Understanding DNA Polymerase's Role in PCR
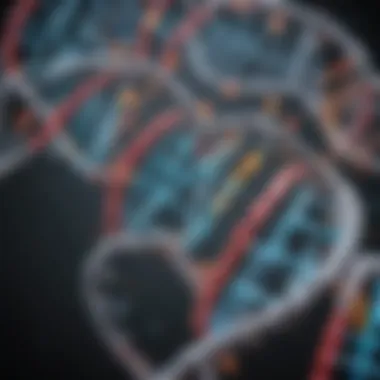
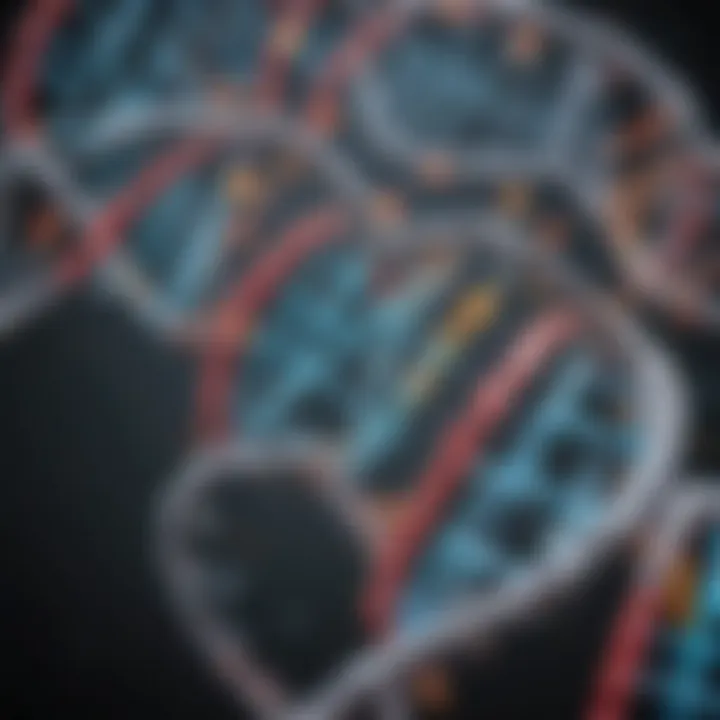
Intro
In the landscape of molecular biology, DNA amplification is a critical technique. Central to this process is DNA polymerase, an enzyme that synthesizes new DNA strands by adding nucleotides to a growing chain, utilizing a template strand. The polymerase chain reaction (PCR) has revolutionized many areas of research, diagnostics, and forensics. Understanding the intricacies of DNA polymerase is essential for mastering PCR applications.
This article investigates the mechanisms underpinning DNA polymerase performance in PCR, including enzyme selection and practical implications of fidelity and efficiency. With implications for a range of scientific fields, from genetics to biotechnology, the insights provided will assist researchers and educators alike in applying this knowledge effectively.
Research Highlights
Key Findings
- Mechanism of Action: DNA polymerase operates through a multi-step process. It binds to the single-stranded DNA during the annealing phase of PCR and catalyzes the addition of complementary nucleotides.
- Types of DNA Polymerases: Varieties exist, such as Taq polymerase, which is widely used due to its heat stability and robust performance at high temperatures.
- Enzyme Fidelity: The accuracy of DNA polymerase in synthesizing new DNA strands is crucial to ensure the reliability of amplification results. High-fidelity enzymes are often recommended for applications requiring precise DNA replication.
- Practical Applications: Beyond basic research, applications of DNA polymerase span medical diagnostics, criminal forensics, and genetic engineering, highlighting its versatile role in science.
Implications and Applications
- In Research: With accurate DNA amplification, researchers can explore genetic variations, study gene function, and investigate complex biological mechanisms.
- In Medicine: PCR plays a vital role in detecting pathogens, diagnosing diseases, and developing personalized medicine strategies.
- In Forensics: The specificity and sensitivity of PCR allow for the analysis of minute quantities of DNA, vital for solving criminal cases.
"The advancement of PCR technology has been pivotal in transforming scientific exploration and applications across various domains."
