Deciphering ADP: Its Role and Applications in Science
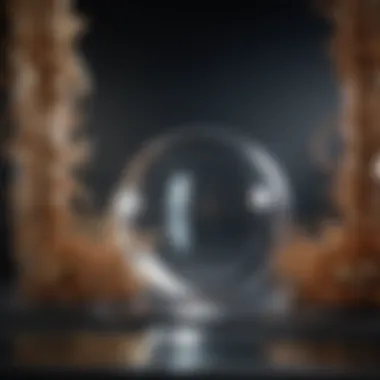
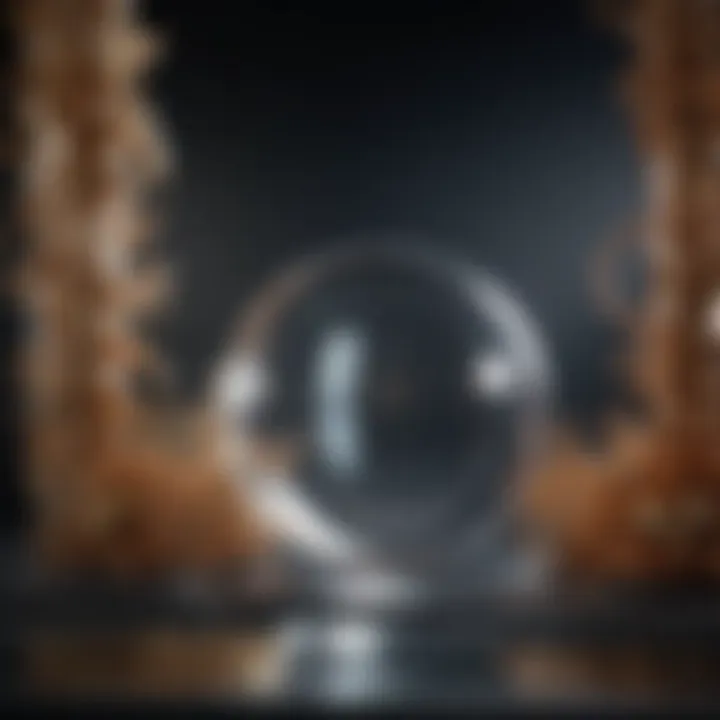
Intro
Adenosine diphosphate (ADP) is a crucial molecule in the biochemistry of living cells. It plays an essential role in the production and regulation of energy within various biological systems. Understanding the significance of ADP goes beyond just its biochemical reactions; it encompasses its vital applications in multiple scientific disciplines including molecular biology, physiology, and medicine. This exploration will shed light on ADP's mechanisms, its relationship to energy metabolism, and how these interactions manifest in practical settings such as research and clinical applications.
Research Highlights
Key Findings
ADP has several notable functions and implications in cellular processes. Here are some key points:
- Energy Transfer: ADP is fundamental in the energy transfer mechanisms of cells. It is converted to ATP (adenosine triphosphate), which is the primary energy carrier. This conversion is critical in aerobic respiration and various metabolic pathways.
- Role in Signaling: Recent research indicates that ADP plays a role in signaling pathways, especially in cellular communication and response mechanisms. It can influence processes like blood clotting and platelet aggregation.
- Enzyme Regulation: ADP is a key allosteric regulator of various enzymes, impacting their activity and metabolic flow. Its levels can indicate the energy status of a cell, signaling when metabolic adjustments are needed.
Implications and Applications
The knowledge surrounding ADP has led to various implications in scientific and clinical fields:
- Clinical Diagnostics: Measuring ADP levels can aid in diagnosing certain metabolic disorders. Abnormal ADP levels may indicate issues with energy metabolism.
- Drug Development: Understanding ADP's interactions has implications for pharmacology. Drugs targeting ADP signaling pathways could lead to new treatments for cardiovascular diseases and other conditions.
- Research in Cellular Bioenergetics: ADP is vital in studies focusing on cell metabolism, aging, and disease processes. Researching its functions deepens our understanding of cellular health and disease mechanisms.
