CRISPR-Cas9 Knock-In Technology: Mechanisms and Implications
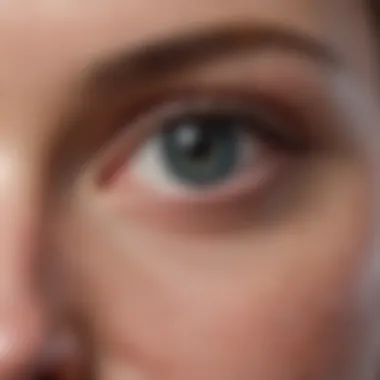
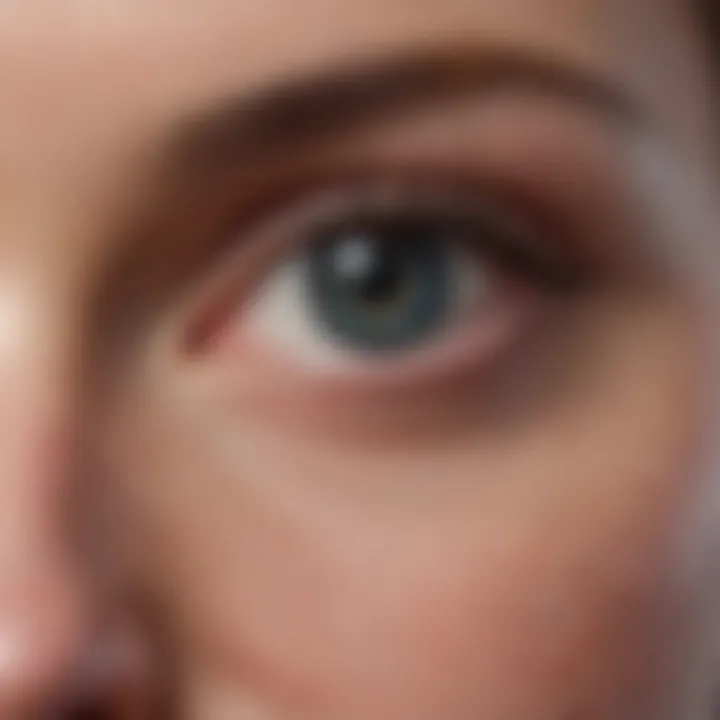
Intro
CRISPR-Cas9 knock-in technology represents a significant advance in the field of genetic engineering. Its mechanism is based on a natural defense system used by bacteria, which has been adapted for precise DNA editing. Understanding the nuts and bolts of this technology is vital for students, researchers, and professionals who are navigating the complexities of genetic modifications. This section provides a foundation for the discussion on CRISPR-Cas9 by outlining essential concepts and motivations behind this revolutionary approach.
Research Highlights
Key Findings
- Precision and Accuracy: CRISPR-Cas9 allows for targeted modifications to specific gene sequences with high precision. This minimizes unintended effects often observed in earlier gene-editing techniques such as zinc-finger nucleases.
- Broad Applicability: Research shows that the knock-in approach, in particular, has versatile applications in fields ranging from agriculture to medicine. For instance, it can be used to create genetically modified organisms that can withstand pests, or to develop treatments for genetic disorders in humans.
- Ethical Considerations: The use of CRISPR technology raises ethical issues, especially regarding its application in human germline editing. The potential to eliminate genetic diseases must be weighed against possible long-term consequences on human evolution and diversity.
"The ability to precisely edit genes opens doors to innovations, but the ethical implications cannot be brushed aside."
Implications and Applications
The implications of CRISPR-Cas9 are vast and transformative. One prominent application is in the realm of gene therapy, where defective genes linked to hereditary diseases are corrected or replaced. This offers hope to patients suffering from conditions such as cystic fibrosis or muscular dystrophy.
Moreover, CRISPR technology is being explored in the quest to develop crops that are more resilient to climate change. This includes increasing drought resistance or enhancing nutritional content.
As we consider these various applications, it is crucial to monitor the development of regulations and policies to ensure responsible use of this powerful technology.
Methodology Overview
Research Design
The research surrounding CRISPR-Cas9 involves a multidisciplinary approach, combining molecular biology, genetics, and bioinformatics. Researchers design experiments that seek to answer specific questions about the efficiency and safety of knock-in techniques.
