The Essential Functions of Mg ATP in Biological Systems
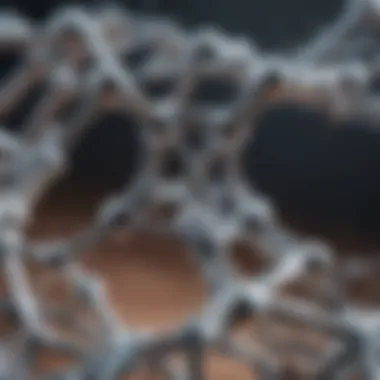
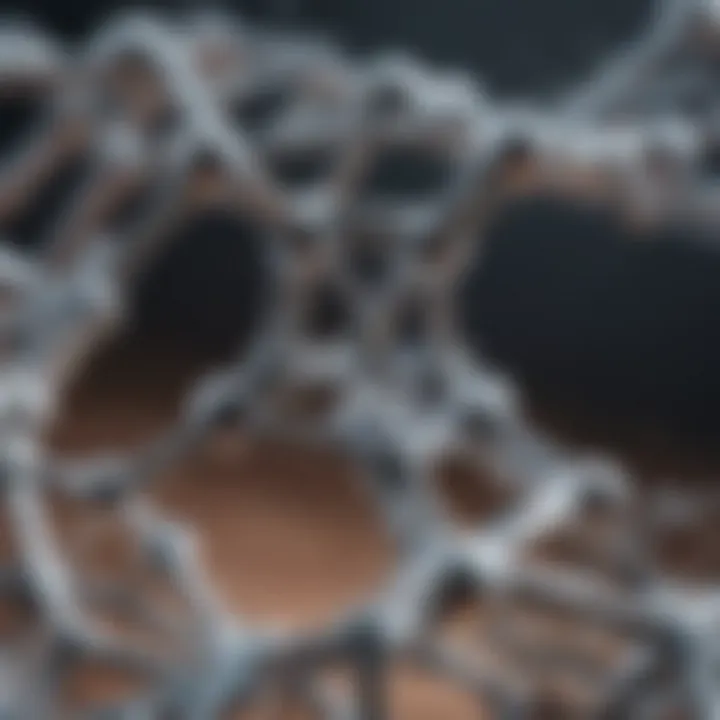
Intro
Magnesium adenosine triphosphate, commonly written as Mg ATP, is more than just a molecule; it’s a linchpin in the intricate machinery that drives life itself. Often overshadowed by its more famous counterpart, ATP, the presence of magnesium ions can significantly alter the functionality and efficiency of ATP, impacting everything from energy transfer to cellular signaling. Understanding the nuances of Mg ATP is crucial for students, researchers, educators, and professionals eager to grasp the complex web of biological processes.
The interaction between magnesium and ATP forms a vital partnership. Researchers have shown that Mg ATP participates in various cellular processes, from muscle contractions to nerve impulses. This has profound implications not only for our understanding of biochemical pathways but also for developments in medical and therapeutic interventions. Magnesium, though often underestimated, plays an essential role that can’t be overlooked.
The exploration of Mg ATP encompasses a multitude of themes, including the significance of magnesium ions in energy transduction and the enzymatic reactions fundamental to metabolism. In addition, it opens the door to understanding the often-overlooked consequences of magnesium deficiency, which can lead to a cascade of health issues. As we dig deeper, we’ll outline the critical findings about Mg ATP, its applications, and the methodologies that have shaped our current understanding.
"Understanding the partner dynamics between magnesium and ATP leads to richer insights into biological systems."
Research Highlights
Key Findings
- Integral Role in Energy Transfer: Mg ATP is necessary for the phosphorylation processes that release energy. Magnesium stabilizes ATP, allowing it to effectively participate in biological reactions.
- Enzymatic Reactions: Many enzymes require Mg ATP as a cofactor, enhancing their activity and specificity, which is critical in metabolic pathways.
- Cell Signaling: Mg ATP functions as a secondary messenger in signaling pathways, influencing cell communication and responses.
- Health Implications: A deficiency in magnesium can disrupt the homeostasis of Mg ATP, leading to metabolic dysfunctions.
Implications and Applications
The implications surrounding Mg ATP extend into various domains, including clinical therapy and nutritional science. For instance, recognizing the essential role of magnesium could guide therapeutic strategies for conditions associated with metabolic disorders. Moreover, the direct relationship between magnesium levels and ATP functionality raises questions about dietary intake and its management in chronic diseases.
Methodology Overview
Research Design
The research surrounding Mg ATP typically combines biochemical techniques with advanced imaging. It involves synthesizing Mg ATP under controlled conditions to study its behavior in different biological systems.