Research into ADP highlights its multifaceted role in life sciences, emphasizing the interconnectedness of energy metabolism and cellular function.
Methodology Overview
Research Design
The investigation into ADP has utilized various research designs, from observational studies to experimental designs that assess its biochemical functions within controlled environments. Researchers often employ techniques such as spectrophotometry and chromatography to analyze ADP's roles and interactions.
Experimental Procedures
Experimental procedures for studying ADP may involve:
- In Vitro Comparisons: Using isolated enzymes or cell cultures to examine how ADP influences enzymatic activity or cellular responses.
- In Vivo Studies: Observing the physiological effects of ADP in animal models, providing insights into its broader implications in living organisms.
- Analytical Techniques: Utilizing methods such as NMR (Nuclear Magnetic Resonance) spectroscopy to explore ADP interactions at the molecular level.
In summary, understanding adenosine diphosphate is crucial in many biological contexts. Its roles in energy metabolism, signaling, and as a regulatory molecule form a foundation for various applications in research and clinical advancements.
Defining ADP
Understanding adenosine diphosphate (ADP) is crucial as it plays a significant role in various biological processes. This section aims to clarify what ADP is and describe its chemical structure. Both the definition and structure of ADP provide insight into its functionality and importance in cellular metabolism.
What is Adenosine Diphosphate?
Adenosine diphosphate (ADP) is a nucleotide, one of the building blocks of nucleic acids. It consists of two phosphate groups, a ribose sugar, and an adenine base. ADP is often described as a low-energy molecule that acts as a precursor to adenosine triphosphate (ATP).
In the context of energy metabolism, ADP is paramount. When energy is needed, ATP releases one of its phosphate groups, thus converting ATP to ADP. This process releases energy that the cell can harness for various metabolic functions, including muscle contraction and protein synthesis.
ADP levels inside the cell can indicate energy status. High levels of ADP might signal that the cell needs more energy. Thus, understanding ADP helps researchers explore how cells manage energy.
Chemical Structure of ADP
The chemical structure of ADP is essential for its function. It is made of three main components:
- Adenine Base: This nitrogenous base is vital for the molecule’s identity and its role in energy transfer.
- Ribose Sugar: The sugar connects the adenine base and the phosphate groups. It allows ADP to link energetically to other molecules.
- Phosphate Groups: These groups are the heart of ADP’s energy capacity. Two phosphate groups in ADP differ from three phosphate groups that are found in ATP.
Structurally, ADP is often represented in a linear or ball-and-stick model, showing the relationships between these parts. The convenient arrangement of these components facilitates the transfer of energy and enables biochemical reactions, both of which are integral to cellular metabolism.
"ADP is not just a byproduct of ATP breakdown; it is also a critical player in the nucleic energy cycles that sustain cell functions."
The understanding of ADP's role cannot be overstated. It bridges the immediate energy currency of ATP and the numerous reactions within the cell that require energy. Recognizing the significance and structure of ADP allows insight into complex metabolic pathways and cellular functions.