Experimental Procedures
Experiments generally involve several key steps:
- Selection of Target Gene: Identifying the gene of interest is the first step.
- Designing gRNAs: Guide RNAs are designed to bind to the target DNA sequence.
- Cas9 Introduction: Cas9 enzyme, nicking the target DNA, is introduced alongside the gRNAs.
- Repair Template Insertion: A repair template is provided to facilitate the knock-in of desired sequences.
- Screening and Validation: Following editing, successful knock-ins are screened using techniques such as PCR or sequencing.
This overview of methodology highlights the systematic approach researchers take to harness CRISPR-Cas9 technology effectively.
Intro to CRISPR-Cas9
The CRISPR-Cas9 system has emerged as a transformative tool in the field of genetic engineering. This section serves to lay the groundwork for understanding how CRISPR-Cas9 operates and why it has garnered unprecedented attention in scientific communities around the world. The importance of CRISPR-Cas9 cannot be overstated, as it simplifies and enhances the precision of gene editing. With applications ranging from medicine to agriculture, its impact permeates several disciplines.
Historical Context
The journey of CRISPR-Cas9 began in the 1980s when scientists first discovered that certain bacteria had the ability to fend off viral infections using short, repeating DNA sequences. These sequences were later found to play a crucial role in the adaptive immune response of bacteria. In 2012, Jennifer Doudna and Emmanuelle Charpentier pioneered the adaptation of this bacterial system into a straightforward and efficient method for editing DNA in other organisms. This innovation facilitated breakthroughs across various research fields.
Mechanism of Action
To fully grasp CRISPR-Cas9’s significance, one must delve into its mechanism of action, which comprises several essential components.
Components of the CRISPR-Cas9 System
The CRISPR-Cas9 system consists mainly of two key elements: the Cas9 nuclease and the guide RNA (gRNA). The Cas9 nuclease is a protein that cuts DNA at specific locations, while gRNA directs this enzyme to the correct section of the genome. This targeted approach is a key characteristic of CRISPR-Cas9, setting it apart from earlier gene-editing technologies.
The versatility of the gRNA allows for easy modification, making it a popular choice for diverse applications. Its user-friendly nature simplifies the design process, enabling researchers to edit genes more efficiently than traditional methods. However, a unique feature of this system is that off-target effects may occur, potentially leading to unintended mutations in the genome, which is a drawback that needs addressing.
Target DNA Recognition
For CRISPR-Cas9 to function successfully, precise recognition of target DNA is critical. The gRNA binds to the complementary DNA sequence and guides Cas9 to the target site. The ability of gRNA to recognize specific DNA sequences is a vital aspect of the overall mechanism, enabling high specificity and efficiency in gene edits.
This feature enhances the potential applications of CRISPR technology. Nevertheless, careful design is necessary, as the accuracy of target DNA recognition can significantly influence the success of the editing process. Misrecognition of targets can result in undesired alterations.
DNA Cleavage Process
Upon successful recognition, the Cas9 protein cleaves the DNA strands. This cleavage is a decisive step, creating a double-strand break that directs the cell's repair machinery to initiate repair. The simplicity of the cleavage mechanism makes CRISPR-Cas9 extremely effective.
The key characteristic of the cleavage process lies in its controlled nature, allowing for predictable outcomes in gene editing. However, the repair mechanisms employed by cells can also lead to random insertions or deletions, introducing potential risks in genetic modifications. Understanding this process is crucial for developing effective applications.