Methodology Overview
Research Design
The design of experiments involving DNA polymerase includes understanding temperature cycling—denaturation, annealing, and extension phases. Each phase is integral to achieving successful amplification, relying on precise coordination of these conditions.
Experimental Procedures
- Sample Preparation: Collect and purify DNA samples suitable for PCR amplification.
- Reagent Preparation: Optimal concentrations of DNA polymerase, primers, nucleotides, and buffer solutions are crucial.
- Thermal Cycling: Rigorous adherence to thermal cycling protocols is necessary to achieve notable amplification.
- Analysis: Utilizing methods such as gel electrophoresis to assess results post-amplification helps confirm the efficacy and quality of the PCR.
This article sets to provide a detailed understanding of the role of DNA polymerase in PCR, facilitating deeper insights relevant to the scientific community.
Intro to PCR
The introduction of polymerase chain reaction (PCR) has revolutionized molecular biology by providing a rapid method for amplifying specific DNA sequences. Understanding PCR is crucial for anyone involved in genetics, diagnostics, or forensic science. This section explores the essence of PCR, detailing its definition and significance, as well as tracing its historical development that has paved the way for modern applications in research.
Definition and Importance of PCR
Polymerase chain reaction, or PCR, is a biochemical technique utilized to exponentially amplify specific segments of DNA. This process involves repeated cycles of denaturation, annealing, and extension, allowing for the meticulous replication of targeted sequences. The importance of PCR cannot be overstated; it serves as the foundation for numerous applications in research, medicine, and forensic science.
PCR enables the identification of genetic material, allowing for the detection of pathogens, genetic disorders, and even the analysis of ancient DNA. For researchers, PCR provides a means to generate large quantities of DNA from minimal samples, facilitating various experiments and analyses. In clinical diagnostics, it plays a crucial role in identifying infections or genetic mutations, thereby informing treatment strategies. Furthermore, in forensic contexts, PCR has become an indispensable tool for analyzing minute samples, helping to uncover evidence that can support legal investigations.
Thus, the significance of PCR extends far beyond basic biology, having profound implications across multiple disciplines.
Historical Background and Development
The development of PCR dates back to the 1980s, with its invention attributed to Kary Mullis. His pioneering work introduced a method that harnessed the natural properties of DNA polymerases to amplify DNA efficiently. In 1985, Mullis applied heat to separate DNA strands, which allowed for the incorporation of primers designed to target specific sequences. The innovation of thermocycling, an automated process of alternating temperature cycles, marked a significant advancement, enabling controlled and repeated reactions without manual intervention.
The original PCR method has undergone numerous refinements since its inception. The introduction of thermostable DNA polymerases, such as Taq polymerase, sourced from Thermus aquaticus, drastically improved the efficiency and reliability of the PCR. This stability allows the enzyme to withstand the high-temperature denaturation phase, a key element in the PCR process. Over the years, research has led to the development of various optimized DNA polymerases, catering to different applications and enhancing fidelity and yield.
Today, PCR is a mainstay of molecular biology, with numerous adaptations, including quantitative PCR (qPCR) and reverse transcription PCR (RT-PCR), further pushing the boundaries of its capabilities. The historical context of PCR underscores its transformative impact on scientific research and its ongoing evolution in response to emerging challenges.
Understanding DNA Polymerase
Understanding DNA polymerase is crucial for grasping the intricacies of PCR technology and its applications. DNA polymerases are enzymes that synthesize DNA molecules from nucleotides, the building blocks of DNA. Their role in PCR cannot be overstated, as they are directly responsible for the amplification of DNA, a process that is central to numerous fields, including molecular biology, genetic research, and clinical diagnostics.
Due to their function, knowing about the types of DNA polymerases, their properties, and their specific functions can shape the results of PCR experiments significantly. The efficiency, fidelity, and longevity of the PCR process hinge on the performance of the chosen DNA polymerase. Furthermore, a solid understanding of DNA polymerases helps researchers select the most suitable enzyme for their applications, whether that be enhancing the accuracy of gene cloning or optimizing amplification for forensic analysis.
