Embryonic Body Formation: A Detailed Exploration
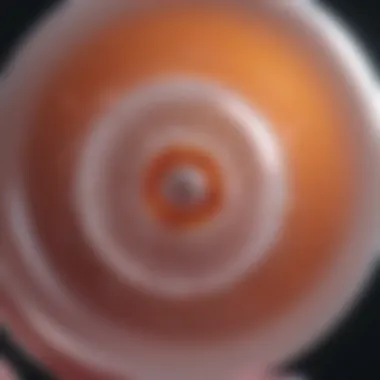
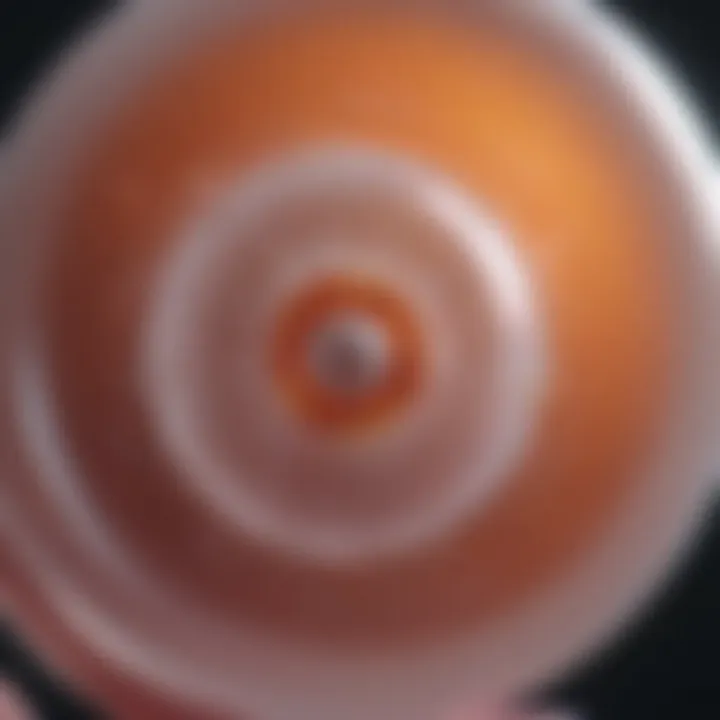
Intro
Embryonic body formation is an essential topic within the field of developmental biology. It not only encompasses the process of how an organism transforms from a single fertilized cell into a complex structure with differentiated systems but also reveals a great deal about the principles of life itself. From the moment of fertilization, where a sperm meets an egg, to the intricate orchestration of cells becoming tissues and organs, each step presents its own set of challenges and marvels.
Understanding this journey sheds light on various aspects of biology, such as genetic regulation, cell signaling, and evolutionary adaptations. The mechanisms involved differ across species, yet share common threads that highlight the unity in diversity of life.
As we embark on this exploration, we'll touch on crucial concepts like cell differentiation, where unspecialized cells become specialized in function, and morphogenesis, the process by which these specialized cells organize into structured forms. By comparing embryonic development across various organisms, we can glean insights into evolutionary processes and developmental anomalies that occur within different species.
Research Highlights
Key Findings
- Cell Differentiation Pathways: Various organisms utilize unique paths in cell differentiation, yet share common signaling molecules that guide these processes.
- Morphogen Gradients: The role of morphogens in establishing body axes and promoting cellular organization is pivotal. These gradients dictate where and how cells differentiate.
- Conservation Across Species: Despite diversification in form and function, there are conserved genes and developmental pathways that underscore the shared ancestry of multicellular organisms.
Understanding these highlights not only enriches our knowledge of biology but also presents avenues for practical applications in fields like regenerative medicine and comparative genomics.
Implications and Applications
The implications of studying embryonic body formation reach far beyond theoretical understanding. Here are some important areas:
- Medical Insights: Comprehending abnormal development can inform medical practices, including the treatment of congenital disorders.
- Conservation Efforts: Knowledge can assist in conservation strategies by understanding the developmental biology of endangered species.
- Biotechnology Applications: Insights into cell differentiation can lead to advancements in tissue engineering and regenerative therapies.
Methodology Overview
Research Design
To grasp embryonic body formation, researchers often adopt a multi-faceted approach that combines observational studies, experimental manipulations, and comparative analyses. The integration of genomics and live imaging techniques proves crucial for tracking developmental changes in real-time.
Experimental Procedures
- Model Organisms: Systems such as zebrafish, fruit flies, and mice are commonly used, allowing researchers to leverage their well-mapped genetics and developmental stages.
- Gene Editing: Techniques like CRISPR-Cas9 enable scientists to manipulate specific genes to observe their effects on development.
- Imaging Techniques: Advanced imaging methods facilitate the observation of cellular behaviors during different developmental stages, helping to visualize how cells change and interact.
Through these methodologies, the complex dynamics of embryonic development can be illuminated, fostering a deeper understanding of the inherent processes that shape life.
With this foundational framework, we shall proceed to discuss the cellular mechanisms, genetic factors, and evolutionary considerations that underpin embryonic body formation in the following sections.
Intro to Embryonic Body Formation
The study of embryonic body formation encapsulates a pivotal aspect of developmental biology, serving as a foundation for understanding the processes that lead from a single cell to a fully formed organism. This journey begins with fertilization, a seemingly simple interaction that layers complexity as it unfolds through cell divisions, migrations, and differentiations.