Comparison to Traditional Genetic Engineering Techniques
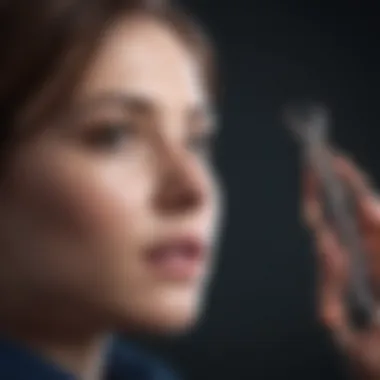
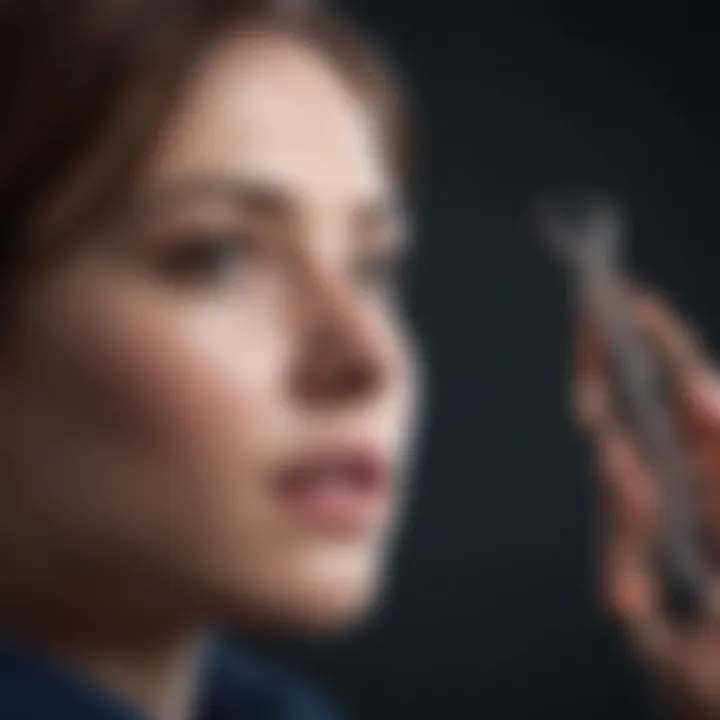
CRISPR-Cas9 stands in stark contrast to traditional genetic engineering techniques such as homologous recombination and site-specific nucleases. While older technologies were often time-consuming and required complex procedures, CRISPR-Cas9 streamlines workflows and offers higher precision and flexibility. Its efficiency has led to its rapid adoption in laboratories worldwide.
Adoption of CRISPR-Cas9 has opened new avenues for research and therapeutic applications. As technology evolves, it is vital to critically assess its implications and applications within society.
Understanding Knock-In Technology
Knock-in technology, leveraging the precision of CRISPR-Cas9, has become a crucial aspect of genetic engineering. This section explores the essence of knock-in technology, its practical applications, and its implications for various fields. Understanding this technology is vital for anyone involved in genetic research or applications as it opens a myriad of possibilities for gene modification and therapeutic interventions.
Definition and Overview
Knock-in technology refers to the process of inserting a specific gene or genetic element into a predetermined location within a genome. This method is particularly powerful because it allows researchers to introduce desired traits with high accuracy. In contrast to random integrations, knock-in ensures that the inserted genetic material becomes part of the target organism's biological makeup at a defined locus. This precise integration is vital for producing stable genetic modifications, facilitating the investigation of gene functions and pathways.
The significance of knock-in technology can be summarized as follows:
- Precision: Unlike traditional modification methods, this technology ensures that the desired genetic change occurs at a specific site, reducing the risk of unintended consequences.
- Stability: Changes made through knock-ins are often more stable than those made using other methods, allowing for longer-term studies.
- Functional Studies: It enables scientists to study gene functions and interactions in detail. Researchers can create models to mimic human conditions or diseases more accurately.
Furthermore, this technology helps in generating animal models that closely resemble human pathologies. Knock-in provides a robust framework for the production of specific mutations, making it possible to investigate hypotheses in genetic research or drug development.
Knock-In vs. Knock-Out Techniques
Both knock-in and knock-out techniques utilize CRISPR-Cas9 but serve different purposes.
- Knock-In: As previously mentioned, this method adds genetic material at a specified location. This is particularly useful in gene therapy, where introducing a functional copy of a gene can potentially correct a genetic disorder.
- Knock-Out: In contrast, the knock-out technique is used to disable a specific gene by introducing breaks in the DNA sequence, leading to disruption of the gene’s function. This method is crucial for studying gene functions by observing phenotypic consequences when a gene is rendered inactive.
In practical applications, the choice between knock-in and knock-out depends on the research goals. While knock-outs are useful in understanding loss-of-function mutations, knock-ins play a vital role in gene therapy applications aimed at correcting genetic defects.
The understanding of these techniques leads to faster advancements in treatments for genetic disorders, enhancement of crops, and the exploration of gene functionalities in various organisms.
"With the advancements in knock-in technology, we usher in new possibilities for gene therapy and agricultural biotechnology, reshaping the future of genetic engineering."