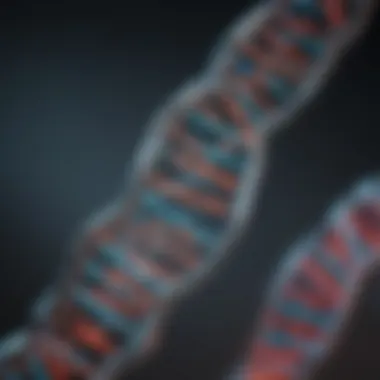
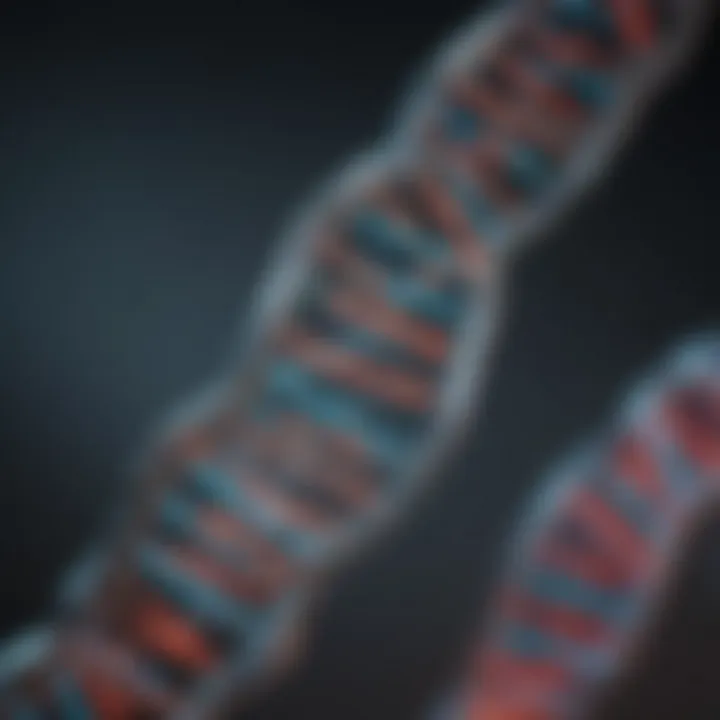
Definition and Functions of DNA Polymerase
DNA polymerase refers to a group of enzymes that facilitate the synthesis of DNA during biological replication. They operate by adding nucleotides one at a time to the growing DNA strand, following a template strand. This addition occurs in a five-to-three direction, making DNA polymerase essential for copying DNA sequences.
The primary functions of DNA polymerases include:
- Synthesis of New DNA Strands: They incorporate nucleotides, creating a complementary strand to the existing template.
- Proofreading Function: Some DNA polymerases have exonuclease activity which allows them to correct mistakes in DNA synthesis, thus enhancing the fidelity of DNA replication.
- Role in Repair Mechanisms: Beyond PCR, DNA polymerases are involved in repairing damaged DNA, which is essential for maintaining genetic integrity.
Types of DNA Polymerases
DNA polymerases can be classified into two main categories: classical DNA polymerases and specialized DNA polymerases. Each type serves distinct functions and is chosen based on specific requirements in research and diagnostic applications.
Classical DNA Polymerases
Classical DNA polymerases, such as Taq polymerase, are widely used in PCR due to their robustness. A notable characteristic of classical DNA polymerases is their high-temperature tolerance, which is critical for the denaturation step of PCR.
The contribution of classical DNA polymerases to PCR lies in their ability to withstand the extreme temperatures required to unwind double-stranded DNA. This property is what makes them a popular choice for many PCR protocols. Another key feature is their relatively high processivity, allowing them to add many nucleotides before falling off the DNA template.
Some advantages of classical DNA polymerases include:
- High efficiency in synthesizing DNA.
- Broad applicability in various types of PCR.
However, they also have limitations, such as higher error rates compared to some specialized polymerases, which can affect the overall fidelity of amplified products.
Specialized DNA Polymerases
Specialized DNA polymerases, including high-fidelity enzymes like Phusion polymerase, are designed for specific tasks that require greater precision. One of their defining features is the ability to achieve very accurate DNA synthesis, resulting in fewer errors during amplification.
