food security but also promote sustainable agricultural practices.
Additionally, CRISPR allows for the development of crops without introducing foreign genes, addressing concerns related to genetically modified organisms (GMOs). This facilitates regulatory approvals, providing a pathway for faster adoption of beneficial innovations in the agricultural sector. Efforts to reduce crop losses due to pests and diseases can significantly impact yield and farmer livelihood.
Biomedical Research
In biomedical research, CRISPR-Cas9 plays a vital role in understanding genetic diseases and the development of therapies. The technology is used to create models of human diseases in organisms such as mice or stem cells. Scientists can investigate gene functions and identify potential targets for treatment by knocking out specific genes or introducing mutations.
Moreover, CRISPR is a powerful tool for drug development. Researchers are using CRISPR to discover new compounds and therapeutic approaches. The ability to conduct high-throughput genetic screens accelerates the pace of drug discovery, contributing to more efficient development processes. With the growing repository of knowledge supported by CRISPR, new insights into complex disease mechanisms are becoming increasingly attainable.
Potential in Disease Treatment
The potential for using CRISPR-Cas9 in treating genetic disorders has generated much enthusiasm. By directly editing genes associated with diseases like cystic fibrosis, sickle cell anemia, and muscular dystrophy, there is hope for curative therapies. Techniques like base editing and prime editing, which build on the CRISPR platform, offer even higher precision, reducing off-target effects that have been a concern in earlier applications.
Current clinical trials illustrate the promise of CRISPR in human medicine. For example, trials are underway to evaluate the safety and efficacy of CRISPR therapies for treating certain types of cancer. As the results of these studies emerge, they will pave the way for broader applications in human health.
The potential for CRISPR technology to cure genetic disorders marks a pivotal moment in the history of medicine, opening doors to possibilities that were previously unimaginable.
Understanding the applications of CRISPR-Cas9 in biotechnology, agricultural enhancement, biomedical research, and disease treatment is essential. These applications underscore a significant shift in our approach to genetic manipulation. As the technology matures, its societal implications will demand careful consideration in both ethical and regulatory landscapes.
Ethical Considerations in CRISPR Use
The significance of ethical considerations in CRISPR use cannot be overstated. This topic arises due to the powerful capabilities of CRISPR technology, particularly in gene editing. It prompts necessary discussions among scientists, ethicists, and the general public. As CRISPR advances rapidly, the implications of its use become increasingly complex. Decisions made today can impact future generations, not just in terms of health and agriculture but also in the broader fabric of society.
Debates Surrounding Germline Editing
Germline editing involves changes made to the DNA in sperm or eggs, which are then passed down to future generations. This process has become a focal point of ethical debate. Supporters argue that it holds the potential to eliminate genetic diseases. For instance, conditions such as cystic fibrosis or sickle cell anemia could be corrected at the embryonic stage. This could lead to healthier populations and significantly reduced healthcare costs in the long run.
On the other hand, critics express concerns about the unpredictable long-term effects. One major worry is the possibility of unforeseen genetic mutations, which could cause more harm than good. There’s also the question of accessibility and equity. If such treatments become commercially available, will they only be accessible to the wealthy? This could widen existing health disparities.
Additionally, the potential for non-therapeutic modifications raises ethical flags. Designing traits such as height, intelligence, or athletic ability might arise, leading to a future where some individuals are seen as more desirable than others. This touches on fundamental questions about what it means to be human and whether it is right to play with the building blocks of life.
Regulations and Policy Challenges
Regulatory frameworks surrounding CRISPR and gene editing technologies are still developing. Different countries have varied stances on the use of CRISPR, particularly for germline editing. For instance, in the United States, the Food and Drug Administration has not yet approved germline editing for clinical purposes, while nations like China have been more progressive in their approach.
The lack of a standardized global policy creates a landscape filled with challenges. Without clear regulations, there is room for misuse. Unregulated experiments might lead to health crises or further societal divides. Ensuring the safety and efficacy of CRISPR applications remains a primary concern, which calls for strong scientific oversight and ethical discourse.
In response to these concerns, many organizations advocate for a framework that ensures ethical use of CRISPR. This includes public engagement in discussions about the future of gene editing technology. Transparency in research and open communication will help bridge the gap between scientific advancement and ethical accountability.