The exploration and application of knock-in technology will continue to drive innovations in multiple areas of science, proving to be a game-changer in how we approach genetic modifications.
Applications of CRISPR-Cas9 Knock-In Technology
The CRISPR-Cas9 knock-in technology has emerged as a pivotal tool in modern genetic engineering. Its applications extend into various fields such as medicine, agriculture, and research, showcasing its versatility and transformative potential. This section will discuss three major domains where this technology is making significant impacts: gene therapy, agricultural biotechnology, and basic research. Each area reveals unique aspects and benefits, contributing to advancements in science and practical applications.
Gene Therapy
Gene therapy represents one of the most compelling applications of CRISPR-Cas9 knock-in technology. This approach involves directly altering the genes within an individual's cells to treat or prevent diseases. Among the various aspects, targeting genetic disorders stands out.
Targeting Genetic Disorders
The ability to target genetic disorders using knock-in technology is crucial. This method allows for precise corrections of mutations responsible for diseases. A key characteristic of this approach is its potential to provide permanent solutions instead of temporary treatments. This makes it a favored choice for addressing inherited diseases, where the genetic cause must be corrected at the source.
A unique feature of targeting genetic disorders with CRISPR-Cas9 is its specificity. Unlike traditional methods that can cause unwanted changes, CRISPR can be designed to edit specific genes without affecting others. Its major advantage lies in the ability to restore gene function effectively. However, the challenge remains in ensuring complete precision to avoid off-target effects, which could lead to unintended consequences.
Advantages of Gene Correction
The advantages of gene correction using CRISPR-Cas9 knock-in technology are substantial, particularly in its effectiveness in treating diseases. This method enhances the potential for treating a wider array of disorders. One key trait is its ability to yield lasting results, as corrected genes can be maintained in the patient's DNA.
Gene correction through CRISPR-Cas9 offers a unique opportunity to not just alleviate symptoms but also eliminate the root cause of genetic disorders. This advancement is beneficial for both patients and the healthcare system, reducing long-term treatment costs and improving life quality. Despite these advantages, ethical discussions around the implications of gene editing in humans continue to arise, placing importance on responsible use of this technology.
Agricultural Biotechnology
Agricultural biotechnology is another area where CRISPR-Cas9 knock-in technology is essential. This application addresses the growing demand for food security and sustainable farming practices.
Crops with Enhanced Traits
Crops with enhanced traits symbolize a leap forward in agricultural practices. Using CRISPR, scientists can modify crops to improve yield, nutritional content, and tolerance to environmental stress. The key characteristic of this application is the ability to introduce beneficial traits without introducing foreign DNA, which is often a concern with traditional genetic modification methods.
A unique feature of enhancing crops is their rapid acceptance in the market. Since the modifications can be achieved in existing crops, farmers can adopt these improvements quickly. The advantages include reduced inputs like water and fertilizer. On the downside, there are ongoing debates regarding regulatory policies that could hinder the broader deployment of these innovations.
Resistance to Pests and Diseases
Another significant application within agricultural biotechnology is developing crops with resistance to pests and diseases. This aspect allows farmers to protect crops more effectively and reduce reliance on chemical pesticides. The central trait is its ability to enhance plant resilience.
The unique feature of CRISPR-induced resistance is its precision. By targeting specific genes associated with pest and disease susceptibility, crops can be engineered to endure various threats naturally. The benefits of this strategy include improved crop yields and lower environmental impact. However, there is still a challenge in addressing public concerns over genetically modified organisms (GMOs) and ensuring consumer acceptance.