The Role of ADP in Cellular Metabolism
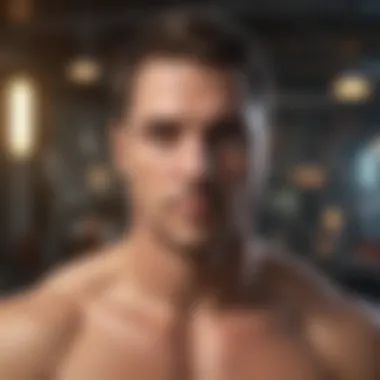
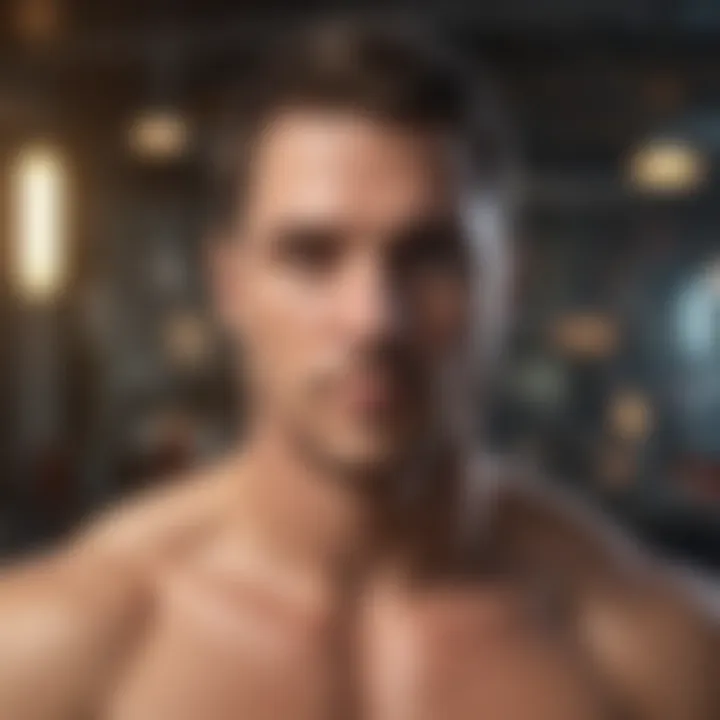
Cellular metabolism encompasses the vast array of biochemical reactions that allow organisms to grow, reproduce, maintain their structures, and respond to environmental changes. Within this intricate framework, adenosine diphosphate (ADP) emerges as a pivotal player. Understanding its role is crucial for students, researchers, educators, and professionals alike. The sheer significance of ADP in energy transfer and metabolic processes underlines its importance in various biological functions.
ADP as an Energy Carrier
ADP functions predominantly as an energy carrier in cells. Unlike its counterpart, ATP (adenosine triphosphate), which stores energy, ADP serves primarily as a conduit for energy exchange. When ATP is hydrolyzed to release energy, it converts into ADP and inorganic phosphate. This reaction is fundamental to many cellular activities, from muscle contraction to biochemical synthesis.
The transition from ATP to ADP illustrates how energy is utilized and conserved within different physiological pathways. Enzymes such as ATPases facilitate this process, ensuring that energy is available when needed.
Key Points:
- ADP is a critical component in the energy cycle of cells.
- It acts as an intermediate in the transfer and use of energy from ATP.
- The conversion is reversible, allowing cells to regenerate ATP through various metabolic processes.
Conversion Between ATP and ADP
The interconversion between ATP and ADP is crucial for maintaining cellular energy balance. When cells experience a high energy demand, ATP is consumed and converted to ADP. Subsequently, through cellular respiration and other metabolic pathways, ADP is phosphorylated back to ATP, replenishing the energy stores.
This cycle of conversion can happen through several processes:
- Substrate-level phosphorylation: This occurs directly in the metabolic pathway, such as glycolysis and the citric acid cycle, where ADP is phosphorylated using energy-rich substrates.
- Oxidative phosphorylation: This process takes place in mitochondria, where ADP is converted back to ATP using energy stored in the proton gradient created during electron transport.
Both processes highlight the seamless flow of energy that is characteristic of cellular metabolism, ensuring that cells have sufficient ATP to meet their energetic needs.
ADP's Role in Cellular Respiration
Cellular respiration is a fundamental process for energy production in aerobic organisms. ADP plays a critical role in this pathway, particularly during the oxidative phosphorylation phase. As glucose and other substrates undergo breakdown, the resulting energy is captured and used to synthesize ATP from ADP.
In essence, ADP acts as a substrate that, when phosphorylated, transforms back into ATP, thus participating actively in cellular energy transactions. This conversion underscores the dynamic nature of metabolism and the role of ADP as a key player in sustaining life.
"ADP is not just a byproduct; rather, it is a vital component in the energy conversion cycles that sustain cellular functions."
Understanding the role of ADP in metabolism illuminates its importance in both normal physiological functioning and in contexts of metabolic diseases. Recognizing how ADP integrates into larger pathways can help researchers identify potential therapeutic targets for enhancing metabolic efficiency or addressing energy-related disorders.