Experimental Procedures
- In Vitro Studies: Laboratory experiments allow researchers to observe the direct effects of Mg ATP on enzymatic activities and energy transfer.
- Cell Cultures: Utilizing various cell lines, including muscle and neuronal cells, researchers can study the impacts of magnesium on ATP-related processes.
- Animal Models: These models provide insight into how Mg ATP functions within a whole organism, allowing the exploration of systemic effects of magnesium deficiency.
Understanding these intricate roles of Mg ATP propels a foundation for future research, ultimately informing practices in health and science.
Preamble to Magnesium and ATP
Understanding the fundamental roles of magnesium and adenosine triphosphate (ATP) extends well beyond mere academic curiosity; it dives deep into the very core of biochemistry. In this article, we explore the intricate relationship between these two critical components and how they underpin various biological processes that sustain life. Magnesium acts not just as a cofactor for many enzymes, it also plays a pivotal part in stabilizing ATP, which in turn functions as the cell's primary energy currency.
The significance of this topic lies in its multifaceted implications. For one, it provides insight into cellular energy metabolism, essential for students, researchers, and professionals delving into biochemistry and related disciplines. Particularly, the efficiency of ATP usage is heavily influenced by magnesium. When magnesium is present in appropriate levels, ATP's energy release and utilization become more effective, showcasing the synergistic relationship between these molecules.
Moreover, the discussion prompts critical considerations regarding magnesium sufficiency for optimal ATP function. Inadequate magnesium levels can lead to impaired energy metabolism, ultimately resulting in health complications. Thus, understanding magnesium's role is not merely academic; it's vital for promoting health and well-being.
Fundamental Concepts
Definition of ATP
Adenosine triphosphate, or ATP, is often described as the molecular unit of currency for energy transfer within cells. Its structure, characterized by a ribonucleotide base, a sugar, and three phosphate groups, uniquely allows it to store and release energy through the breaking and forming of chemical bonds. ATP's role as an energy carrier is unparalleled; it facilitates various biological functions essential for life.
One key characteristic of ATP is its high-energy phosphate bonds. When one of these bonds, particularly the bond between the second and third phosphate group, is broken, a substantial amount of energy is released. This energy release is not just efficient; it’s also a highly beneficial feature for organisms that rely on rapid energy transfer for metabolic processes.
A unique feature of ATP lies in its versatility. It can power reactions involved in muscle contractions, nerve impulse propagation, and even biochemical synthesis. However, this benefit comes with the disadvantage that ATP is not stable in the long term; it needs to be continuously replenished under physiological conditions.
Role of Magnesium in Biochemistry
Magnesium is often overlooked yet is fundamentally important in biochemistry. It serves several vital functions, one of which includes acting as a cofactor required for numerous enzymatic reactions. In many cases, magnesium ions stabilize ATP, thus enhancing its overall functionality within cells.
A key characteristic of magnesium is its ability to form complexes with ATP. This relationship allows the enzyme ATPase to hydrolyze ATP efficiently, further demonstrating how magnesium enhances ATP’s usability. Without magnesium, the roles of ATP in energy transfer would be compromised, fundamentally affecting metabolic processes on a cellular level.
The unique combination of magnesium and ATP creates an environment conducive to various metabolic pathways. Their interaction not only fosters proper enzymatic activity but also influences cellular signaling pathways significantly. An important advantage here is that magnesium facilitates improved efficacy of ATP in energy transfer, but it also raises concerns in scenarios of deficiency; magnesium levels must be carefully maintained to ensure optimal ATP function.
Historical Perspectives
Discovery of ATP
The discovery of ATP marks a seminal moment in biochemistry, as this molecule serves as a cornerstone for understanding energy transfer in living organisms. First identified in 1929 by the scientist Karl Lohmann, ATP was hailed for its role in cellular processes. This discovery laid the groundwork for researching cellular metabolism and its intricate relationship with energy.
A significant characteristic of this discovery is its broad implications across various fields, including biochemistry, molecular biology, and physiology. Understanding the structure and function of ATP has been pivotal in unraveling complex metabolic pathways and the way cells generate and utilize energy.
The unique feature of ATP, as an energy carrier, has actually transformed biochemical research. It’s prevalent in nearly every living cell, from simple bacteria to complex multicellular organisms. The discovery of ATP enabled scientists to establish cellular respiration's fundamental mechanisms. However, this critical development also illuminated the need for further investigations into the molecular underpinnings of energy metabolism, hence, raising a host of questions that remain the focus of ongoing research.