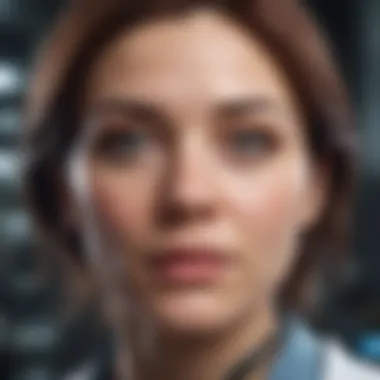
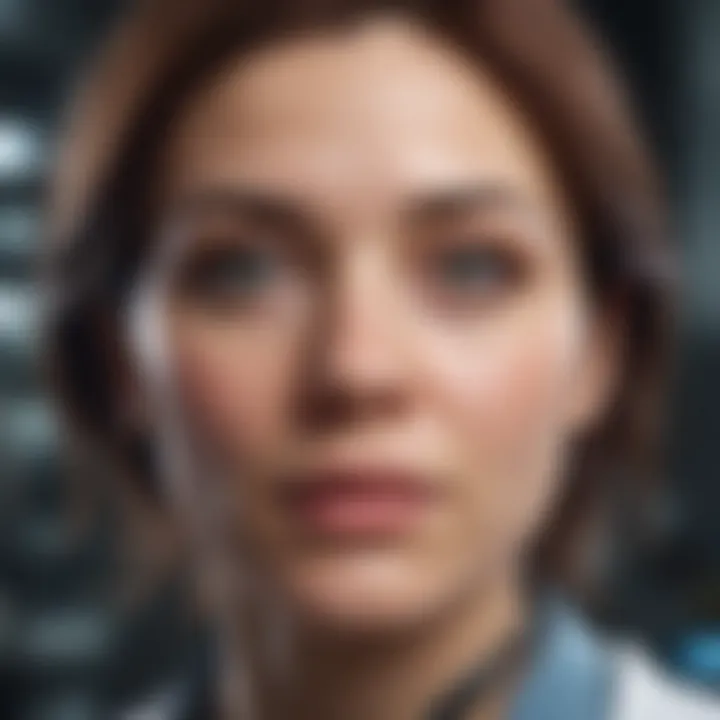
Basic Research and Functional Genomics
Lastly, basic research and functional genomics rely heavily on CRISPR-Cas9 technology. This field plays a critical role in understanding gene functions and exploring biological processes.
Studying Gene Functions
Studying gene functions is fundamental in biology, and CRISPR-Cas9 has simplified the process. A significant aspect of this application is the ability to edit genes in model organisms, which accelerates the discovery of gene roles in pathways and diseases. This brings a key advantage: faster identification of gene functions compared to previous methods.
Moreover, the unique ability of CRISPR to create precise knock-ins allows researchers to investigate gene regulation more thoroughly. However, understanding complex interactions remains a challenge, and researchers must also consider the implications of their findings on broader ecological systems.
Model Organisms for Research
Utilizing model organisms is a significant aspect of functional genomics. Organisms like zebrafish or mice serve as essential platforms for research in genetic research. They allow for detailed study of gene functions and interactions.
Model organisms are widely accepted in the scientific community, as they share many genetic pathways with humans. Their use in CRISPR research enables the efficient assessment of genetic alterations. However, while model organisms provide invaluable insights, translating findings to human applications can be complex due to biological differences.
"The applications of CRISPR-Cas9 knock-in technology extend far beyond human health, influencing agricultural practices and fundamental research, showcasing its profound versatility."
In summary, the applications of CRISPR-Cas9 knock-in technology demonstrate its transformative potential across multiple domains. Whether it’s improving health outcomes or enhancing food security, the implications of this technology are far-reaching, and its continued development promises to yield significant advancements.
Methodological Framework of CRISPR-Cas9 Knock-In
The methodological framework of CRISPR-Cas9 knock-in technology outlines the essential procedures for precise genetic modifications. Understanding this framework is crucial for researchers and professionals aiming to harness the full potential of gene editing. This section covers the steps necessary for successful knock-in, emphasizing the importance of designing guide RNA, constructing donor templates, employing efficient delivery methods, and screening edited cells for confirmation.
Designing gRNA
Selecting Target Sites
Selecting target sites is a foundational aspect of designing guide RNA (gRNA) for CRISPR-Cas9 knock-in applications. It involves identifying specific DNA sequences within a genome where double-stranded breaks can be introduced. The ideal target site must be close to the region where the knock-in will occur and have a protospacer adjacent motif (PAM) sequence. This characteristic ensures that Cas9 protein can bind effectively to the DNA.
The choice of target sites significantly contributes to the efficiency of the entire editing process. Selecting the right target sites can lead to higher success rates in knock-in experiments. A beneficial feature of this selection process is its adaptability across various organisms, making it a widely accepted practice in genetic engineering.
However, challenges arise if the chosen site is not unique to a genome. Off-target effects may occur, leading to unwanted mutations. Thus, careful evaluation of potential off-target sites is paramount while determining the best target.