Significance of Embryonic Development
Embryonic development plays an instrumental role in the health and stability of species. It isn't merely about how an organism comes into existence; it's about how life ensures its continuity and adaptability. Each stage of embryonic formation contributes to a myriad of biological functions and identifies how organisms respond to their environments.
- Adaptation and Survival: The varied developmental paths taken by different organisms are prime examples of evolution in action and adaptation.
- Medical Importance: Understanding embryonic development can lead to breakthroughs in regenerative medicine and therapies, especially concerning congenital anomalies and infertility.
- Insight into Evolution: By examining embryos across species, researchers can unravel evolutionary relationships and developmental strategies.
Ultimately, the significance of embryonic development extends well beyond academics; it is deeply intertwined with key biological innovations that affect health, biodiversity, and ecological balance.
Overview of Developmental Biology
When we turn to developmental biology, we are essentially peering into the mechanisms that govern the creation and organization of life. This branch of biology not only considers how organisms grow but also why they follow particular pathways. The intertwining of genetics, environment, and evolution creates a complex tapestry of interactions that guide development.
- Key Concepts: Understanding the fundamentals such as cell signaling, differentiation, and morphogenesis allows a more profound appreciation of how organisms are more than mere collections of cells.
- Current Research: The field is rife with ongoing investigations into how epigenetic factors influence development and how advancements in genomic techniques can unveil new layers of understanding.
Fertilization: The Initiating Event
Fertilization serves as the cornerstone of embryonic development, marking the very moment when life embarks on its complex journey from a single cell to a fully formed organism. This pivotal event lays the groundwork for all subsequent developmental processes. Understanding fertilization is essential, as it not only initiates the development of the zygote but also sets the stage for genetic diversity and the transmission of traits from one generation to the next.
Gamete Interaction
At the heart of fertilization lies the interaction between gametes—the sperm and the egg. Each gamete carries half of the genetic material necessary for forming a complete organism. The interaction begins when the sperm encounters the egg, facilitated by a series of biochemical signals. The sperm has to undergo a process called capacitation, which enhances its ability to penetrate the egg's protective layers.
Once the sperm succeeds in adhering to and penetrating the egg's outer shell, a cascade of events is triggered. The egg, equipped with specialized structures like the Zona Pellucida, ensures that only one sperm can enter. This polyspermy prevention is crucial; otherwise, the genetic blueprint would be overwhelmed, disrupting normal development.
In essence, the successful interaction between the sperm and egg is a fine-tuned orchestration involving:
- Cell recognition
- Membrane fusion
- Activation of the egg
Each of these steps is vital to ensuring that fertilization occurs successfully without complications.
Zygote Formation
Following successful gamete interaction, the creation of the zygote marks the next step in this extraordinary journey of development. The zygote, a product of sperm and egg fusion, contains a complete set of genetic instructions, ready to kickstart a multitude of cellular processes. But it’s not merely a matter of merging the two genetic materials; the process is accompanied by a series of rapid cell divisions known as cleavage, paving the way for embryonic development.
After fertilization, the zygote travels down the fallopian tube, undergoing several divisions en route. This journey is more than just transportation; it is during this time that initial cell specialization begins. As the zygote divides, each resulting cell—called a blastomere—is a clone of the original zygote but gradually starts to show differences in function and fate as they’ll contribute to various tissues and organs.
"The creation of the zygote is not just the merging of two cells; it’s the inception of a new life that embodies potential and complexity."
By the time the zygote reaches the uterus, it typically forms into a blastocyst, which will eventually implant into the uterine lining, marking the transition into the next critical phase of development.
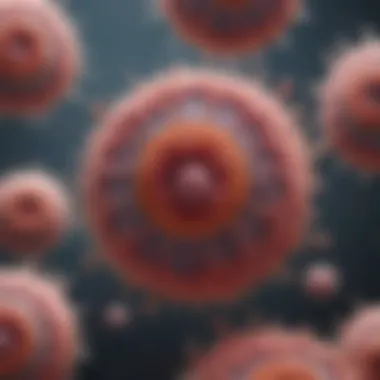
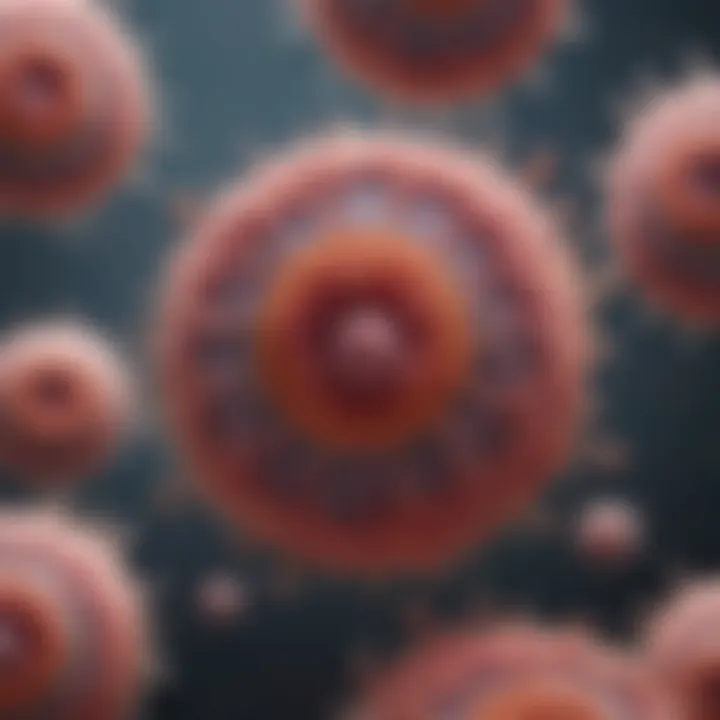
The significance of zygote formation cannot be underestimated. It serves as the point at which genetic diversity is established, influenced by factors like parental genome contributions, mutations, and even environmental interactions, all of which shape the future organism.
