Exploring the Deep Sea Ecosystem: Earth's Final Frontier
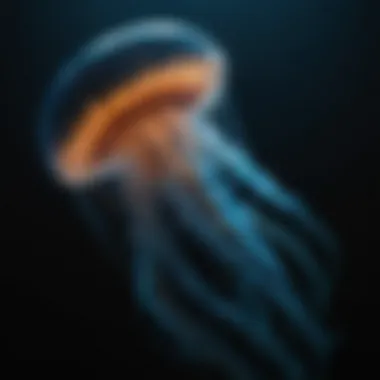
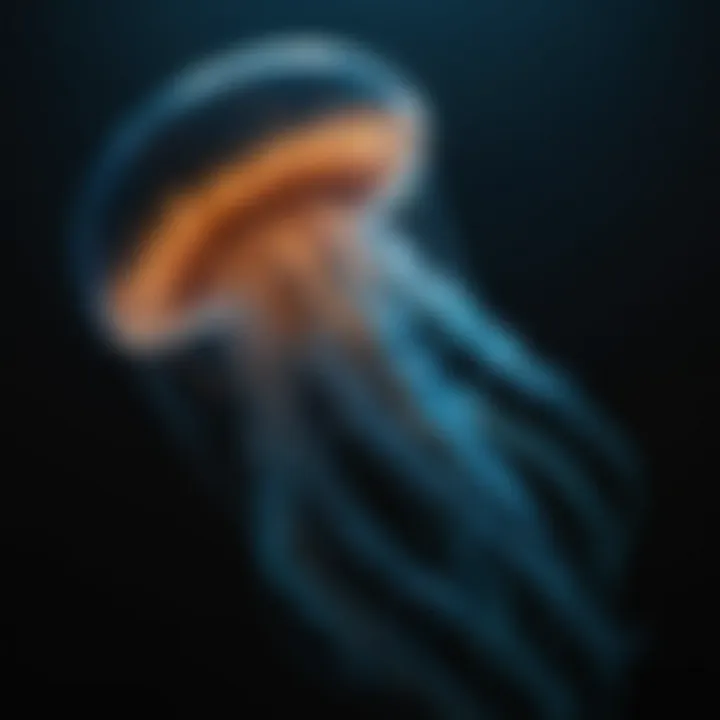
Intro
The deep sea ecosystem remains an area of profound intrigue and mystery. Situated beneath the sunlit surface waters, this ecosystem harbors a rich tapestry of life forms that have adapted to extreme conditions. Deep-sea habitats are characterized by high pressures, low temperatures, and complete darkness. This unique environment offers a window into the resilience of life on Earth, showcasing organisms that defy conventional biological limits.
Understanding the deep sea is critical not only for the knowledge it provides about biodiversity but also for its influence on global biogeochemical cycles. Deep-sea ecosystems play an essential role in regulating carbon storage, influencing climate patterns, and supporting a vast array of marine species. However, with increasing human activity, these ecosystems face numerous threats, leading to urgent calls for their protection and sustainable management.
With this overview, we aim to navigate through the essential aspects of deep-sea ecosystems, examining their structure, functions, and the myriad organisms that inhabit them. The aim is to shed light on the need for further exploration and conservation efforts, while also addressing the implications of human actions on these vital ecosystems.
Intro to the Deep Sea Ecosystem
The deep sea ecosystem is one of the most mysterious and least explored areas of our planet. It encompasses vast ocean depths that remain largely untouched by human activities. Understanding this ecosystem not only enhances our knowledge of marine biology but also reveals the interconnectedness of life on Earth. The deep sea plays a vital role in regulating global climate, providing vital resources, and supporting unique biodiversity.
Moreover, the deep sea serves as a reservoir for information regarding the history of the planet. By studying its features and organisms, scientists can gain insight into past climate conditions and biological evolution. Investing in research in this area has implications for environmental conservation, sustainable resource management, and better comprehension of natural processes.
In summary, examining the deep sea is not just about understanding its dense ecosystems. It involves recognizing the significance of deep-sea environments in broader ecological and geological contexts. This section sets the stage for an in-depth exploration of such topics, enhancing awareness about the importance of the deep sea and the pressing need to conduct further studies.