Evolution of Understanding Magnesium's Role
The understanding of magnesium's significance evolved gradually, particularly in relation to ATP. Initially deemed an innocuous mineral, magnesium was only recognized as an essential nutrient later, with studies from the mid-20th century highlighting its biological importance.
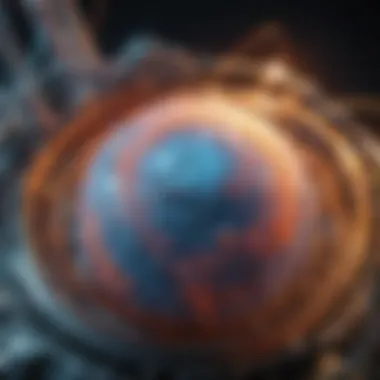
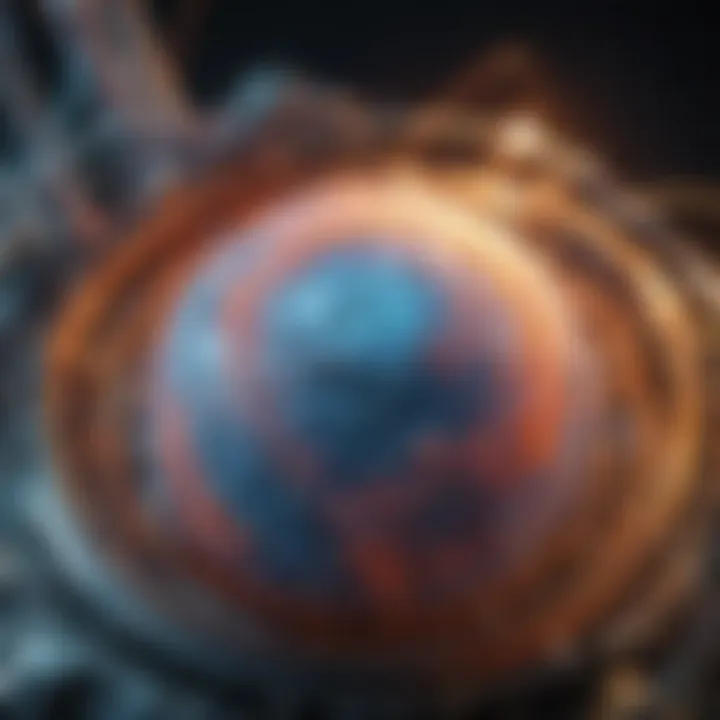
A pivotal aspect of this evolution is the eventual recognition of magnesium as a vital cofactor in enzymatic reactions involving ATP. As research unfolded, it became evident that magnesium not only activates numerous enzymes but is also critical for stabilizing ATP molecules, thus enabling their functionality.
The unique insight into magnesium's role prompted a shift in research focus towards its implications in metabolic health, suggesting that magnesium insufficiency could impair ATP function. The ongoing exploration into magnesium's undervalued role reflects a growing appreciation of interconnections in biochemistry, prompting inquiries that could lead to breakthroughs in understanding metabolic disorders and avenues for therapeutic interventions.
Chemical Properties of Mg ATP
The chemical properties of magnesium adenosine triphosphate (Mg ATP) are not just essential to understand the molecule itself, but they also cast a wide net over various biological processes. This section dives into the intricacies of its molecular and physical attributes, illustrating why Mg ATP is often considered the linchpin in several biochemical mechanisms. Understanding these properties is crucial for demonstrating the role of Mg ATP in energy dynamics and metabolic reactions.
Molecular Structure
ATP and its Phosphate Groups
Adenosine triphosphate comprises a nucleotide with three phosphate groups, which are the true powerhouses for energy release. The key characteristic of ATP is its high-energy phosphoanhydride bonds. When these bonds are broken, usually during hydrolysis, significant energy is released. This feature makes ATP not just favorable but indispensable for cellular processes.
One unique aspect here is the ability of the phosphate groups to repulse one another due to their negative charges. This inherent tension gives rise to a wealth of energy, making ATP an effective energy currency.
- Key advantages:
- High energy yield: Provides immediate energy for various cellular functions.
- Versatility: Can be utilized in multiple pathways like glycolysis and oxidative phosphorylation.
However, the very property that makes ATP an energy reservoir also leads to its instability. Once its phosphate bonds break, ATP rapidly converts to adenosine diphosphate (ADP), necessitating a constant regeneration via cellular metabolism.
Magnesium Coordination in ATP
Magnesium plays a critical role in stabilizing ATP’s structure. It coordinates with the negatively charged oxygen atoms in the phosphate groups, which mitigates their repulsive forces and enhances ATP’s stability in biological systems. This interaction is vital as it plays a part in ensuring that ATP remains an effective fuel for energy transfer.