Understanding fertilization extends beyond mere biological curiosity; it unveils the miraculous complexity of how life begins and how it is intricately woven with genetic heritage. By examining these processes, we open the door to advancements in fields like reproductive technology, genetic engineering, and even evolutionary biology.
Early Developmental Stages
Understanding the early developmental stages of embryonic formation is crucial as these stages lay the groundwork for the successful development of an organism. From the moment a zygote is formed after fertilization, every subsequent division and differentiation sets the stage for future complexity and functionality in the organism. Researchers and students alike are keenly aware of the importance of these initial phases, for they determine not only the physical structure but also the timing of various cellular functions and the overall health of the resulting embryo.
Crucially, early development consists of several distinct phases - cleavage, blastocyst formation, and gastrulation. Each of these stages has its own significance, intricately tied to cellular behavior and gene expression. This interplay is vital for forming the foundational body plan of an organism, influencing how organs and systems will eventually evolve. Thus, a thorough comprehension of this spectrum of stages benefits a diverse audience, ranging from biologists exploring developmental mechanics to medical professionals who utilize this knowledge for advances in regenerative medicine and treatment of congenital anomalies.
Cleavage Patterns
In the moment following fertilization, the zygote begins to divide in a process termed cleavage. Unlike typical cell division, cleavage is characterized by rapid, successive divisions that contribute to an increasing number of cells without a corresponding increase in size. This results in a cluster of smaller cells called blastomeres. The patterns of cleavage—such as holoblastic, where the entire egg divides, or meroblastic, where only a portion divides—play a critical role in the way the developing embryo will form.
For example, in holoblastic cleavage, seen in species like frogs, the entire zygote divides into smaller units evenly, allowing for symmetrical development. In contrast, meroblastic cleavage, typical of birds, results in a yolk-rich single cell where only a part undergoes division, causing an asymmetrical structure. The pattern of cleavage affects not just cell size but also the cellular fate in later stages, leading to the formation of specific tissues and organs.
"The pattern of cleavage is a prelude to the choreography of embryonic development, setting the stage for the complex interactions that follow."
Blastocyst Formation
Following cleavage, the formation of the blastocyst marks a turning point. The blastocyst consists of an inner cell mass, which will eventually develop into the embryo, and an outer layer called the trophoblast. The trophoblast is essential for implantation into the uterine wall, initiating a critical connection to the mother’s bloodstream for nutrient exchange.
The transition from a solid ball of cells to a blastocyst involves the accumulation of fluid within the blastomeres, which pushes them outward, forming a hollow structure. This fluid-filled cavity, known as the blastocoel, not only provides pivotal structural support but also initiates the first signs of differentiation among the cells. For many organisms, this stage ultimately determines the viability and success of pregnancy, shedding light on how early failures can lead to miscarriage or developmental anomalies.
Gastrulation: A Turning Point
Gastrulation serves as a transformative experience for the embryo and is often considered one of the most significant stages of early development. This phase reorganizes the single-layered blastoderm into a multi-layered structure. Cells migrate and rearrange into distinct layers known as germ layers: the ectoderm, mesoderm, and endoderm. Each layer is a precursor to specific tissues and organs; for instance, the ectoderm will evolve into skin and nervous system components, the mesoderm into muscles and bones, and the endoderm into internal organs.
The process of gastrulation is complex and varies notably among species, yet its importance cannot be overstated. It is during this time that the embryo begins to establish its body plan, set the course for organogenesis, and introduces vital signaling pathways that govern future developmental processes. The reorganisation of cells during this period can have profound implications, impacting the organism's structural and functional outcome.
Cell Differentiation Mechanisms
Cell differentiation is a fundamental process in embryonic development. It's the stage where unspecialized cells transform into specialized cells, each with distinct functions. This transformation is like a well-orchestrated movie; every actor plays a vital role in the production. Understanding the intricacies of cell differentiation mechanisms is essential for grasping how complex organisms develop from a single zygote into a fully formed entity.
The importance of this topic in embryology can't be overstated. Not only does it reveal the blueprint that dictates how cells emerge and evolve, but it also highlights the delicate interplay between various biophysical and biochemical signals. By studying these mechanisms, researchers gain insights that can lead to potential medical breakthroughs and treatments for developmental disorders.
Role of Stem Cells
Stem cells are at the heart of differentiation. Often viewed as a blank canvas, they possess the unique ability to become any cell type in the body. This versatility underlies the whole mechanism of growth and repair. Imagine a carpenter who can create whatever the client desires—this is essentially what stem cells can do in the biological world.