Defining the Deep Sea
The term "deep sea" generally refers to oceanic zones below the photic layer, primarily beyond 200 meters depth. While the epipelagic zone, which is the uppermost layer, accommodates light penetration and supports photosynthesis, the deep sea is characterized by complete darkness. It encompasses several unique zones, including the mesopelagic, bathypelagic, abyssopelagic, and hadopelagic regions.
Each of these zones has distinct environmental conditions such as temperature, pressure, and chemical makeup. For example, in the abyssal zone, which ranges from 3000 meters to about 6000 meters, temperatures hover around 2 degrees Celsius, and the pressure exceeds 200 times that of the surface. The hadopelagic zone, even deeper, features extreme conditions, making it a frontier for some of the most unusual forms of life.
Importance of Studying the Deep Sea
The exploration of the deep sea is critical for numerous reasons. Firstly, it contributes to our understanding of biodiversity at extreme depths. Organisms that dwell in the deep sea have adapted in remarkable ways to their surroundings. These adaptations include bioluminescence for communication and predation, extreme pressure tolerance, and unique metabolic processes that allow survival in nutrient-poor environments.
Secondly, the deep sea significantly impacts global biogeochemical cycles. It plays a crucial role in carbon storage, which is vital for regulating Earth's climate. For example, deep-sea organisms contribute to the sequestration of carbon dioxide through processes like the biological carbon pump. Additionally, understanding nutrient cycling in such complex environments aids the scientific community in addressing global issues such as climate change.
Lastly, recognizing human impacts on the deep sea is essential for conservation efforts. Activities such as deep-sea mining and pollution threaten ecosystems that are already vulnerable. By studying the deep sea, we can better advocate for protective measures and sustainable practices that safeguard these vital habitats for future generations.
Geological Features of the Deep Sea
The deep sea is not merely a vast waterbody; it encompasses unique geological features that are essential for understanding the ecosystem. This section addresses how these geological structures influence ocean dynamics, habitat availability, and the distribution of marine life. Studying these features can provide insight into the earth’s processes and the life forms adapting to extreme conditions. The geological features of the deep sea also play a vital role in global circulation patterns and biogeochemical cycles, making them significant beyond the depths of the ocean.
Continental Shelves and Slopes
Continental shelves are shallow areas extending from the land to the deep ocean. They cover about 7.5% of the ocean's area, yet they are among the most productive marine regions. These shelves provide habitat for many species. The slope is where the continental shelf transitions to the deep sea. This steep descent hosts unique formations such as canyons and submarine landslides.
The productivity of the continental shelves results from several factors. Shallower waters allow sunlight penetration, fostering photosynthesis. Nutrient runoff from land is common, supporting various marine life. Additionally, these areas are essential breeding grounds for many fish species.
In contrast, the continental slope features more extreme conditions. The slope increases pressure and decreases temperature, creating harsh living environments. Organisms in this area are uniquely adapted to survive these conditions. Their deep-sea habitats play a crucial role in overall ocean health, as many species rely on these areas for part of their life cycles.
Abyssal Plains
Abyssal plains represent some of the flattest and most featureless regions on Earth, typically found at depths between 3,000 and 6,000 meters. These plains cover about 50% of the Earth's surface. Sediments that accumulate here come from various sources such as terrestrial runoff, marine snow, and volcanic activity. The conditions are quite stable, with low temperatures and little disturbance, fostering a unique environment for life.