Biochemical Reactions Involving ADP
Understanding biochemical reactions involving adenosine diphosphate (ADP) is crucial to grasp its role in cellular metabolism and energy transfer. ADP is not merely a byproduct but a significant player in various biochemical pathways. Without ADP, numerous metabolic functions would be severely impaired. As a key intermediary in energy conservation and release processes, ADP's involvement in phosphorylation, glycolysis, and the citric acid cycle highlights its integral role in energy dynamics.
ADP and Phosphorylation
Phosphorylation refers to the addition of a phosphate group to molecules, significantly influencing their function. ADP takes on an essential role here as it can accept a phosphate group to become adenosine triphosphate (ATP). This conversion is critical because ATP serves as the primary energy currency of the cell. The mechanism of phosphorylation often occurs during cellular respiration, making ADP a key participant in energy metabolism.
Key points about ADP and phosphorylation include:
- Energy Transfer: Phosphorylation allows energy stored in nutrients to be transferred to ADP, forming ATP.
- Regulatory Function: The levels of ADP can signal a cell's energy status and regulate metabolic pathways accordingly.
- Enzyme Activity: Enzymes, such as creatine kinase, promote the conversion of ADP to ATP. The presence of ADP can stimulate or inhibit other enzymes, reflecting its regulatory significance in metabolism.
ADP in Glycolysis
Glycolysis is the first step in cellular respiration, converting glucose into pyruvate while generating ATP and NADH. In this process, ADP plays a role in harnessing energy. When glucose is broken down, energy release occurs, facilitating the phosphorylation of ADP, forming ATP.
Important points regarding ADP's role in glycolysis include:
- Energy Production: Four molecules of ATP can be produced per each glucose molecule, with ADP acting as a substrate in the process.
- NADH Generation: Alongside ATP, NADH is formed, further contributing to cellular respiration and energy availability.
- Energy Yield Regulation: ADP accumulation indicates high ATP consumption, leading to increased glycolytic activity to restore ATP levels.
The Role of ADP in the Citric Acid Cycle
The citric acid cycle, also known as the Krebs cycle, is central to aerobic respiration. It not only yields ATP but also produces key metabolites used for other biosynthetic processes. ADP is crucial in the transition from glycolysis to this cycle, facilitating further ATP production.
Here are some critical roles of ADP in the citric acid cycle:
- Substrate for ATP Production: Like in glycolysis, ADP is phosphorylated to create ATP at specific points within the cycle. This efficiency underscores ADP's importance in energy metabolism.
- Metabolic Intermediate: ADP assists in regulating the cycle by signaling the need for ATP during high energy demand.
- NADH and FADH₂ Production: The cycle also produces NADH and FADH₂, contributing to the electron transport chain, which ultimately leads to the production of more ATP.
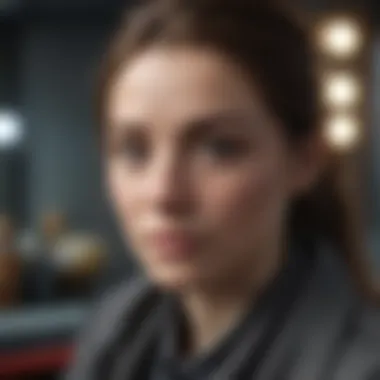
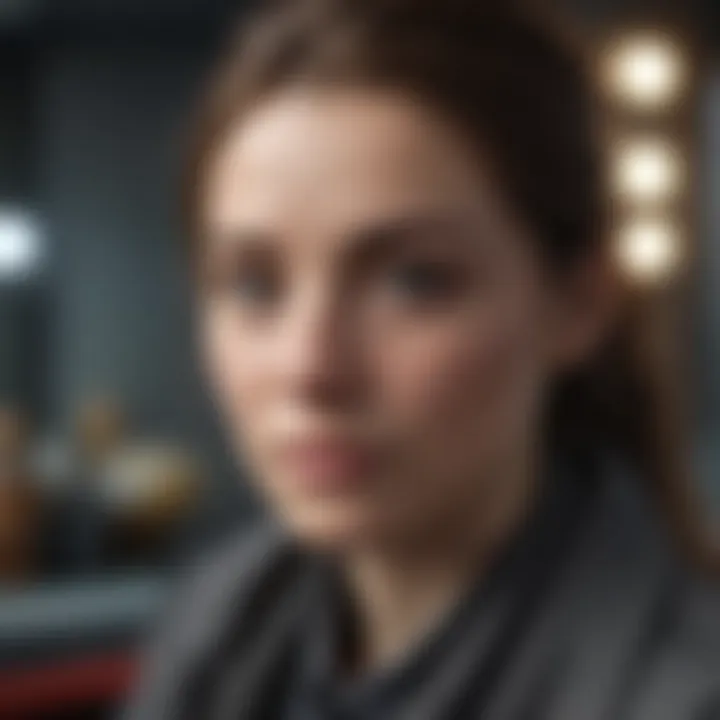
ADP's intricate connections in metabolic pathways emphasize its significance as more than a simple molecule; it is central to energy management in the cell.