These polymerases typically exhibit higher fidelity than classical enzymes, making them preferred for applications where precise DNA replication is necessary. Additionally, many specialized DNA polymerases can be engineered to work under specific conditions, enhancing their utility in various research contexts.
Advantages of specialized DNA polymerases include:
- Lower error rates, improving the quality of the amplified DNA.
- Ability to work with challenging templates, such as GC-rich or complex regions.
Nonetheless, they may have disadvantages, such as higher costs and potentially more complex reaction conditions. Understanding these differences is vital for choosing the right polymerase based on the goals of the experiment.
The selection of the appropriate DNA polymerase is critical in determining the success and accuracy of PCR results, influencing downstream applications and research outcomes.
The PCR Process
The polymerase chain reaction (PCR) is a cornerstone of molecular biology. Understanding The PCR Process is crucial as it lays out the steps in which DNA is amplified. This amplification is essential for various applications, from genetic research to forensic science. The process allows scientists to create billions of copies of a specific DNA segment, making it easier to analyze, manipulate, and utilize that DNA. The efficiency and accuracy of PCR directly hinge on the steps involved, highlighting the significance of DNA polymerase, the enzyme that plays a pivotal role in this amplification.
Steps Involved in PCR
The PCR technique is divided into three essential steps: Denaturation, Annealing, and Extension. Each step is critical in ensuring successful DNA amplification. Understanding each phase is fundamental for anyone aiming to use PCR in research or clinical settings.
Denaturation
Denaturation is the first step in the PCR process. During this phase, the double-stranded DNA template is heated, typically to around 94-98 degrees Celsius. This heat causes the hydrogen bonds between the nucleotide pairs to break, effectively 'unzipping' the DNA strands. This process is necessary because it generates single-stranded DNA, which is crucial for the subsequent annealing. The key characteristic of denaturation is its high temperature, enabling the rapid separation of DNA strands. It is beneficial because it initiates the entire amplification process. A notable feature of this step is its ability to quickly prepare the DNA for the following stages, although extreme heating can increase risks of damage to the sample.
Annealing
Following denaturation, the temperature is lowered to between 50-65 degrees Celsius for the annealing phase. During this phase, short sequences of DNA known as primers bind or anneal to the single-stranded DNA templates. Primers are essential as they provide a starting point for DNA synthesis. The key characteristic of annealing is its specific temperature range, which is vital for the precise binding of primers. This step is popular because correct annealing ensures that the resulting DNA strands are accurate copies of the desired sequences. A unique feature of this step is its reliance on temperature specificity. If the temperature is too low, incorrect binding can occur, leading to non-specific amplification. Conversely, if it’s too high, the primers might not bind adequately.
Extension
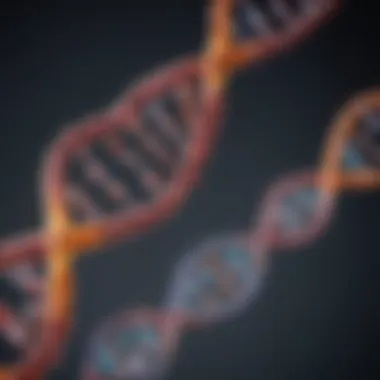
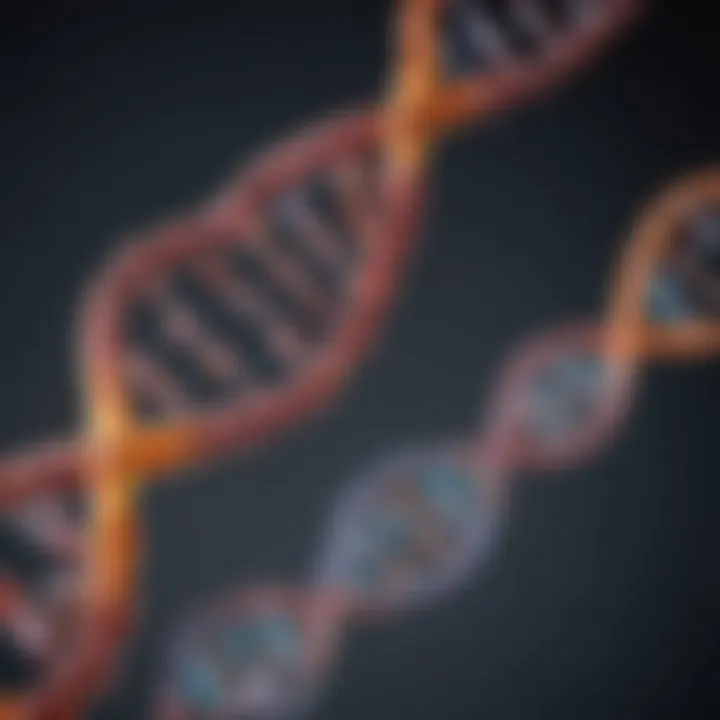