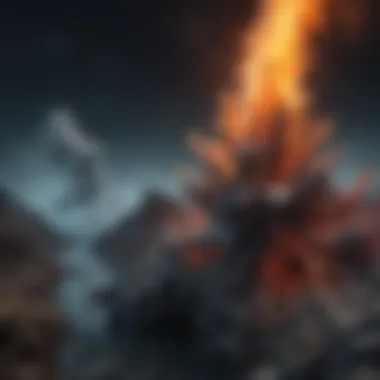
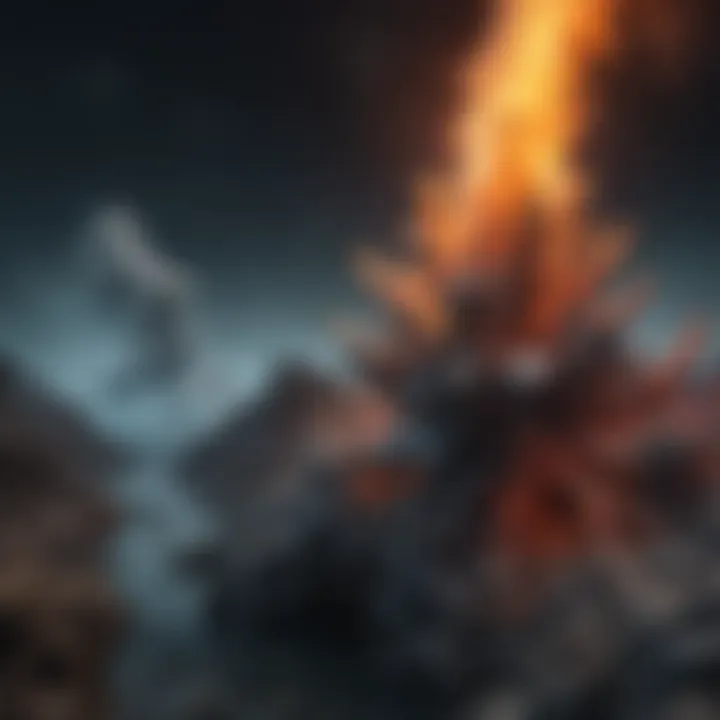
Life in the abyssal plains is sparse due to scarce food resources. However, organisms that thrive here have evolved fascinating adaptations. Many are scavengers, relying on the food that sinks from upper layers of the ocean. Some species have developed symbiotic relationships with microbes, utilizing the chemical energy produced by these bacteria. Thus, while the abyssal plains are often perceived as desolate, they support a range of unique and resilient life forms.
Mid-Ocean Ridges
Mid-ocean ridges are underwater mountain ranges formed by tectonic plate movements. These ridges are the longest mountain ranges in the world, stretching over 60,000 kilometers. They are crucial for the geological processes of our planet. The formation of new oceanic crust occurs at these ridges through volcanic activity.
Mid-ocean ridges also create unique habitats. Hydrothermal vents often found near these ridges emit mineral-rich water, supporting complex ecosystems. These vents provide energy sources for unique organisms that survive without sunlight. Chemosynthesis, a process whereby bacteria convert chemical energy from the vents into organic matter, sustains many species in these extreme environments.
"The interplay between geological features and marine life underlines the intricate connections that define the deep sea ecosystem."
Studying these characteristics can aid scientists in understanding oceanic ecology and the consequences of human activities.
Biodiversity of the Deep Sea
Biodiversity in the deep sea is a crucial topic for understanding the complex interactions and life forms thriving in one of the planet's last unexplored frontiers. The deep sea is not just a cold, dark place; it is a dynamic ecosystem full of unique organisms that have adapted to extreme conditions. The richness of species found here offers insights into evolutionary biology and ecological resilience. It is fundamental to recognize the importance of conserving this biodiversity, as many deep-sea creatures play significant roles in global ecological processes.
Unique Species and Their Adaptations
Inhabitants of the deep sea display remarkable adaptations that enable them to survive in such an inhospitable environment. For instance, the anglerfish uses a bioluminescent lure to attract prey in the pitch black waters. Other unique creatures include the giant squid and deep-sea jellyfish, both of which have adapted their bodies to withstand high pressure and cold temperatures.
These adaptations are vital for survival. Many species have developed specialized methods for food gathering, reproduction, and even communication. Some have evolved slow metabolisms to conserve energy in an environment where food is often scarce.
Microbial Life in the Abyss
Microbial life constitutes a significant part of the deep-sea ecosystem, supporting the overall biodiversity and functioning of these habitats. Various microorganisms, such as bacteria and archaea, are capable of surviving in extreme conditions such as high pressure, darkness, and low nutrient availability. These microbes play a critical role in nutrient cycling, breaking down organic materials and recycling nutrients that sustain larger life forms.