These biochemical reactions involving ADP highlight its essential contributions to energy metabolism and signaling. Understanding ADP's roles prepares us for further discussions about its physiological significance.
Physiological Significance of ADP
The understanding of adenosine diphosphate (ADP) extends far beyond its basic chemical properties. ADP holds significant importance in various physiological processes. It plays a crucial role in energy transfer within cells and is pivotal in enhancing our knowledge of muscle physiology and hormonal interactions.
ADP levels in the body directly influence cellular metabolism. An increase in ADP concentration triggers pathways that enhance energy production. This aspect makes it a vital player in the regulation of metabolic processes. Its role as a signaling molecule becomes especially clear in muscle contraction, where it serves as a mediator for energy utilization and muscular efficiency.
Furthermore, the physiological implications of ADP are not limited to energy metabolism. ADP is also involved in hormonal regulation. Its interaction with hormones enhances our understanding of body dynamics and cellular responses. This relationship is critical in various biological contexts, including stress response and metabolic health, linking energy balance with hormonal activity.
"ADP serves as more than just an energy carrier; it also participates in complex signaling processes that regulate key physiological functions."
ADP in Muscle Contraction
ADP plays a key role in muscle contraction. When muscles contract, they utilize a molecule called adenosine triphosphate (ATP). During muscle contraction, ATP breaks down into ADP and inorganic phosphate. This process is vital for muscle fibers to generate force effectively. The conversion of ATP to ADP is an energy-releasing reaction, allowing the actin and myosin filaments in muscle cells to slide past each other and produce contraction.
The recycling of ADP back to ATP is equally critical. Muscle fibers require a constant supply of ATP to sustain contractions. When ADP levels are high, this indicates a need for more energy. Enzymes, such as creatine kinase, help recycle ADP into ATP, ensuring muscle function does not falter during prolonged activity. This process of converting ADP back into ATP underlines the importance of ADP in maintaining muscle performance during physical exertion.
ADP and Hormonal Regulation
Hormonal regulation is another area where ADP's significance is noteworthy. Different hormones can influence the levels of ADP in the body. For example, insulin can lead to increased glucose uptake in cells, which subsequently raises ATP levels and lowers ADP concentrations. In contrast, during states of energy deficit, such as fasting, ADP levels may rise, signaling the body to mobilize energy reserves.
Additionally, ADP interacts with receptor sites on the surface of various cells, playing a role in signal transduction. Its ability to influence pathways related to hormones further emphasizes its importance in the endocrine system. By modulating hormone response, ADP contributes to maintaining homeostasis in biological systems.
In summary, the physiological significance of ADP is multifaceted, encompassing muscle contraction, energy metabolism, and hormonal regulation. Understanding these roles provides insight into how our bodies manage energy and respond to various physiological demands.
ADP's Role in Biochemical Signaling
Adenosine diphosphate (ADP) serves as more than just a component in energy metabolism. It plays a critical part in biochemical signaling, which is vital for cellular communication and response. This signaling is crucial in various processes, including those related to cardiovascular health, immune responses, and even metabolic regulation. Understanding how ADP functions as a signaling molecule emphasizes its broader implications in both normal physiology and pathological states.