The final stage is the extension step, where the temperature is raised to about 72 degrees Celsius, optimal for DNA polymerase activity. This enzyme synthesizes new DNA strands by adding nucleotides to the primer-template junction. The key characteristic of extension is its dependence on the enzyme’s function, making it crucial for synthesizing the new strands. The process is advantageous because it exponentially increases the amount of DNA, as each cycle effectively doubles the number of DNA copies. A unique aspect of this step is the time taken, typically around 1 minute per 1,000 base pairs of DNA, though this may vary with different polymerases. The efficiency of this stage has direct implications for the overall yield of the PCR process.
Role of DNA Polymerase in PCR
DNA polymerase serves as the engine behind the PCR process. Its function encompasses the synthesis of new DNA strands during the extension phase. The choice of polymerase can greatly influence the success of PCR due to variations in their fidelity and efficiency. For instance, Taq DNA Polymerase, derived from the thermophilic bacterium Thermus aquaticus, withstands the high temperatures of denaturation. This robustness is indispensable for effective PCR, allowing researchers to amplify DNA with greater reliability. Furthermore, the activity of DNA polymerase is critical at each stage of the PCR process, from primer extension to ensuring accurate replication of the target DNA. Without the specificity and efficiency provided by DNA polymerase, the PCR would not achieve the desired outcomes in terms of quantity or quality of DNA amplification.
Selection Criteria for DNA Polymerase
Selecting the appropriate DNA polymerase is critical for the successful execution of PCR. The performance of the polymerase directly influences the efficiency, specificity, and yield of the amplification process. A careful assessment of various selection criteria ensures not only optimal results but also boosts reproducibility across experiments.
Fidelity of DNA Polymerases
Fidelity refers to the accuracy with which DNA polymerases replicate DNA. High-fidelity polymerases maintain low error rates, which is essential in applications like cloning and sequencing where precision is paramount. Errors introduced during DNA synthesis can lead to significant downstream issues, including incorrect gene expression and altered phenotypes. Moreover, many high-fidelity polymerases have proofreading activity that allows them to recognize and correct mismatches. This capability significantly enhances the reliability of results, making these enzymes preferable for critical applications.
Factors affecting fidelity include enzyme structure, concentration, and the temperature of the reaction. Typically, Taq polymerase is known for its speed, but a trade-off exists as it has a higher error rate. Thus, in applications requiring high fidelity, alternatives such as Phusion DNA Polymerase or Q5 Polymerase may be favored.
Processivity and Efficiency
Processivity defines how many nucleotides a DNA polymerase can incorporate before detaching from the DNA strand. High processivity is advantageous because it allows faster and more efficient DNA synthesis. Enzymes like Klenow Fragment and Taq polymerase can incorporate nucleotides rapidly, which enhances throughput in PCR reactions.
Efficiency also encompasses how well the enzyme can work under different conditions. Environmental factors such as ionic strength, pH, and temperature can alter enzyme performance. Finding a polymerase with optimal efficiency for your specific conditions is crucial. By conducting preliminary tests, researchers can determine which polymerase functions best under their unique experimental conditions, thus ensuring reliable amplification.
In summary, selection criteria for DNA polymerase, including fidelity and processivity, must be thoughtfully considered to enhance PCR outcomes. The right choice can vastly improve the reliability of experimental data and significantly affect subsequent analyses.