Through processes like chemosynthesis, these microbes can produce energy from inorganic substances, such as hydrogen sulfide, which is abundant near hydrothermal vents. This forms the basis of a food web that supports not only microbial life but also larger organisms, demonstrating the interconnectedness of the ecosystem.
Ecosystem Engineers
Certain species are recognized as ecosystem engineers, meaning they significantly modify habitats and create conditions conducive to other organisms. For example, the tube worm, which thrives near hydrothermal vents, provides a habitat for various marine species by creating structures that offer shelter.
Coral reefs found in the deep sea also serve as critical areas for biodiversity. They act as nurseries for many species and provide food and shelter for a multitude of marine organisms. Understanding these engineering roles is essential for conservation efforts, as the loss of such species can lead to a decline in overall ecosystem health.
Understanding the biodiversity and roles of different organisms in the deep sea is essential as it helps us predict how changes, such as climate change or human intervention, can impact these ecosystems.
Moreover, ongoing research efforts are vital to document biodiversity in the deep sea, fostering our understanding of ecological interactions that may have broader implications for global health.
Energy Sources in the Deep Sea
Energy sources in the deep sea play a critical role in sustaining the unique ecosystems found at extreme depths. Unlike terrestrial environments, sunlight does not penetrate these waters, making traditional photosynthesis impossible. Instead, deep-sea organisms rely on alternative methods to derive energy. This understanding is vital for comprehending the intricate food webs and biological processes that govern deep-sea life. Not only do these energy sources support various organisms, but they also contribute to broader ecological and biogeochemical cycles.
Chemosynthesis vs. Photosynthesis
Chemosynthesis is often contrasted with photosynthesis, as it represents a unique energy acquisition method. While photosynthesis relies on sunlight to convert carbon dioxide and water into glucose and oxygen, chemosynthesis utilizes chemical reactions, specifically involving sulfur or methane compounds, to create organic matter. This process is primarily carried out by microorganisms in extreme environments, such as hydrothermal vents. Organisms that depend on chemosynthesis form the base of the food chain in these deep-sea habitats.
Key characteristics of chemosynthesis include:
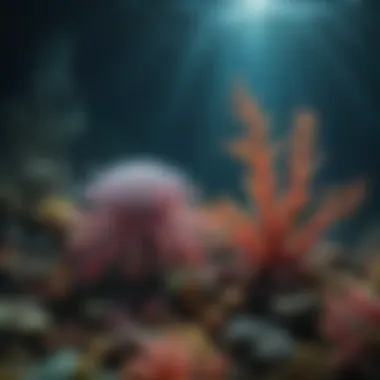
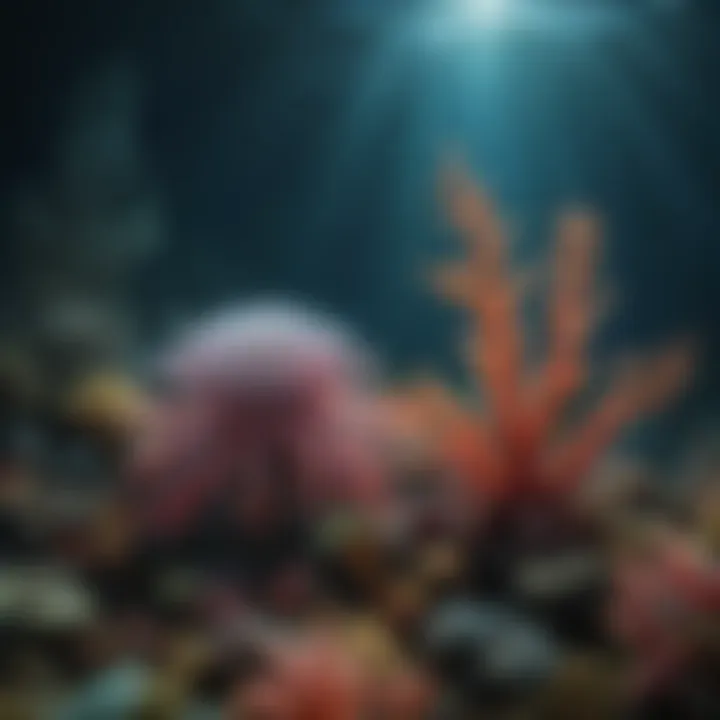
- Energy source: Uses chemical energy from inorganic molecules.