There are two main types of stem cells relevant to this process:
- Embryonic Stem Cells: Found in the early stages of development; they can differentiate into any cell type.
- Adult Stem Cells: These are more limited, typically differentiating into a specific type of cell relevant to the tissue or organ they are derived from.
The role of stem cells in differentiation goes beyond mere potential; they respond to a myriad of signals that guide their fate. Factors such as the local environment, physical connections with other cells, and intrinsic signals all work together like a well-tuned orchestra, ensuring that the correct cell types are formed at the right time and location.
Signaling Pathways in Differentiation
Signaling pathways form the communication lines that regulate cell differentiation. These pathways involve a series of molecular interactions that translate external cues into cellular responses. Think of them as a complex set of traffic lights, directing when and how cells should progress on their journey to specialization.
Several key pathways are particularly important:
- Wnt Signaling: This pathway plays a role in cell fate determination and is crucial during early cell divisions.
- Notch Signaling: Involved in lateral inhibition, this ensures that neighboring cells do not adopt the same fate, maintaining diversity in cell types.
- Hedgehog Pathway: This signaling is vital for establishing body patterns during development.
By closely examining these pathways, scientists can identify how cells communicate during differentiation and how interruptions in these pathways may lead to anomalies. The more we understand these signaling networks, the better equipped we are to address developmental issues or diseased states in various organisms.
Cell differentiation is not merely a phase of development; it's the symphony of life that composes our very being, orchestrated through the collaborative efforts of stem cells and signaling pathways.
Morphogenesis: Shaping the Body
Morphogenesis is central to understanding embryonic body formation, serving as the blueprint for how a simple group of cells transfigures into a complex, multicellular organism. This process involves a series of orchestrated cellular behaviors that shape the tissues and organs of the developing embryo. Without morphogenesis, embryonic structures would lack form, and developmental processes critical for survival would be hindered.
At its core, morphogenesis relies on cellular movement and organization. Cells must not only migrate to their appropriate locations but also communicate with each other to establish the various layers and systems that characterize an organism. This includes the intricate balancing act between adhesion and differentiation. Cells must stick together in the right fashion for tissues to hold but also be able to change their roles as the embryo grows, maintaining a fluidity that is key to development.
Cell Migration Processes
Cell migration is a fundamental act during morphogenesis. During this phase, cells on the embryo’s surface begin to move from their original location to form new structures. For instance, in vertebrates, epiblast cells migrate during gastrulation to form the mesoderm and endoderm.
This migration can be described in several steps:
- Initiation: The process often begins with signals from neighboring cells, nudging targeted cells to exit their current position.
- Adhesion Breakdown: Cells must detach from their original cytoskeleton connections and break the adhesive links that have held them in place.
- Movement: Using cellular machinery such as actin filaments or microtubules, cells crawl towards new locations.
- Re-establishment of Adhesion: Finally, cells make new connections in their intended locations, forming new intercellular bonds.
"Cell migration is not just a journey, but a carefully orchestrated performance where each part must know its role."
Tissue Formation Dynamics
As cells migrate, they don’t merely travel aimlessly. The process of tissue formation is tightly interwoven with the dynamics of cell migration, morphing loose groups of cells into highly structured tissues. This happens through several processes:
- Cell Proliferation: New cells are generated, allowing tissues to grow and fill spaces, which helps in the formation of organ systems.
- Differentiation: Not all cells will play the same role; they adapt to specific functions. For example, in forming muscle tissues, some cells will become muscle fibers, while others will become connective tissues to support muscle structure.
- Extracellular Matrix (ECM) Interaction: The ECM serves as a scaffold for cells. How cells interact with this matrix determines not only their migration patterns but also their final shape and function.
In summary, morphogenesis integrates various aspects of cellular activities and the surrounding microenvironment. The resultant organization establishes anatomical structures that are vital for functional capabilities in the organism. Understanding these processes provides key insights into developmental biology, shedding light on how structure emerges from what initially appears as chaos. This knowledge is critical, especially as researchers seek to address developmental disorders and investigate regenerative medicine.