ADP as a Signaling Molecule
ADP is recognized as a signaling molecule that activates specific pathways within cells. It impacts various receptors, particularly purinergic receptors. These receptors respond to nucleotides such as ATP and ADP. When ADP binds to these receptors, it triggers a cascade of cellular events. This can include modulation of cellular responses such as differentiation, proliferation, and migration.
In platelets, for instance, ADP plays a notable role in hemostasis. It promotes platelet aggregation and activation. When platelets are activated, they release ADP which, in turn, recruits more platelets to the site of injury. This creates a rapid response to blood vessel damage, crucial for preventing excessive bleeding. The significance here lies in ADP’s ability to amplify and sustain the signaling necessary for efficient clot formation.
"ADP’s involvement in platelet activation exemplifies the intricate regulation of signals within the body. Its role goes beyond a mere energy state to a positioning in signaling events that hold cellular communication together.”
Effects on Platelet Activation
The effect of ADP on platelet activation is significant and multifaceted. When vascular injury occurs, exposed collagen activates platelets. The activated platelets then release ADP, which interacts with the P2Y1 and P2Y12 receptors on adjacent platelets. This interaction does not only enhance their activation but also leads to further aggregation.
- P2Y1 receptor: This receptor is responsible for mediating the initial shape change and aggregation of the platelets.
- P2Y12 receptor: This receptor plays a role in the amplification of the platelet response and is targeted by antiplatelet therapies like clopidogrel.
The sequence of events underscores the importance of ADP in the clotting process, providing a clear link between its biochemical properties and physiological outcomes. Thus, ADP functions as a pivotal mediator in platelet signaling pathways, impacting overall cardiovascular health.
In summary, ADP’s role in biochemical signaling is pivotal. Understanding its functions helps illuminate how cells communicate and respond to various stimuli, particularly in events like clot formation. By identifying pathways through which ADP operates, scientists can better understand its implications in diseases like thrombosis and other cardiovascular conditions. This research could lead to new therapeutic strategies targeting ADP signaling pathways.
Research Applications of ADP
Adenosine diphosphate (ADP) is not just a key player in cellular metabolism; it has significant applications in research across various scientific disciplines. Understanding ADP's multifaceted roles enhances our grasp of biological processes. This section highlights its importance in molecular biology and pharmaceutical research, two fields where ADP serves as an invaluable tool.
ADP in Molecular Biology Studies
In molecular biology, ADP is crucial for understanding energy dynamics within cells. Researchers often study ADP to gain insights into cellular metabolism. For instance, the conversion of ATP to ADP is a fundamental aspect of energy transfer. This process enables researchers to dissect energy usage in cell functions. By studying fluctuations in ADP levels, scientists can infer the activity of ATP-generating processes like oxidative phosphorylation.
Furthermore, ADP acts as a substrate in various enzymatic reactions. Kinases, for example, utilize ADP as a participant in phosphorylation processes. The study of these reactions helps elucidate signal transduction pathways. When researchers explore these pathways, they uncover how cells communicate and respond to internal and external stimuli.