- Primary producers: Bacteria and archaea are the main organisms, unlike plants in photosynthesis.
- Habitat: Mainly found in environments lacking light, such as deep sea.
This shift from photosynthesis to chemosynthesis allows for a unique and rich biodiversity to thrive in areas devoid of sunlight. The impact is significant, as it showcases the adaptability of life and its ability to harness various energy sources in different environments.
Hydrothermal Vents and Cold Seeps
Hydrothermal vents and cold seeps are critical locations in the deep sea where energy sources dramatically influence the ecosystem.
Hydrothermal vents are areas on the ocean floor where heated water rich in minerals is expelled from beneath the Earth's crust. These vents provide extremely high biodiversity hotspots due to the unique habitats they create. Microbial communities flourish here, forming the foundation of the food web. Organisms such as tube worms, clams, and various crustaceans thrive in this environment, relying on chemosynthetic bacteria to convert hydrogen sulfide into energy.
Cold seeps are another form of energy source in deep waters. They are areas where hydrocarbons seep into the ocean floor. Bacterial communities here also perform chemosynthesis but utilize methane or other hydrocarbons. Both hydrothermal vents and cold seeps offer unique conditions for life, resulting in significant biological diversity.
"These ecosystems showcase the resilience and adaptability of life, thriving under conditions that seem inhospitable to many other forms of existence."
The Role of Deep Sea Ecosystems in Global Cycles
Deep sea ecosystems play a critical role in maintaining the overall health of the planet's marine environment and its global biochemical cycles. Understanding their functions and interactions helps appreciate their significance in climate dynamics. The deep ocean acts as a massive carbon sink, storing vast amounts of carbon dioxide and influencing climate patterns. Furthermore, these ecosystems are essential for nutrient distribution, supporting marine life and sustaining food webs. They enable various chemical reactions that are vital for the balance of marine ecosystems and, by extension, terrestrial ecosystems.
Carbon Cycling and Storage
The deep sea significantly contributes to carbon cycling through processes that sequester carbon away from the atmosphere. One of the primary mechanisms is the biological carbon pump. This process begins when photosynthetic organisms, such as phytoplankton, absorb carbon dioxide during their growth. When these organisms die, their remains sink to the ocean floor, carrying carbon with them. This deep-sea carbon storage is crucial as it helps mitigate climate change by reducing atmospheric CO2 levels.
The sedimentation of organic material results in long-term carbon storage. Studies suggest that up to 30% of the carbon captured by phytoplankton is deposited in the deep sea. Various factors influence how much carbon is sequestered, including temperature, depth, and the presence of specific organisms. The resilience of these carbon stores is vital because disturbances such as climate change and ocean acidification can release stored carbon back into the atmosphere, further exacerbating global warming.
Nutrient Recycling
Nutrient recycling in the deep sea is essential for sustaining marine productivity. Deep ocean waters are rich in nutrients like nitrogen and phosphorus that are crucial for the growth of marine organisms. When organisms die and decompose, these nutrients are released back into the water column through microbial activity. This recycling process supports a diverse range of life forms, including those that inhabit the food webs above.
The deep sea's unique conditions, such as high pressure and low temperature, promote specialized microbial communities capable of breaking down complex organic materials. These microbes play a key role in transforming nutrients into forms that can be utilized by other marine organisms. As a result, deep-sea ecosystems are integral in maintaining nutrient availability and thus supporting life in the surface waters.
"Nutrient recycling is a silent process that plays a prominent role in the health of marine ecosystems and ultimately affects global food security."
Deep ocean ecosystems, through their role in carbon cycling and nutrient recycling, are indispensable to the maintenance of global cycles that support both marine and terrestrial life. Awareness of these systems is crucial for conservation efforts as human activities pose significant threats to their integrity.
Human Impacts on Deep Sea Ecosystems
Understanding human impacts on deep sea ecosystems is crucial, as these environments are delicate and resilient yet vulnerable to the activities of humankind. The actions undertaken on land and the surface of the ocean can deeply affect the intricate balances existing in the deep ocean. Knowledge of these impacts reinforces the need for effective management and conservation strategies.