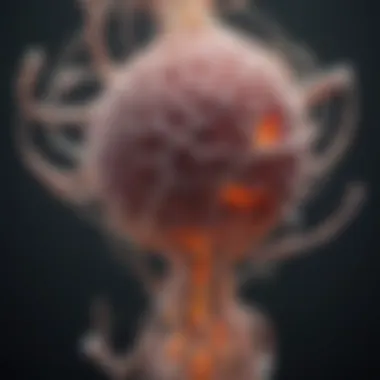
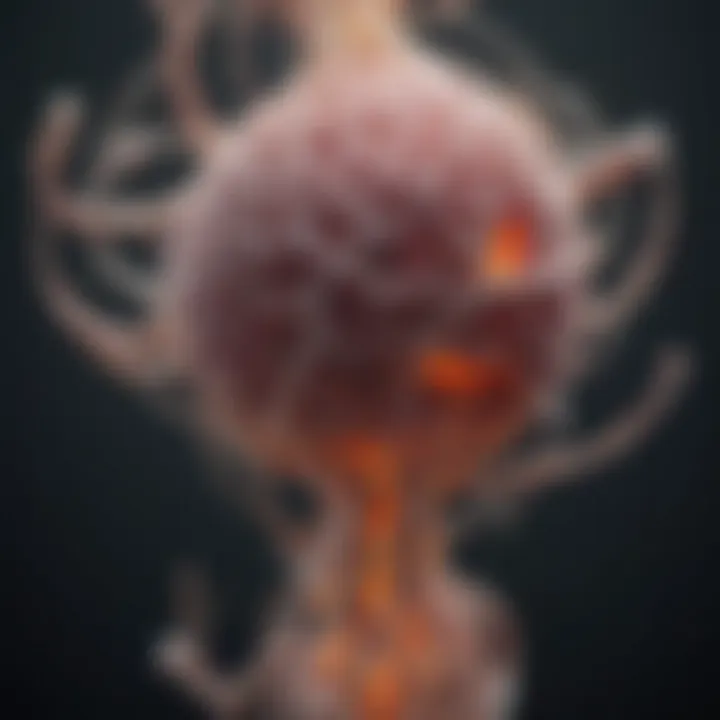
Genetic Regulation of Development
Understanding genetic regulation is a cornerstone in the study of embryonic development. This intricate web of molecular interactions guides cells through a precise choreography of differentiation and specialization, laying the groundwork for complex organismal structures. Without these genetic instructions, the intricate patterns that define an organism would be mere chaos.
The importance of genetic regulation lies in its ability to orchestrate development at multiple levels. Key mechanisms involve various types of genes, such as regulatory genes, structural genes, and even non-coding RNAs, which are fundamental to controlling how and when genes are expressed. Such regulation ensures that cells respond appropriately to internal and external cues, ultimately guiding the overall embryonic formation process.
Role of Homeotic Genes
Homeotic genes are the champions of genetic regulation. They dictate where body structures will form during embryological development. You might have heard of the Hox genes, which are a subset of homeotic genes; these are critical in determining an organism's layout, from head to tail.
The role of homeotic genes can be summarized as follows:
- Body Plan Specification: They assign specific identities to segments of the embryo, guiding where limbs, organs, and other features will grow.
- Conserved Nature Across Species: Fascinatingly, many homeotic genes remain similar across a range of species, suggesting a common evolutionary ancestry.
- Mutational Consequences: When these genes malfunction, it can lead to drastic developmental anomalies. For example, the mutation of a single homeotic gene can result in the development of a limb in place of an antenna in fruit flies, showcasing their power in development.
Homeotic genes are, in essence, like the blueprint for a house. They provide the foundational layout and define how each part fits together.
Epigenetic Factors in Embryogenesis
Epigenetics can be viewed as the fine-tuning of genetic regulation, influencing the way genes express themselves without altering the underlying DNA sequence. This aspect is particularly critical during embryogenesis, wherein a single fertilized egg transforms into a multicellular organism.
Some essential points concerning epigenetic factors include:
- Modifications by Environment: External influences such as temperature, nutrition, and pollutants can modify how genes are expressed. For instance, if a developing embryo encounters stress, the epigenetic marks may change the expression of vital genes.
- Transgenerational Effects: Interestingly, epigenetic changes are not always confined to the individual embryo but can also be passed down to future generations, impacting their development and health.
- Potential for Medical Advances: Understanding epigenetic regulation opens doors for innovative therapies and regenerative medicine. Researchers are exploring if modifying epigenetic marks could lead to better treatments for genetic diseases.
In summary, genetic regulation is a multifaceted domain that encompasses both homeotic genes and epigenetic factors. Together, they provide a comprehensive framework that enables precise control over embryonic development, ensuring that each stage unfolds according to plan.
Comparative Embryology
Comparative embryology examines how different organisms develop from fertilization through to the establishment of their body plans. This branch of biology is crucial not just for understanding the processes of development, but also for shedding light on evolutionary relationships among species. By exploring the similarities and differences in embryonic development, we can glean insights into how various life forms adapted to their environments through the ages, all while harnessing underlying biological principles.
One significant value of comparative embryology lies in its ability to highlight conserved developmental mechanisms. For instance, the genetic pathways guiding embryogenesis are often surprisingly consistent across diverse species, suggesting a shared evolutionary heritage. Organisms such as fruit flies, frogs, and mice may look drastically different in their adult forms, but when you look at the embryos, similarities start to emerge. These relationships help biologists trace back the evolutionary lineage, uncovering common ancestors hidden in the gene pool.
Embryonic Development in Different Species
When comparing embryonic stages across various species, one can't help but notice the remarkable diversity that arises from seemingly similar beginnings. The early cleavage patterns in a broad range of organisms, from humans to echinoderms, demonstrate variances in symmetry and potential fates that set the stage for vastly different developmental pathways. For example, the radial cleavage observed in amphibians differs significantly from the spiral cleavage of mollusks, indicating divergent evolutionary strategies.
Moreover, the blastocyst formation in mammals is another fascinating example. This stage of development is notable for its significance in implantation and further development. In contrast, species such as reptiles exhibit a different approach to early development, featuring yolk-rich eggs which result in varying growth patterns. These variations reinforce the notion that embryonic development is influenced by environmental factors, reproductive modes, and evolutionary pressures. Understanding these processes deepens our grasp of biological systems and opens avenues for potential medical advancements down the line.