Efficiency and Specificity Considerations
Efficiency and specificity considerations play a crucial role in the success of CRISPR-Cas9 knock-in technology. It is essential to ensure that the designed gRNA efficiently binds to the target DNA while minimizing off-target activity. One key characteristic is the length of the gRNA; it typically consists of 20 nucleotides, optimizing the balance between specificity and efficiency.
A popular choice for researchers is to use bioinformatics tools to predict the efficiency of gRNA candidates. These tools analyze potential gRNA sequences against the genome to estimate their likelihood of success. One unique feature of this approach is that it allows researchers to refine their gRNA selections iteratively, improving the overall specificity of their edits.
However, it is crucial to note that the efficiency of the gRNA can vary based on chromatin accessibility and genomic context. Thus, monitoring the performance of different gRNA across various target sites may be necessary.
Construction of Donor Templates
The construction of donor templates is another critical component of the CRISPR-Cas9 knock-in methodology. Donor templates are essential for providing the desired genetic information during the repair process following the introduction of double-strand breaks. These templates can take the form of single-stranded oligonucleotides or double-stranded DNA fragments, depending on the desired alteration to the genome.
An important aspect of donor template design is the incorporation of homology arms, which flank the intended edit. These homology arms facilitate the integration of the donor template into the genome during the DNA repair phase. An effective donor template must exhibit high fidelity, ensuring that the genetic change is accurately incorporated.
Cell Delivery Methods
Lentiviral Vectors
Lentiviral vectors are a widely used method for delivering CRISPR-Cas9 components into target cells. These vectors can efficiently transduce both dividing and non-dividing cells, making them versatile for various experimental settings. A significant advantage of lentiviral vectors is their ability to integrate into the host genome, allowing for prolonged expression of the CRISPR-Cas9 system.
This feature makes lentiviral vectors an attractive choice when stable knock-in events are desired. However, one disadvantage could be the potential for insertional mutagenesis, which poses a risk of disrupting essential genes or regulatory elements.
Electroporation Techniques
Electroporation techniques present another valuable approach for delivering CRISPR-Cas9 components into cells. This method uses an electrical field to increase cell membrane permeability temporarily. It allows for direct introduction of Cas9 protein and gRNA, or donor DNA into the cytoplasm.
The primary benefit of electroporation lies in its simplicity and speed. This technique can be applied to various cell types and is particularly useful for primary cells and stem cells. On the downside, controlling electroporation efficiency is challenging, as excessive electric fields may lead to cell death or damage.
Screening for Successful Knock-Ins
Molecular Techniques
Screening for successful knock-ins is essential to evaluating the effectiveness of CRISPR-Cas9 applications. Molecular techniques such as PCR (Polymerase Chain Reaction) and Sanger sequencing enable researchers to confirm the presence of the desired genetic modification. These methods provide reliable tools for analyzing edited DNA.
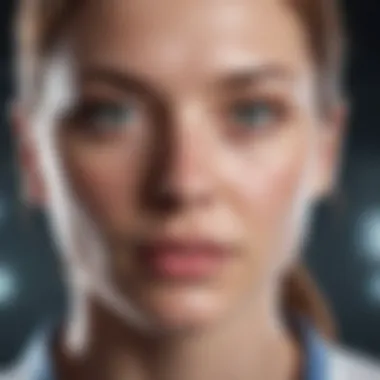
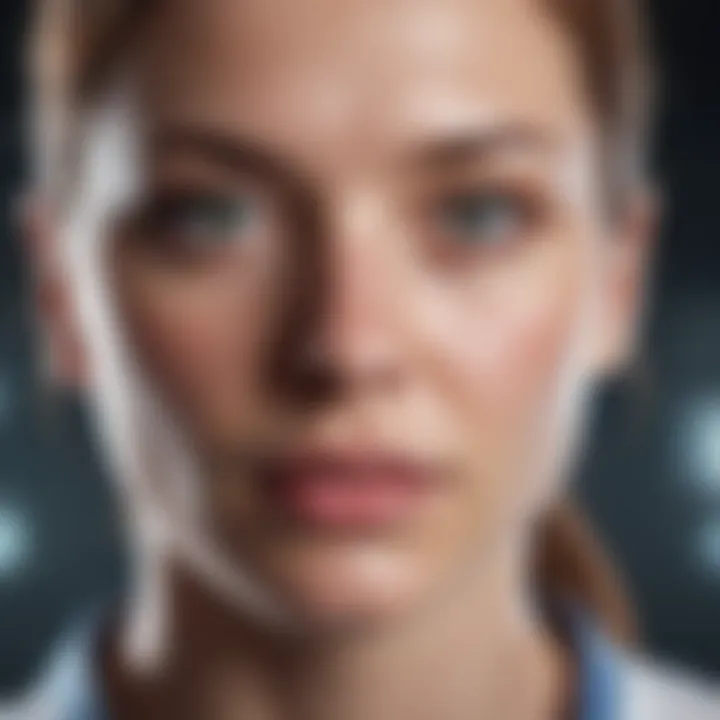
One key characteristic of molecular techniques is their ability to identify not just successful knock-ins, but also to assess potential off-target events. Researchers often pair these techniques with next-generation sequencing to gather comprehensive data about genetic edits.