Current Applications of DNA Polymerase in Research
The use of DNA polymerase in research has transformed the landscape of molecular biology. It enables scientists to explore genetic sequences, clarify forensic evidence, and diagnose clinical conditions effectively. The following sections will discuss three significant applications of DNA polymerase: genetic research and manipulation, forensic science applications, and clinical diagnostics.
Genetic Research and Manipulation
In genetic research, DNA polymerase plays a crucial role in amplifying specific DNA segments. This amplification is vital for various applications such as cloning, sequence analysis and gene expression studies. Researchers often utilize polymerases with high fidelity for these purposes to ensure that the resultant DNA is an accurate replica of the original template.
The choice of DNA polymerase impacts the success of experiments. For instance, Taq polymerase is commonly used for its robustness, while Pfu polymerase is chosen for its proofreading ability, enhancing fidelity. By manipulating genetic material, researchers can create transgenic organisms or study gene functions, which further contributes to fields like gene therapy and biotechnology.
In summary, the advancements in genetic manipulation hinge on the ability to replicate DNA accurately, underscoring the importance of DNA polymerase in these applications.
Forensic Science Applications
Forensic science heavily relies on DNA polymerase for analyzing genetic material obtained from crime scenes. The polymerase chain reaction (PCR) is instrumental in amplifying trace amounts of DNA, which is often all that can be recovered in complex samples. This amplification is key in linking a suspect to a crime or exonerating innocent individuals.
Different types of DNA polymerases are employed based on the nature of the samples. For instance, high-fidelity polymerases may be used to minimize errors while analyzing degraded DNA. Additionally, the use of multiplex PCR allows for simultaneous amplification of multiple loci, increasing the efficiency of DNA profiling.
"The application of DNA polymerases in forensic science allows for the resolution of cases that were previously deemed unsolvable due to lack of sufficient DNA evidence."
The integration of DNA polymerase technology continues to evolve, contributing to forensic databases and aiding in legal investigations.
Clinical Diagnostics
In clinical diagnostics, DNA polymerase is pivotal in various testing methods including those for infectious diseases and genetic disorders. PCR assays enable rapid testing for pathogens by amplifying their genetic material from patient samples, thus facilitating timely treatment. This application is shown in tests for conditions such as COVID-19, where rapid and accurate results are essential.
Furthermore, in the realm of genetic disorders, polymerase is used to identify mutations in specific genes. By selecting appropriate polymerases, technicians can tailor the amplification process to satisfy specific diagnostic needs. This precision enhances the accuracy of tests and improves patient outcomes.
Overall, the role of DNA polymerase in clinical diagnostics underscores its significance, providing healthcare professionals with essential tools for disease detection and patient management.
Challenges in PCR Related to DNA Polymerase
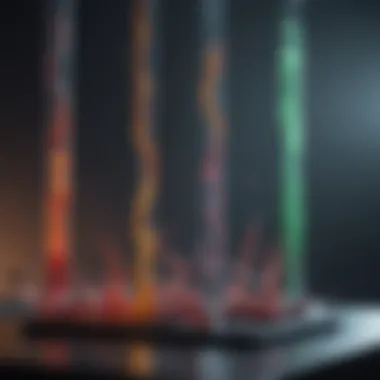
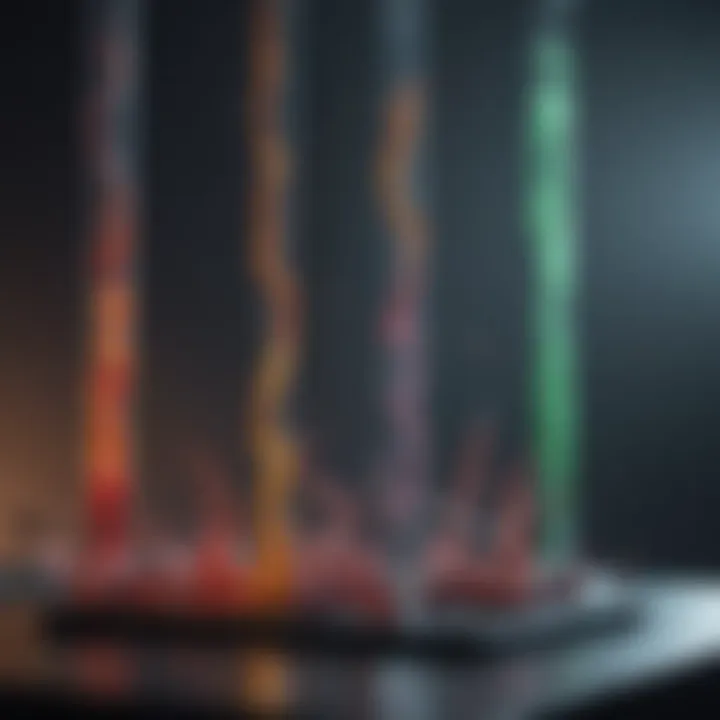
The effectiveness of the polymerase chain reaction (PCR) relies significantly on the properties and performance of DNA polymerase. While DNA polymerase is a fundamental component of this technique, there are challenges that researchers must confront to optimize their results. Understanding these challenges is crucial for improving the accuracy and efficiency of PCR applications.