Evolutionary Perspectives
The evolutionary perspective in comparative embryology offers a lens through which we can view the interconnectedness of life forms. It is not merely about tracing back lineages but also observing how developmental processes optimize survival in changing environments. Consider the variation in embryonic development between placental mammals and marsupials. While both groups share a common ancestor, their divergent paths regarding gestation reflect adaptations to their ecological niches.
This illustrates the principle of evolutionary developmental biology (evo-devo). Here, it's fascinating to note how certain developmental genes, known as homeobox genes, play a role across multiple species, ensuring that critical body structures develop in a harmonious manner. As such, the simplicity of a developmental process can disguise its complexity, hinting at an intricate balance of conservation and innovation sustained through evolution.
*"In comparative embryology, the key lies not just in studying the end products of evolution, but in understanding the developmental stages that shape these outcomes."
From an educational standpoint, incorporating findings from comparative embryology into curriculum frameworks enriches student perspectives on biological diversity. Furthermore, the evolutionary insights gained can inform conservation efforts, as understanding how species develop may assist in devising strategies to protect vulnerable species.
In summary, comparative embryology serves as a bridge connecting the realms of biology, evolution, and ecology, offering profound insights into the very fabric of life. This exploration allows us to understand not only the various paths taken by different species, but also the universal themes that underpin the beauty of embryonic development.
Anomalies in Embryonic Development
Anomalies in embryonic development serve as a critical area of study within the broader field of embryology. The investigation of these anomalies extends beyond mere curiosity; it provides essential insights that can impact medical science and health outcomes. Understanding how developmental processes can go awry enables researchers to identify methods for early diagnosis and potential treatment solutions for various congenital conditions. Additionally, studying these anomalies gives clues about normal developmental mechanisms, further enhancing our comprehension of the complexity inherent in embryonic formation.
Types of Developmental Anomalies
Developmental anomalies can be categorized into several types, each with distinct characteristics and implications:
- Structural anomalies: These refer to physical malformations in the body’s architecture. Common examples include cleft lip, spina bifida, and congenital heart defects. Such abnormalities often arise from disruptions occurring during critical periods of development.
- Functional anomalies: These affect how a part of the body functions rather than its physical structure. Examples include metabolic disorders or issues with organ function that can stem from errors in genes responsible for crucial processes.
- Chromosomal anomalies: This category involves abnormalities in the number or structure of chromosomes. Conditions like Down syndrome are a result of such chromatin errors, often leading to significant physical and intellectual disabilities.
- Developmental delays: While not structural, these may present as issues in growth or cognitive abilities based on the usual developmental timeline, with origins that could correlate with both environmental and genetic factors.
Understanding these categories helps to inform therapeutic strategies and provides a framework for further research, aiming to mitigate the impact of these anomalies.
Causes and Implications
The causes of developmental anomalies can vary widely, tracing back to genetic predispositions as well as environmental influences.
- Genetic Factors: Many anomalies arise due to inherited traits or mutations. For instance, single-gene disorders can significantly disrupt the regular sequence of embryonic development. Understanding the role of genes, such as homeobox genes, is vital as they are pivotal in determining body plan and organ formation.
- Environmental Factors: Certain external factors, often referred to as teratogens, can cause significant disturbances. These include substances like alcohol or certain drugs, infections during pregnancy, and even nutritional deficiencies. For instance, maternal exposure to folic acid deficiency has been linked to neural tube defects.
- Multifactorial Causes: Often, it is a combination of genetic and environmental factors that lead to anomalies. This complexity suggests that future strategies in prevention might require a multidimensional approach involving both genetic counseling and environmental assessments.
"Understanding the causes of anomalies allows for developing pathways towards prevention and treatment, addressing both genetic errors and environmental exposures."
The implications of these anomalies are vast. They not only affect individuals but also impact families, healthcare systems, and society at large. Early identification and intervention can mitigate many associated challenges, fostering a more profound understanding of both normal and aberrant development while ultimately enhancing quality of life.
Technological Advances in Embryology
Technological advancements have transformed the field of embryology, providing new tools and methods for understanding the complex processes of embryonic development. The significance of these technologies extends beyond mere observation; they create opportunities for researchers to interrogate developmental mechanisms at a molecular level, enabling targeted studies and potentially groundbreaking applications in medicine and science. These innovations equip scientists with sharp instruments to dissect the intricate layers of embryogenesis, tracking changes that have vast implications for both individual health and species evolution.
Genomic Techniques
One of the cornerstones of modern embryological study is the use of genomic techniques. Methods such as CRISPR-Cas9 gene editing have revolutionized the ability to manipulate genes in various organisms. By altering specific genes, researchers can observe the resultant changes in development, providing insight into genetic regulation during embryogenesis. For instance, in studies looking at zebrafish embryos, scientists can utilize this technology not only to knock out genes but also to add fluorescent markers. This allows for real-time monitoring of cell lineage and development.