Characterization of Edited Cells
Characterization of edited cells is final step to ensure that CRISPR-Cas9 knock-ins have the intended effect on the cellular phenotype. This step involves analyzing cellular functions, gene expression levels, and phenotypic changes. Characterization techniques may include flow cytometry, Western blotting, and other assays that assess cellular activity.
The distinctiveness of this characterization process lies in its ability to provide insights into the viability and functionality of edited cells. These analyses help elucidate whether the genetic change has led to the desired biological outcome, contributing to the robustness of CRISPR-Cas9 technology.
Overall, the methodological framework of CRISPR-Cas9 knock-in is a complex, yet systematic approach that highlights the necessary steps for successful implementation of gene editing. Understanding these methodologies provides a foundation for ongoing research and application in various fields.
Challenges in CRISPR-Cas9 Knock-In Applications
The application of CRISPR-Cas9 knock-in technology presents various challenges that must be thoughtfully addressed. Understanding these difficulties is crucial, as it aids researchers in refining their techniques and improving the overall efficacy of this powerful tool. Issues such as off-target effects, efficiency, delivery mechanisms, and ethical considerations all play a significant role in the implementation of knock-in strategies. Acknowledging these obstacles allows for more informed research and application.
Off-Target Effects
Off-target effects remain one of the most prominent challenges encountered in CRISPR-Cas9 knock-in applications. These occur when the CRISPR system inadvertently modifies genes other than the intended target. Such unintended modifications can potentially lead to adverse effects and complicate the interpretation of experimental results. Researchers have been working to enhance the specificity of the gRNAs to minimize these impacts. Several strategies, like rational design and high-throughput screening, have been employed to assess and improve the precision of gene editing protocols. Reducing off-target activities is fundamental to ensuring the reliability of CRISPR-Cas9 techniques.
Efficiency and Delivery Issues
Another key challenge in CRISPR-Cas9 knock-in applications revolves around the efficiency of gene editing and the delivery of CRISPR components to target cells. The success of a knock-in largely depends on how well the CRISPR machinery is introduced into the correct cells and how effectively it performs the editing. Various factors can affect this, including cell type, delivery method, and the design of the donor template. Common delivery methods, like lentiviral vectors and electroporation, each have their own advantages and limitations. For instance, while lentiviral vectors can integrate genes stably in dividing cells, they may not be suitable for non-dividing cells. Continuous optimization and innovation in delivery strategies are crucial for improving the overall efficiency of knock-in technology.
Ethical Considerations
Contemplating ethical considerations is essential when discussing CRISPR-Cas9 knock-in technology, especially in the context of gene editing in humans. The potential to alter the human genome raises significant concerns regarding safety, consent, and the implications for future generations. This technology has the power to eliminate genetic disorders but also carries risks of unintended consequences. Ethical discussions must address these risks and pave the way for responsible usage.
Gene Editing in Humans
Gene editing in humans represents a frontier filled with possibilities and concerns. The ability to correct genetic defects or modify traits can lead to advancements in personalized medicine and treatment options. However, the key characteristic of human gene editing lies in its potential to produce both benefits and unforeseen consequences. Opponents often highlight the risk of exacerbating social inequalities, as enhancements could be out of reach for many. This highlights the unique feature of gene editing in humans: it necessitates an ongoing, critical dialogue among scientists, ethicists, and policymakers.