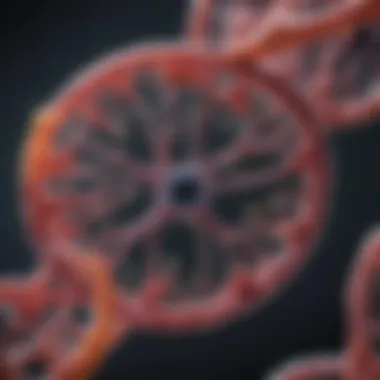
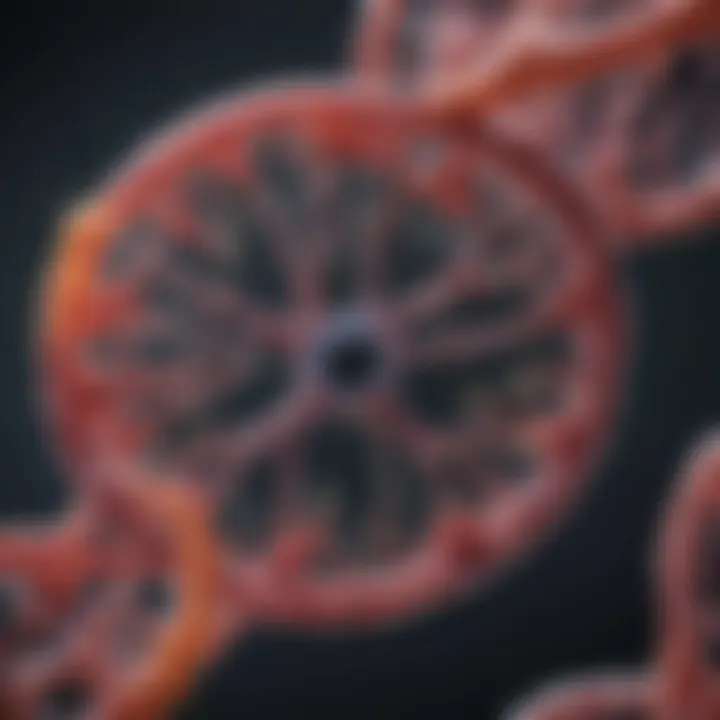
"The interplay of ethics and science is essential to responsibly navigate the future of CRISPR technology."
Establishing comprehensive guidelines can help balance innovation with moral responsibilities. A collaborative approach involving policymakers, scientists, and ethicists will be key to navigating this rapidly evolving landscape.
Future Prospects of CRISPR Technology
The potential future of CRISPR technology is vital for understanding how it can continue to influence our lives. The innovations developing from this technology can usher in new advancements not only in molecular biology but across various fields. Recognizing the importance of future applications can lead to new ways to address many of society's most pressing concerns. As researchers engage with CRISPR-Cas9, they embrace its flexibility for use in diverse contexts such as agriculture, medicine, and more.
Innovations in CRISPR Applications
Innovations in CRISPR applications are noteworthy as they expand the technology's reach. Researchers have explored numerous avenues for practical implementation, focusing on enhancing existing methods or developing new strategies. Several significant innovations include:
- Base Editing: This technique allows for precise changes at a single base pair level without cutting the DNA backbone. The implications for treating genetic disorders are substantial.
- Prime Editing: Touted as a more efficient editing tool, prime editing can achieve targeted genomic editing with greater accuracy than previous methods. It's a promising pathway for future research in curing genetic conditions.
- CRISPR-based Diagnostics: Notably, CRISPR's capacity for detection has led to its use in diagnostics. Tests powered by CRISPR, like SHERLOCK and DETECTR, can rapidly identify pathogens, which is critical for public health.
These innovations showcase the ongoing evolution of CRISPR technology and its remarkable versatility. The potential for developing targeted therapies for complex diseases hinges on these advancements, paving the way toward tailored medicine for various genetic conditions.
Integration with Synthetic Biology
The integration of CRISPR technology with synthetic biology enhances its capabilities even further. Synthetic biology focuses on designing and constructing new biological parts or systems. By combining this with CRISPR, scientists can craft tailored organisms with specific traits or functions.
Some examples of how this integration is reshaping fields include:
- Biofuel Production: Tailored microorganisms can be developed to optimize biofuel yield through targeted metabolic pathways. This can offer more sustainable energy sources.
- Pharmaceuticals: The ability to program cells to produce desired compounds can streamline drug development processes, reducing time and costs associated with traditional methods.
- Ecological Engineering: By modifying organisms to better adapt to changing environments, scientists can preserve biodiversity and improve ecosystem resilience.
The integration of CRISPR with synthetic biology is changing our approach to biological problems, showing how closely intertwined they have become.