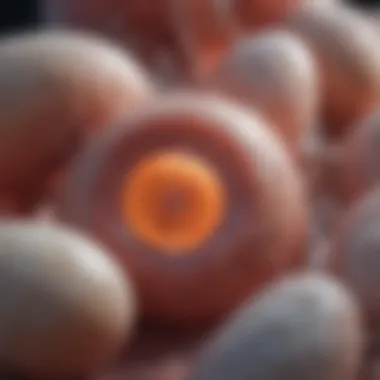
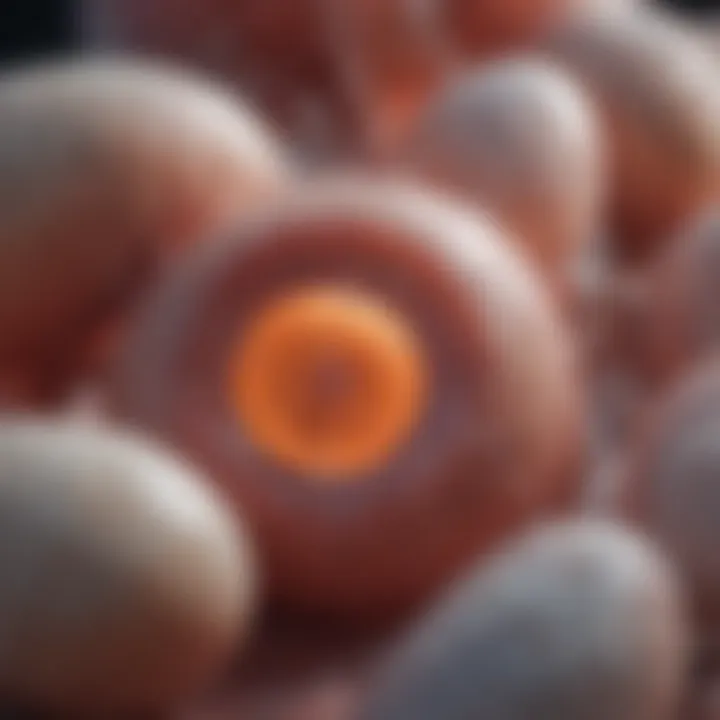
Some notable genomic techniques include:
- Next-Generation Sequencing (NGS): This technology has increased the efficiency and reduced the cost of whole-genome sequencing, enabling large-scale studies that were previously impractical.
- RNA-Seq: This allows for a comprehensive analysis of gene expression, facilitating the identification of genes that play vital roles during specific stages of embryonic development.
- Gene Knock-In and Knock-Out: These techniques enable the precise insertion or deletion of sequences within the genome, allowing for detailed studies of gene function.
Overall, genomic techniques provide a powerful lens through which to view the many facets of embryonic development, bridging the gap between molecular biology and developmental processes.
Imaging Technologies in Developmental Studies
Imaging technologies have also carved a niche in embryological research, becoming indispensable in observing how embryos develop over time. Traditional methods often provided static snapshots of development, but newer imaging technologies enable dynamic visualization, offering a clearer picture of the processes occurring in real-time.
Among these emerging technologies:
- Confocal Microscopy: This technique improves resolution and contrast in images, allowing for comprehensive three-dimensional reconstructions of developing embryos.
- Light Sheet Microscopy: This cutting-edge method illuminates the sample from the side, dramatically reducing photo-damage and allowing for prolonged observation of living embryos.
- Fluorescence Imaging: Using fluorescent tags attached to specific proteins, researchers can track cellular behaviors and interactions over time, revealing crucial information about cellular mechanisms within the embryo.
Importantly, these imaging technologies contribute to a holistic understanding of morphogenesis, offering insights into cell behavior that genomic data alone cannot provide. With the intricacies of embryonic development being unveiled by these techniques, researchers can better inform therapeutic approaches to developmental disorders.
"As we harness technological advancements in embryology, we begin to peel back layers of complexity in developmental biology, unveiling crucial insights that can inform future medical breakthroughs."
In summary, the integration of genomic techniques and advanced imaging tools has drastically altered the landscape of embryological studies. These advances not only enrich our basic understanding of embryonic development but also hold promise for practical applications in regenerative medicine and therapeutic interventions. Researchers are continuously seeking innovative ways to utilize these technologies, ensuring the future of embryology is bright and filled with potential.
Ethical Considerations in Embryonic Research
When engaging in the complex world of embryonic body formation, one cannot overlook the ethical considerations that accompany such research. As technology advances, the lines between scientific progress and moral implications become increasingly blurred. Understanding these ethical aspects is crucial not only for researchers but also for policymakers, legislators, and the general public. The ongoing conversations surrounding the ethical dimensions can greatly influence future research directions and public acceptance.
Debates Around Stem Cell Research
Stem cell research stands at the forefront of debates that touch deeply on ethical concerns. These cells, which may either be derived from embryos or adult tissues, have sparked intense discussions about the moral status of the human embryo.
Some arguments in favor of stem cell research highlight its potential benefits:
- Therapeutic Uses: Stem cells can differentiate into various cell types, opening doors for treatments of conditions such as Parkinson's disease, diabetes, and spinal cord injuries.
- Understanding Disease Mechanisms: Research can help uncover the paths of certain diseases through cell growth and differentiation, providing insights critical for prevention and treatment.
However, critics assert that using embryonic stem cells disregards the potential life and raises fundamental moral questions:
- Moral Status of the Embryo: Some believe human life begins at conception, making the use of embryos for research unethical.
- Exploitation Concerns: There is fear that the potential market for embryonic stem cell therapies could lead to the commodification of human life.
The debate is ongoing, with various countries adopting differing stances. Some nations have implemented strict regulations regarding stem cell research, while others have embraced more permissive approaches.