Deep Sea Mining
Deep sea mining has become a significant concern in recent years. It involves the extraction of mineral resources from the ocean floor. These resources include valuable metals such as gold, silver, and copper, as well as polymetallic nodules and sulfide deposits. The process is conducted using various techniques, from remote-operated vehicles to massive dredging machines.
The mining process disrupts ecosystems that have evolved over millions of years. Coral reefs and deep-sea habitats often suffer irreparable damage from sediment plumes, habitat destruction, and the introduction of toxic substances into the surrounding water. Not only does this damage the organisms living in the area, but it also affects species that depend on these ecosystems for food and shelter.
Governments and organizations are starting to consider regulations in an effort to mitigate these impacts. However, the balance between economic interests and environmental protection remains tenuous. The long-term consequences of deep sea mining are not fully understood, which further complicates decision-making processes.
Pollution and Climate Change
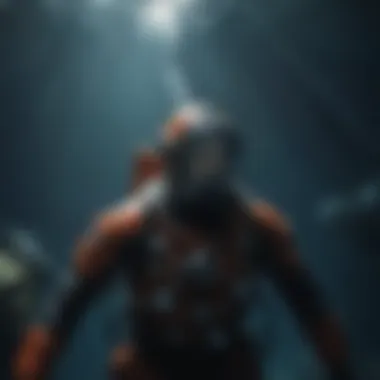
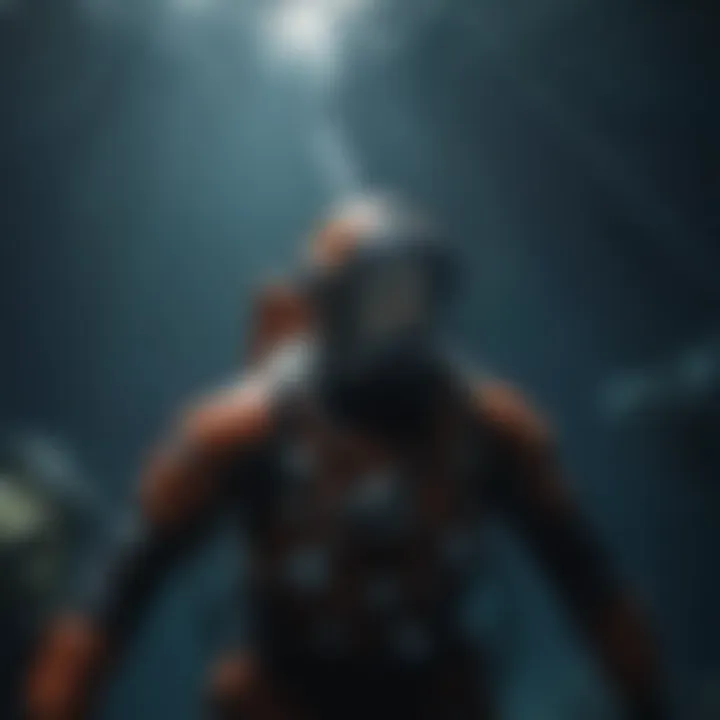
Pollution poses another severe threat to deep sea ecosystems. Plastics, heavy metals, and other contaminants find their way into deep-sea habitats, adversely impacting marine life. Marine organisms may ingest or become entangled in plastics, leading to significant health issues, such as starvation or suffocation.
Climate change compounds these problems. As ocean temperatures rise, the chemistry of the sea changes, affecting the solubility of gases and nutrients. Increased CO2 levels can lead to ocean acidification, which threatens calcifying organisms, such as deep-sea corals and shellfish. The warming ocean also disrupts fish migratory patterns and breeding cycles.
Research indicates that the overall ecosystem functioning can decline due to these combined factors, resulting in diminished biodiversity.
Conservation Efforts and Challenges
Conservation efforts are crucial for the deep sea ecosystems due to their immense biodiversity and the growing threats they face from human activities. Understanding these challenges enables us to formulate effective strategies to protect these fragile habitats. Each step taken to conserve deep sea environments can have widespread benefits for marine life and global ecological balance.