Challenges in CRISPR Research
The research surrounding CRISPR technology is not without its challenges. Despite its potential transformative applications in gene editing, several factors impede progress and warrant careful consideration. Addressing these challenges is crucial for the effective utilization of CRISPR and its integration into both research and clinical practice.
Off-Target Effects
One of the primary concerns in CRISPR research is the possibility of off-target effects. This refers to instances where the CRISPR-Cas9 system unintentionally modifies sequences in the genome that differ slightly from the intended target. These unintended edits can lead to problematic consequences, including unintended gene disruption or activation, which may result in unforeseen cellular responses or pathological states.
To illustrate, a study found that off-target mutations can occur within a single base pair deviation, underscoring the precision required in gene editing. As a result, developers continuously work on improving specificity to minimize these effects. Techniques such as optimizing guide RNA design and employing high-fidelity Cas9 variants have shown promise, yet they require rigorous validation to ensure safety in therapeutic applications. Public understanding of off-target effects is equally important, as misconceptions may arise regarding the overall reliability of CRISPR technology.
Technical Limitations
Technical limitations also pose significant barriers in CRISPR research. While the CRISPR-Cas9 system has demonstrated remarkable versatility and ease of use, challenges remain in areas such as delivery mechanisms, efficiency, and scalability. Current delivery methods, including viral vectors or lipid nanoparticles, can sometimes lead to low transfection rates or immune responses. This limits the overall effectiveness in various applications, particularly in therapeutic settings where precise dosage and targeted delivery are essential for success.
Furthermore, the scaling of CRISPR technology for large-scale applications involves complexities that still require solutions. Researchers must navigate these hurdles to enable broader use across healthcare and agricultural sectors. These technical considerations are fundamental for advancing research practices and optimizing outcomes.
"As CRISPR technology evolves, addressing off-target effects and technical limitations will be key to unlocking its full potential."
Continuous innovation in both off-target mitigation and delivery strategies will play a pivotal role in overcoming these impediments. Ultimately, collaboration between scientists, engineers, and policymakers is necessary to facilitate the advancement of CRISPR technology in effective ways that can withstand scrutiny from the scientific community and the public.
Comparative Analysis of Gene Editing Techniques
The comparative analysis of gene editing techniques is essential to understand the unique features and potential limitations of CRISPR-Cas9 in the broader landscape of genetic manipulation. This examination not only highlights the efficacy of CRISPR-Cas9 but also illustrates its distinct advantages and challenges relative to other methods. As CRISPR technology grows more prevalent, it is crucial to educate students, researchers, educators, and professionals about the nuances that differentiate these methods.
CRISPR vs. Traditional Gene Editing Methods
Traditional gene editing methods, such as zinc-finger nucleases (ZFNs) and transcription activator-like effector nucleases (TALENs), have been foundational in the field of genetics. However, they present certain limitations in terms of complexity and cost.
- Ease of Design: CRISPR-Cas9 offers a simpler design process for guide RNA compared to the laborious crafting of protein motifs in ZFNs and TALENs. This ease allows researchers to quickly modify genetic sequences with reduced time and resource investment.
- Multiplexing Capability: CRISPR-Cas9 can target multiple genes simultaneously, a significant advantage over traditional methods that only target one gene at a time. This multiplexing is critical for complex genetic studies.
- Accessibility: CRISPR technology is generally more accessible for laboratories due to lower costs associated with reagents and tools.
- Off-Target Effects: Despite its advantages, CRISPR-Cas9 is not without flaws. Off-target effects can lead to unintended modifications in the genome, which is a concern that traditional gene-editing methods minimize.
The choice between these techniques often hinges on specific research goals, genetic contexts, and the desired precision of gene editing.
Emerging Technologies in Gene Editing
The world of gene editing does not stand still, and new technologies continue to emerge that may provide alternatives or enhancements to CRISPR-Cas9. Some notable innovations include:
- Base Editing: This technique enables the conversion of one DNA base into another without causing double-strand breaks. It offers higher precision and reduces the chances of unintended mutations compared to CRISPR-Cas9.
- Prime Editing: Often referred to as a "molecular pencil," this method allows researchers to make targeted alterations to the genome more accurately than traditional CRISPR approaches. It addresses some limitations associated with off-target effects.
- CRISPR-Cas12 and Cas13: These novel CRISPR systems offer improved targeting capabilities and can be used for applications such as diagnostics and RNA targeting, expanding the versatility of CRISPR technology.