Implications for Human Embryo Studies
The implications of research on human embryos are multifaceted and merit careful consideration. As scientists delve into embryonic processes, they invariably encounter ethical dilemmas about intent, application, and long-term consequences.
One primary consideration is informed consent. It is imperative that individuals supplying embryos for research fully understand what the research entails, its potential benefits, and the possible consequences. Furthermore, the complexity of genetic editing technologies raises questions about the boundaries of acceptable manipulation. What changes can be ethically justified to alleviate suffering without venturing into the realm of designer babies or unintentional genetic consequences?
Moreover, regulatory frameworks vary significantly around the globe. Some nations restrict embryo studies to prevent potential abuses, while others encourage such exploration in the name of scientific advancement. Navigating these waters requires a balance between innovation and ethical accountability. Thus, the implications reach beyond scientific inquiry and enter the realms of law, personal beliefs, and societal norms.
"The ethical dimensions of embryonic research need to be navigated with caution, recognizing the profound respect that human life demands."
The Future of Embryonic Research
The future of embryonic research promises to bring groundbreaking advancements that can reshape medicine and biology as we know it. As we peel back the layers of molecular and cellular interactions that govern early development, we uncover opportunities that were once confined to the realm of science fiction. Not only does this research hold the potential to enhance our understanding of basic developmental processes, but it also opens doors to innovative therapies and solutions that could revolutionize health care. Below, we'll explore two significant aspects of this evolving field.
Potential Applications in Medicine
Advances in embryonic research have profound implications for the medical field. Here are some of the key areas where we might see significant transformations:
- Regenerative Medicine: The ability to derive stem cells from embryos has already provided insights into regenerative therapies. Future research aims to refine these techniques to create tissues and organs on demand, possibly addressing organ shortages.
- Personalized Medicine: By understanding the genetic and epigenetic factors that influence development, treatments could be tailored to individuals based on their unique embryonic development patterns. This could lead to more effective therapies with fewer side effects.
- Genetic Therapies: With innovations like CRISPR and other genome-editing technologies, we may see a rise in therapies that target genetic anomalies at the embryonic stage, potentially eradicating hereditary diseases before they can manifest.
The potential here is massive and hinges on continued research and ethical considerations to navigate the complexities of embryonic manipulation.
Innovations on the Horizon
Emerging technologies are set to propel embryonic research into uncharted waters:
- Computational Biology: The marrying of biology with computational models can accelerate research into embryonic patterns and predict the outcomes of interventions. By simulating developmental processes virtually, researchers can identify optimal conditions for growth and differentiation.
- Advanced Imaging Techniques: Cutting-edge imaging technologies like light-sheet microscopy allow scientists to observe embryonic development in real-time and at a high resolution. These methods provide insights that are crucial for understanding the dynamic processes at play in the early stages of life.
- Bioprinting: 3D bioprinting is a revolutionary technique that can create complex biological structures. Applications in embryonic research could pave the way for designing organoids that mimic multiple layers and functions of embryonic tissues, enhancing our understanding of development.
"As we stand on the brink of significant advancements in biotechnology, the responsibility lies with us to ensure ethical integrity in every step of embryonic research."
Through these innovations, future research in embryology is positioned to not only deepen our comprehension of developmental processes but also broaden the horizons of modern medicine. As the interplay between technology and biology becomes more intricate, we can expect to witness incredible developments that challenge our current understanding.
Closures
In the study of embryonic body formation, understanding the intricacies of various developmental processes proves invaluable. The significance of this exploration lies in its capacity to illuminate how multicellular organisms evolve from a single fertilized egg into complex systems. This knowledge not only enhances our fundamental grasp of biology but also has profound implications for medicine, genetics, and evolutionary studies.
Summary of Key Insights
Throughout this article, we have traversed a variety of topics that shape the landscape of embryology. Here are some of the pivotal points that were emphasized:
- Developmental Stages: Each stage of embryonic development, from fertilization to gastrulation, plays a critical role in dictating the eventual body plan of the organism.
- Cell Differentiation: The processes by which stem cells transform into specialized cells underscore the complexity of embryogenesis.
- Morphogenesis: This involves the physical processes that shape the body's form, indicating how organized patterns arise during development.
- Genetic Regulation: The fundamental role played by genetics and epigenetics illustrates how hereditary material influences developmental outcomes.
- Comparative Embryology: Analyzing embryonic processes across different species offers insights into evolutionary adaptations and helps us understand anomalies better.
The synthesis of these insights reveals not only an intricate web of biological interactions but also highlights the profound unity underlying all lifeforms.
Future Directions in Embryology
Looking ahead, numerous avenues for research and application stand out:
- Stem Cell Research: Continued exploration in this field may foster breakthroughs for regenerative therapies, potentially allowing us to repair or replace damaged tissues or organs.
- Genomic Advances: Innovations in genome editing technologies hold promise for correcting congenital defects before birth, fundamentally changing the way we approach hereditary diseases.
- Understanding Anomalies: By digging deeper into the causes and implications of developmental anomalies, researchers can develop preventive strategies, shedding light on disorders related to improper embryonic development.
- Technological Integration: Employing imaging techniques and computational modeling in studying embryonic development can facilitate a more comprehensive understanding of morphological changes and cell behaviors.