Several factors can inhibit the activity of DNA polymerases, leading to suboptimal amplification. Other issues, such as error rates and misincorporation events, can also affect the fidelity of the PCR products. This section explores these challenges in a detailed manner.
Inhibition Factors
Inhibition of DNA polymerase can result from different sources during the PCR process. These inhibitors can come from various biological samples, including proteins, phenolic compounds, and other contaminants.
- Sample Type: Different types of biological materials, such as blood or tissue, may contain inhibitors that reduce DNA polymerase activity. For instance, heme found in blood products can bind to enzymes and limit their function.
- Chemicals: Environmental substances like ethanol and detergent can impede polymerase performance. Commonly used extraction and purification methods can leave residuals in samples that negatively influence PCR.
- Complex Mixtures: The presence of multiple DNA or RNA species can cause competition for the enzyme, affecting the efficiency of amplification.
These inhibition factors necessitate careful preparation and purification of samples to minimize interference. Removing known inhibitors before PCR setup is essential for maximizing amplification yield and accuracy.
Error Rates and Misincorporation
Despite advances in DNA polymerase technology, error rates during DNA synthesis remain a significant concern. Errors like misincorporation can lead to the generation of incorrect sequences, which in turn affects downstream applications such as cloning or sequencing. Understanding the implications of these errors is vital, especially in fields like forensic science or clinical diagnostics where precise results are crucial.
- Fidelity Variability: Different DNA polymerases exhibit varying fidelity levels, which is influenced by their intrinsic properties. Higher fidelity polymerases, like Phusion or Q5, tend to have lower error rates than traditional ones such as Taq polymerase.
- Temperature Sensitivity: PCR conditions like temperature cycles can impact misincorporation rates. Higher temperatures during denaturation can allow more flexibility among the enzymes, leading to an increased chance of errors.
- Polymerase Processivity: Processivity refers to the ability of DNA polymerase to synthesize a long strand of DNA without dissociating from the template. Low processivity can result in more frequent errors due to repeated attempts to bind to the template, increasing the chances of misincorporation.
Careful selection of DNA polymerase and optimization of PCR conditions can reduce error rates and enhance the accuracy of amplified products.
Overall, addressing these challenges requires a deep understanding of the interaction between DNA polymerase and the PCR environment. Only through detailed consideration of these factors can researchers achieve consistent and reliable results in their experiments.
Recent Advances in DNA Polymerase Technology
The advancements in DNA polymerase technology are essential for improving the efficacy and versatility of polymerase chain reaction (PCR). These innovations address critical challenges in amplification processes, making them more efficient and reliable. Keeping pace with the rapidly evolving landscape of molecular biology, the enhancements in DNA polymerase not only boost the performance of existing methodologies but also enable new applications that were once inconceivable.