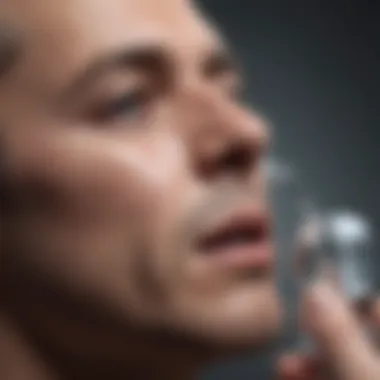
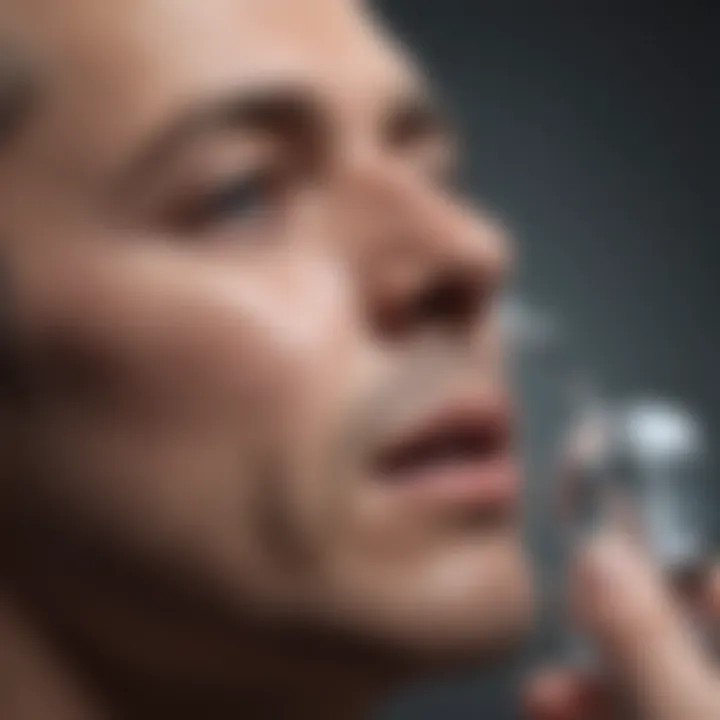
Understanding ADP’s behavior can lead to developments in targeted therapy, revealing how cellular energy dynamics are altered in diseases.
ADP is also utilized in constructing biosensors for detecting nucleotide presence. These biosensors employ changes in electronic properties upon ADP interaction, thus enhancing molecular detection methods. This technology is vital for diagnostics, allowing researchers to monitor cellular conditions effectively.
ADP in Pharmaceutical Research
The pharmaceutical sector also places great emphasis on ADP. Its role in thromboembolic disorders has spurred investigations into ADP receptor antagonists. These drugs, such as clopidogrel, are essential in managing cardiovascular diseases by inhibiting platelet aggregation. Understanding the pharmacodynamics of ADP allows for the design of new medications that target its pathways.
Moreover, ADP is involved in the mechanism of action for various drugs designed to treat metabolic disorders. Many clinical studies focus on how modulating ADP levels can influence overall metabolic health. By adjusting these levels, it's possible to develop therapies that promote energy balance in patients with conditions like diabetes.
In addition, research into ADP's role in signaling pathways is ongoing. The knowledge gained here can facilitate the creation of drugs that precisely manipulate cellular responses. As the understanding of these mechanisms grows, it opens doors to new therapeutic approaches.
In summary, the research applications of ADP in molecular biology and pharmaceutical studies indicate its vital role in advancing our understanding of various scientific fields. It is clear that as research evolves, so too will the roles and applications of this important molecule.
Clinical Implications of ADP
Understanding the clinical implications of adenosine diphosphate (ADP) is essential in both medical research and patient care. ADP's role in various physiological processes makes it a focal point for diagnostics and therapeutic approaches. This section will elucidate the important aspects regarding cardiovascular health and thrombotic disorders, highlighting their interrelation with ADP.
ADP and Cardiovascular Health
ADP plays a significant role in cardiovascular health. It is a crucial component in the regulation of platelet activation and aggregation. When blood vessels are damaged, ADP is released from activated platelets, signaling the need for hemostasis. This process helps to form a thrombus, which is essential for preventing excessive bleeding. However, an overactive ADP signaling pathway can lead to pathological conditions such as thrombosis.
Research indicates that elevated levels of ADP can contribute to a higher risk of cardiovascular events. For example, individuals with metabolic syndromes often exhibit altered ADP responses, which can result in increased platelet activity. This heightened aggregation can subsequently lead to conditions such as myocardial infarction or stroke.
Moreover, therapeutic strategies targeting ADP signaling pathways are seen as promising avenues for improving cardiovascular outcomes. Antiplatelet drugs like clopidogrel and ticagrelor work by inhibiting ADP receptors and thus preventing unnecessary platelet activation, ultimately reducing the risk of thromboembolic events.