Regulatory Frameworks
The establishment of regulatory frameworks is critical to ensuring that CRISPR-Cas9 technologies are applied responsibly. These frameworks govern the ethical use of gene editing and provide guidelines for researchers. A robust regulatory landscape encourages innovation while safeguarding public interests. The unique feature of regulatory frameworks is their adaptive nature, allowing for evolution based on new discoveries in genetic research. However, improper regulation can stifle advancements and limit the benefits of technology.
"Ethical considerations are paramount in the application of CRISPR-cas9 technology, guiding decisions that impact human health and society."
Addressing these challenges not only promotes safer applications but also reinforces trust between the public and the scientific community. Thus, ongoing efforts in refining techniques, enhancing delivery methods, and establishing ethical guidelines will shape the future of CRISPR-Cas9 knock-in applications.
Future Directions in CRISPR-Cas9 Research
The future of CRISPR-Cas9 technology has significant implications for a variety of fields including medicine, agriculture, and biotechnology. As the genomic editing landscape evolves, understanding these future directions becomes crucial not only for researchers but also for policymakers and the public. Innovations in this area promise enhanced precision and efficiency, paving the way for new therapeutic and agricultural solutions. This section will explore innovations, therapeutic potentials, and the broader implications of CRISPR-Cas9 expanding applications.
Innovations and Technological Advancements
Recent advancements in CRISPR-Cas9 technologies have paved the way for innovations that enhance the efficacy of gene editing. New tools such as CRISPR-Cas13, which targets RNA instead of DNA, show potential in treating diseases caused by RNA viruses. Furthermore, improvements in delivery mechanisms, such as lipid nanoparticles, have enhanced the delivery efficiency of genetic material into cells.
Recent years have also seen developments in base editing, which allows for precise editing of single bases. This offers a route to correct point mutations without introducing double-strand breaks. Another area of innovation is the creation of high-fidelity Cas9 proteins. These variants promise reduced off-target effects and increase the safety profile of CRISPR applications.
"Innovations in CRISPR technology will redefine the boundaries of genetic engineering, allowing for safer and more precise interventions."
Potential for Therapeutic Applications
The therapeutic applications of CRISPR-Cas9 technology are vast, particularly in the treatment of genetic disorders. Gene therapy approaches that utilize CRISPR-Cas9 aim to correct mutations responsible for diseases such as sickle cell anemia and cystic fibrosis. Clinical trials are currently underway, aiming to demonstrate the safety and effectiveness of these methods in humans.
Additionally, the use of CRISPR in developing therapies for cancers and other complex diseases is gaining traction. By systematically knocking in or out genes that regulate cellular processes, researchers can identify potential treatments that were previously elusive. These therapeutic avenues represent not just hope for patients but a transformative approach in modern medicine.
Research Toward Broader Applications
As research progresses, the applications of CRISPR-Cas9 technology extend beyond healthcare. In agriculture, the potential for creating crops with desirable traits continues to be a focal point. Genetic modifications using CRISPR could lead to crops more resilient to climate change, pests, and diseases.
Environmental applications also hold promise. CRISPR technology can potentially be used to engineer microorganisms for bioremediation, capable of breaking down pollutants or capturing carbon dioxide.
In summary, the future directions in CRISPR-Cas9 research stand to reshape various spheres of life. As innovations evolve and therapeutic avenues widen, monitoring ethical considerations will become increasingly essential. Therefore, careful discourse will be crucial to navigate the future landscape of genetic engineering.
Culmination
The exploration of CRISPR-Cas9 knock-in technology has revealed significant insights into its functionalities and potential applications. This technique represents a notable advancement in genetic engineering, allowing for precise modifications within the genome. The mechanisms of CRISPR-Cas9 contribute to its effectiveness, enabling targeted alterations that traditional methods cannot achieve with the same accuracy.
Summary of Findings
Throughout this article, several key points emerged:
- Mechanism of Action: The CRISPR-Cas9 system effectively identifies and modifies specific DNA sequences through its components—the guide RNA and Cas9 enzyme.
- Applications: This technology has broad implications across various fields, including gene therapy, agriculture, and basic research. Its ability to introduce desirable traits or correct genetic defects positions it as a pivotal tool in both medicine and biotechnology.
- Challenges: While promising, CRISPR-Cas9 faces hurdles, such as off-target effects and ethical concerns, particularly regarding its use in humans. Addressing these issues is critical as the technology evolves.
Final Thoughts on CRISPR-Cas9 Knock-In Technology