Innovations in Polymerase Development
One significant area of development has been the engineering of DNA polymerases with enhanced characteristics. Many researchers focus on increasing fidelity, which refers to the ability of the enzyme to accurately incorporate nucleotides during DNA synthesis. High-fidelity polymerases are pivotal for applications requiring precise replication, such as cloning and sequencing. Recent innovations include the modification of enzyme structures to minimize misincorporation events without sacrificing speed.
Additionally, specialized polymerases have emerged, designed to function in extreme conditions. The thermostable polymerases from organisms like Thermus aquaticus have made it possible to carry out PCR at high temperatures, greatly improving the efficiency of the process. Newer versions of such enzymes exhibit even higher stability and activity, facilitating rapid cycling in PCR protocols.
Another innovative approach is the development of polymerases with unique accessory domains that promote processivity. Processivity refers to the ability of polymerases to continuously synthesize DNA without dissociating from the template. This is particularly crucial in applications that require long-range amplification. By integrating additional functional domains, researchers enhance the ability of polymerases to maintain interaction with the template, allowing for longer stretches of DNA to be synthesized efficiently.
Enhanced PCR Techniques
Accompanying the innovations in polymerase development are novel techniques that optimize the PCR process itself. Improvements in PCR protocols have been instrumental in achieving higher yields and specificity in amplification. Some techniques involve fine-tuning the concentration of magnesium ions, essential cofactors for polymerase activity. Adjusting the magnesium concentration can significantly influence both the fidelity and yield of PCR.
Another advancement is the introduction of multiplex PCR, which enables the simultaneous amplification of multiple targets within a single reaction. This technique is particularly useful in clinical diagnostics where testing for several pathogens or genes is essential. Enhanced polymerases with higher tolerance to inhibitors present in complex samples have made these multiplex assays more reliable.
Moreover, the development of real-time PCR (qPCR) has transformed the landscape of quantitative analysis. Using advanced DNA polymerases, qPCR allows researchers to monitor the progress of PCR in real-time, providing quantitative data regarding the amount of starting material. This application is invaluable in research contexts such as gene expression analysis and viral load quantification.
"The evolution of DNA polymerase technology is playing a key role in advancing molecular diagnostics and research capabilities."
Future Directions for DNA Polymerase Research
The landscape of DNA polymerase research is rapidly evolving, propelled by advancements in molecular techniques and a growing understanding of genetic processes. This section discusses the significance of future research in DNA polymerases, addressing various trends and potential clinical applications.
Emerging Trends in Molecular Biology
Recent developments in molecular biology indicate a shift towards innovative DNA polymerases that exhibit superior performance. These include polymerases engineered for enhanced tolerance to extreme conditions, such as high temperatures or reactions with complex templates. Furthermore, next-generation DNA sequencing (NGS) has sparked interest in proofreading polymerases that can reduce error rates during amplification. The trend is moving towards polymerases that not only function optimally in standard PCR but also adapt to unique challenges presented in complex genomic sequences.
"The future of DNA polymerases is intertwined with the advancements in gene editing and synthetic biology, promising broader applications across various disciplines."
Moreover, the exploration of polymerases derived from microorganisms inhabiting extreme environments, like hot springs or arctic conditions, has opened pathways to discover enzymes with new properties. Enhanced specificity and modified processivity of these polymerases can result in higher yields of DNA synthesis and more reliable results in applications such as cloning and gene expression studies.
Potential Clinical Applications
The implications of novel DNA polymerases are profound, especially in clinical settings. One significant area is the development of polymerases that can facilitate rapid diagnostics. Such advancements pave the way for more accurate tests within shorter timeframes, critical in situations like infectious disease outbreaks or genetic screenings. The use of high-fidelity polymerases in PCR-based tests enhances the precision of genetic mutations detection, improving personalized medicine approaches.
In addition, engineered polymerases are crucial in gene therapy. The need for efficient and safe gene editing tools has led to an interest in polymerases that can be seamlessly incorporated into CRISPR systems. This integration could lead to targeted gene correction with minimized off-target effects, presenting a revolutionary approach to treating genetic disorders.
Furthermore, the integration of DNA polymerases in microfluidic devices highlights a future direction where portable and efficient diagnostics become accessible. Such devices, using advanced polymerases, could analyze samples in field conditions, benefiting remote healthcare applications.