In summary, understanding ADP's role allows clinicians to better assess cardiovascular risk and optimize therapeutic strategies for prevention.
Role in Thrombotic Disorders
Thrombotic disorders represent a significant medical concern, and ADP is central in their pathophysiology. In these disorders, the balance between coagulation and fibrinolysis is disrupted, leading to strong blood clots within the blood vessels. ADP contributes to this process by enhancing platelet aggregation through its receptors, primarily P2Y1 and P2Y12.
Conditions such as Deep Vein Thrombosis (DVT) and Pulmonary Embolism (PE) are influenced by increased ADP activity. In DVT, for example, ADP can exacerbate the condition by promoting clot stability. This leads to complications when segments of the thrombus break free and travel to the lungs, causing a pulmonary embolism.
Monitoring ADP levels can significantly impact clinical approaches to managing thrombotic disorders. Targeted therapies that modulate ADP signaling offer potential solutions to manage these conditions. Understanding individual patient responses to such therapeutics could lead to more personalized treatment strategies.
The Future of ADP Research
The ongoing research surrounding adenosine diphosphate (ADP) is essential, significantly shaping our understanding of biochemical processes and medical applications. The relevance of this topic lies not only in how ADP functions within cells but also in the broader implications for health and technology. As we advance, a few key elements become crucial in shaping the trajectory of ADP research.
First, understanding the mechanisms of ADP in various biological contexts can lead to more effective therapeutic strategies. For instance, the relationship between ADP and cardiovascular health is increasingly critical. As cardiovascular diseases remain one of the leading causes of mortality, deciphering how ADP influences blood clotting and vascular health could result in new treatments.
Second, leveraging technological advancements will enhance ADP analysis. Improved methods will allow for more precise measurements of ADP levels in various biological systems, providing better insight into its role in different conditions. This technological innovation is essential for developing personalized medicine approaches, where patient-specific biochemical profiles can inform targeted therapies.
Finally, the intersection of ADP research with other scientific domains illustrates its versatility and potential. Not only is ADP a focal point in biochemistry, but it also plays an integral role in molecular biology, genetics, and pharmacology.
"The future research on ADP could redefine our approach to treating various diseases, from metabolic disorders to heart conditions."
Emerging Technologies in ADP Analysis
Emerging technologies are revolutionizing how researchers study ADP. High-throughput screening methods are among the forefront innovations. These allow researchers to quickly analyze large datasets concerning ADP levels and its metabolic pathways. This efficiency can drastically reduce time spent on research while increasing the reliability of the data collected.
Additionally, advancements in mass spectrometry enable scientists to quantitatively analyze ADP and its metabolites in real-time. This offers insights into dynamic changes that occur in cellular environments. Facilitating such studies can lead to a greater understanding of the metabolic states of cells under varying conditions.
Other relevant technologies include biosensors that detect ADP levels with high sensitivity. Such devices can be used in both laboratory settings and clinical diagnostics. Accurate quantification of ADP in blood or tissue samples aids in monitoring conditions related to energy metabolism, thereby enhancing clinical decision-making.
Potential Therapeutic Developments
The future of ADP research holds considerable promise for therapeutic development. As scientists deepen their understanding of ADP's biochemical roles, new drug targets may emerge. Specific inhibitors or enhancers of ADP interactions could lead to innovative treatments for various disorders.
For example, in cardiovascular health, manipulating ADP function could provide novel antiplatelet therapies. By selectively targeting ADP receptors, researchers may develop drugs that prevent unwanted platelet aggregation without significantly affecting normal coagulation processes.
Moreover, as ADP's role in cellular signaling becomes clearer, potential treatments for metabolic disorders could be explored. Regulating ADP levels might help manage conditions like diabetes or obesity, where energy metabolism is disrupted.
In summary, future ADP research is not only about understanding its functions better but also about translating these insights into practical applications. The opportunities to improve health outcomes through targeted therapies and diagnostics are both exciting and vital for advancing healthcare.