Protective Legislation
Protective legislation serves as a bedrock for the conservation of deep sea ecosystems. Various national and international laws seek to regulate activities that could potentially harm these environments. The United Nations Convention on the Law of the Sea (UNCLOS) is an essential framework that governs the usage of ocean resources. It sets guidelines to prevent pollution, protect marine species, and manage fishing practices.
Additionally, countries are increasingly enacting national laws to safeguard their marine territories. For example, the Marine Protection, Research, and Sanctuaries Act in the United States provides mechanisms for designating marine protected areas (MPAs). These areas prohibit destructive activities, allowing ecosystems to recover and flourish.
Moreover, international agreements, such as the Convention on Biological Diversity, encourage countries to protect marine biodiversity and establish conservation measures. Overall, effective legislation can significantly mitigate threats like overfishing, seabed mining, and pollution.
International Collaboration Initiatives
International collaboration initiatives are vital for addressing the challenges facing deep sea ecosystems. Given the transboundary nature of marine environments, countries must work together to tackle issues like climate change and deep sea mining. Collaborative efforts, such as the Global Ocean Alliance, aim to promote ocean health globally by sharing best practices and establishing targets for marine conservation.
Other initiatives include the Deep Sea Conservation Coalition, which focuses on protecting deep-sea biodiversity through advocacy and policy development. They work towards creating a moratorium on deep sea mining, which poses significant risks to vulnerable habitats and species.
Additionally, researchers from different countries often engage in joint expeditions to study deep sea ecosystems. This collaborative science enhances understanding and paves the way for informed conservation strategies.
"The preservation of marine biodiversity is no longer a choice. It is an obligation to our planet and humanity."
Implementing effective strategies is a collective responsibility that demands engagement from governments, scientists, and the public.
The Future of Deep Sea Research
The deep sea remains one of the least explored parts of our planet. Research efforts have lagged behind other scientific domains mainly due to the technical challenges posed by extreme conditions. However, the future of deep sea research holds promise, particularly as emerging technologies and innovative methodologies are developed. These advancements can enhance our understanding of deep-sea ecosystems, drive conservation efforts, and unravel the mysteries surrounding the organisms that inhabit these depths.
Emerging Technologies
Advancements in technology will shape the future of deep sea exploration significantly. Underwater drones, such as those developed by Ocean Infinity and Bluefin Robotics, allow scientists to explore areas that were previously inaccessible. These autonomous vehicles can conduct extensive mapping and sampling of the seafloor. Robotic technology has improved dramatically, enabling high-resolution imaging and data collection.
Moreover, sensors that measure various environmental parameters—like temperature, salinity, and pressure—are becoming more sophisticated. These devices can provide crucial information about climate change impacts on ocean systems. Also, advances in machine learning and artificial intelligence empower researchers to analyze vast datasets efficiently, leading to insights that supersede traditional research methods.
- Enhanced data collection tools
- Autonomous underwater vehicles
- Improved imaging techniques
- AI and machine learning for data analysis
In addition, interdisciplinary collaboration is vital. Scientists, engineers, and policymakers must work together to forge new paths in exploration. Thus, the future of deep sea research will favor technology that transcends the limitations of past methods.
Potential Discoveries and Insights
As research expands, the probability of discovering new species increases. The deep sea remains largely uncharted, and each expedition can yield organisms previously unknown to science. Some species might harbor unique biochemical properties useful in medicine or biotechnology. For instance, chemicals derived from deep-sea organisms have the potential to lead to breakthroughs in pharmaceuticals.
"The ocean is a gateway to understanding life beyond our planet, and it reshapes our understanding of biology, ecology, and evolution."
Furthermore, studying the deep sea can provide insights into Earth's history. Sediment layers serve as records of past climate conditions and can elucidate how ecosystems adapt or fail under changing climates. The potential understanding gained could inform better management practices for both marine and terrestrial environments.
However, to tap into these possibilities, researchers must prioritize sustainable practices in their explorations. Ensuring minimal impact on ecosystems is essential. By fostering a coexistence approach, the insights gained from the deep sea can contribute not only to science but also to global conservation efforts.