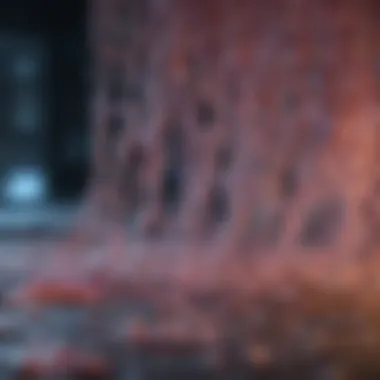
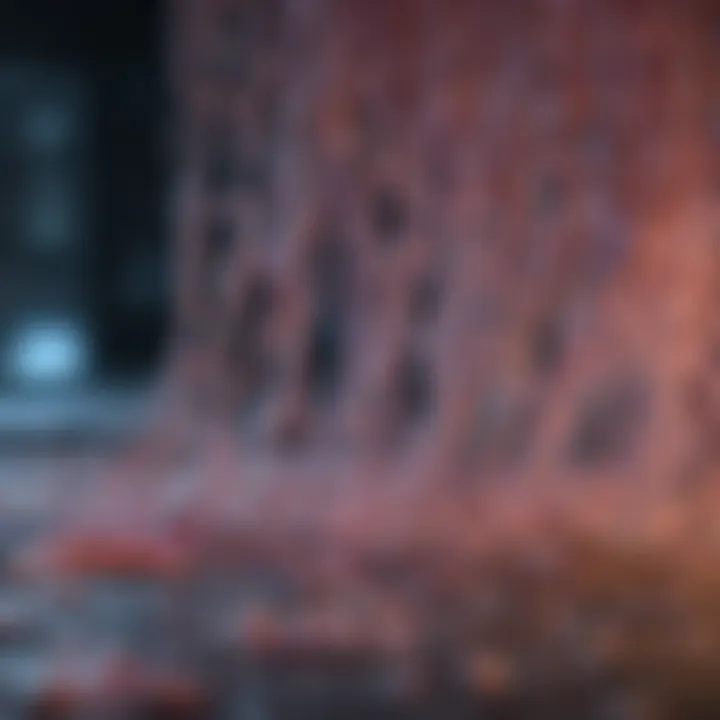
"Understanding the comparative aspects of gene editing techniques is crucial for making informed decisions in scientific research."
This detailed analysis fosters a deeper appreciation of the evolving landscape of gene editing, ensuring that both current and future innovations are critically evaluated for their potential impact.
Public Perception and Awareness of CRISPR
Public perception and awareness of CRISPR technology are vital factors influencing its development and application. As the CRISPR-Cas9 system progresses from a scientific novelty to a practical tool in various fields, understanding how the wider community perceives this technology becomes essential. Public sentiment can shape research funding, regulatory frameworks, and ultimately the ethical landscape surrounding gene editing.
The benefits of having a well-informed public about CRISPR are numerous. Awareness enables citizens to engage in conversations about the implications of gene editing, fostering a more informed debate. This dialogue is crucial for ensuring that innovations align with societal values and ethical standards. Educated citizens can advocate for responsible usage and policies that govern CRISPR technology, thus playing a crucial role in its ethical deployment.
Despite the advantages, misconceptions about CRISPR and gene editing persist. For instance, many people equate CRISPR with "designer babies" or fear its use in altering human genetics without proper oversight. Such misunderstandings can lead to resistance against scientific advancements. Hence, addressing misinformation is necessary for clear public perception, encouraging constructive discourse regarding innovation.
"Informed public discourse can drive ethical frameworks and responsible policies in gene editing."
Institutions, researchers, and educators must work collaboratively to improve awareness about CRISPR’s nature, applications, and implications. In doing so, these efforts can foster an environment where ethical and social considerations are at the forefront of scientific advancements.
Educational Initiatives
Educational initiatives play a crucial role in shaping public understanding of CRISPR technology. Many institutions have begun integrating scientific literacy into their curriculums. This includes workshops, seminars, and educational programs aimed at demystifying gene editing processes. Understanding the foundational principles behind CRISPR helps bridge the gap between complex science and community understanding.
Popular science blogs, open courses, and public lectures serve as useful tools for delivering information. By presenting data in an accessible format, these initiatives can engage various audiences, from high school students to older adults. The emphasis should always be on critical thinking, encouraging individuals to assess both the benefits and risks associated with CRISPR.
Media Representation of CRISPR
Media representation has a significant impact on how CRISPR is perceived by the public. Coverage in both mainstream and scientific media shapes narratives around gene editing. Positive portrayals of breakthroughs can foster excitement and support for research. However, sensationalism often leads to fear and misunderstanding. Reports focusing solely on dystopian scenarios or genetic enhancements can skew perceptions, making the public wary of even beneficial applications.
It is imperative for media outlets to strive for balanced reporting. Fact-checking and expert opinions should be standard features in articles discussing CRISPR. Engaging expert scientists in public discourse via interviews and public forums can help clarify complex topics. Journalists must also highlight responsible research practices and ongoing regulatory discussions, providing readers with a broader context.
Case Studies of CRISPR Applications
Case studies serve as powerful tools for understanding the practical implications of CRISPR technology. They provide concrete examples of how gene editing can be applied in real-world scenarios. This section highlights the significance of CRISPR applications in agriculture and medicine, demonstrating the technology’s versatility and transformative potential.
CRISPR in Agriculture
In agriculture, CRISPR technology is fundamentally changing how crops are developed. Farmers face numerous challenges, including climate change, pest infestations, and soil degradation. CRISPR enables the development of crops that can withstand adverse conditions. These include drought-tolerant corn and pest-resistant soybeans. By editing specific genes, scientists can enhance desired traits in plants more efficiently than traditional breeding methods.
Another prominent example is the development of improved rice varieties. Golden Rice, for instance, has been engineered to produce beta-carotene, a precursor to vitamin A. This modification addresses malnutrition, especially in developing countries. Furthermore, CRISPR can reduce the reliance on chemical pesticides, promoting more sustainable farming practices.
"The benefits of CRISPR in agriculture extend beyond yield. They encompass environmental sustainability and enhanced nutritional profiles."
Despite these advantages, ethical considerations surround the use of gene editing in crops. Concerns about biodiversity loss and the long-term effects of modified plants on ecosystems remain prevalent. Regulatory frameworks must adapt to address these issues effectively while promoting innovation.
CRISPR in Medicine
The medical applications of CRISPR technology are equally promising. Researchers are investigating its role in treating genetic diseases, cancers, and infectious diseases. For instance, sickle cell disease has been a focus for CRISPR trials. By editing the genes responsible for the production of abnormal hemoglobin, researchers aim to restore normal blood function in patients. Early trials have shown encouraging results, making the case for CRISPR's potential to cure genetic disorders.
Moreover, CRISPR technology is also being explored for its potential in combating viral infections. The technology can target and cut viral DNA or RNA, effectively inhibiting the virus's ability to multiply. This application has been investigated in the context of HIV and hepatitis B, among other viruses.
Another significant advancement is CRISPR's role in cancer treatment. Researchers are developing strategies to target cancer cells more precisely, sparing healthy cells from damage. This precision could revolutionize how treatments like chemotherapy are administered, leading to fewer side effects and more effective outcomes.
In summary, the case studies in both agriculture and medicine illustrate CRISPR's diverse applications. They highlight the urgency and importance of ongoing research and ethical considerations in the implementation of such powerful technology.
Epilogue
The exploration of CRISPR-Cas9 technology offers significant insights into the future of genetic engineering. This technology, originally derived from bacterial immune systems, has transformed our approach to genetic manipulation. The implications of CRISPR are vast, impacting fields such as agriculture, medicine, and environmental science.
Summary of Key Insights
CRISPR-Cas9 is not just a tool for gene editing; it represents a paradigm shift in biotechnology. Here are some key takeaways:
- Versatility of Applications: CRISPR can be used for precise gene editing across various organisms, offering solutions in agriculture for crop resilience and in medicine for treating genetic disorders.
- Ethical Considerations: The ability to modify genes raises ethical questions, particularly regarding germline editing. The discussions on regulations are ongoing and critical to ensure responsible use.
- Future Challenges: Issues like off-target effects and technical limitations need further research to enhance the precision and safety of CRISPR technologies.
Future Directions in CRISPR Research
Looking ahead, the research landscape for CRISPR-Cas9 is set to expand. Key areas for development include:
- Improving Specificity: Future studies will focus on minimizing off-target effects, making CRISPR more reliable for therapeutic applications.
- Combining Technologies: Integrating CRISPR with other gene editing tools could create new avenues for precision medicine.
- Broader Applications: The potential to address complex diseases and environmental issues will be areas of significant research interest.
CRISPR-Cas9 technology has the power to revolutionize fields across biology, but it comes with responsibilities that must be acknowledged.