- Key point: The Mg-ATP complex is favored as it not only serves to protect the energy-rich phosphoanhydride bonds but also aids in enzymatic reactions.
The advantage of this coordination is twofold; it reinforces the structural integrity of ATP while also ensuring that the energy release occurs in a regulated manner. The presence of magnesium makes Mg ATP a robust participant in many biological reactions, as it effectively modulates ATP's affinity toward various enzymes.
Physical Characteristics
Solubility and Stability
Mg ATP showcases notable solubility in biological fluids, enabling it to effectively engage in intramolecular exchange as well as intermolecular interactions. Its solubility is a key property as it ensures that ATP can be readily available in various cellular compartments, assisting in quick energy transactions.
A significant feature of Mg ATP is that its stability parallels its solubility. While it can dissolve in water, it maintains a relatively stable structure in physiological conditions. This characteristic is vital because if ATP were instable, cellular processes reliant on ATP could face disruption, leading to inefficient energy transfer and metabolism.
- Advantages:
- ** Essential for flow of energy:** Maintains a balance between being available but not too reactive.
- Survivability in diverse environments: Can perform well under varying conditions within the cell.
However, challenges arise during high-energy demands, as excessive instability could hinder cellular processes when ATP levels diminish too rapidly.
Interactions with Other Molecules
Mg ATP is not a lone actor in the cellular milieu. It engages in complex interactions with various biomolecules, including proteins and nucleic acids. This interaction is crucial for enzymatic activities where Mg ATP serves as a cofactor, facilitating binding to substrates and modifying enzyme activity.
- Salient features:
- Cofactor Role: Acts alongside enzymes in metabolic pathways, making it a vital partner in sustaining life.
- Signaling Functionality: Magnesium-bound ATP plays critical roles in signal transduction pathways, enhancing the efficacy of signaling molecules.
The ability of Mg ATP to participate in these interactions showcases its versatility. It helps in maintaining cellular equilibria while also communicating signals that govern cell operations. However, factors like concentration and competitive binding with other ions can influence these interactions, potentially leading to dysregulated cellular functions.
Understanding the chemical properties of Mg ATP offers tremendous insights into its critical roles in metabolism and energy transfer, highlighting its importance in maintaining life as we know it.
Biological Significance of Mg ATP
The role of magnesium adenosine triphosphate, or Mg ATP, can't be overstated when you look at its biological significance in living systems. It's not just another molecule; it's the linchpin of various crucial processes. Mg ATP isn't merely a source of energy; instead, it serves as a central player in energy metabolism, catalyzing reactions that form the foundation of life itself.
Understanding Mg ATP brings to light how organisms harness energy from their surroundings. This energy currency, as we’ll explore more fully later, is essential for processes ranging from muscle contraction to cellular replication. Magnesium’s presence in this equation is vital; it influences many biochemical processes, ensuring that energy release and utilization occur smoothly and efficiently.
Role in Cellular Energy Transfer
ATP as an Energy Currency
ATP is often referred to as the energy currency of the cell. This designation really hits the nail on the head. In simple terms, ATP stores energy in its high-energy phosphate bonds, ready to be used when the cell needs it. Whenever a cell requires a burst of energy, it breaks down ATP, releasing energy in the process.
One key characteristic of ATP is its relatively simple and rapid conversion to ADP (adenosine diphosphate) and inorganic phosphate. This quick turnaround is what makes ATP such an attractive choice for cellular energy needs. Unlike other energy molecules, ATP provides a readily accessible form of energy, capable of being regenerated quickly through various cellular processes.
Also interesting is how ATP's structure allows it to be easily transported within the cell. It’s like currency that you can carry with you anywhere, which makes it incredibly versatile and essential for various biochemical pathways. However, this mechanism isn't without drawbacks. While ATP is efficient, the process of regenerating it from ADP is energy-intensive itself, requiring coupling with other processes like glucose metabolism or oxidative phosphorylation.
Magnesium's Influence on Energy Release
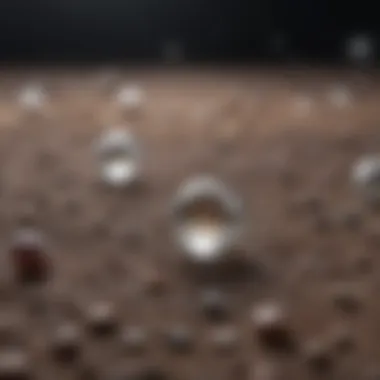
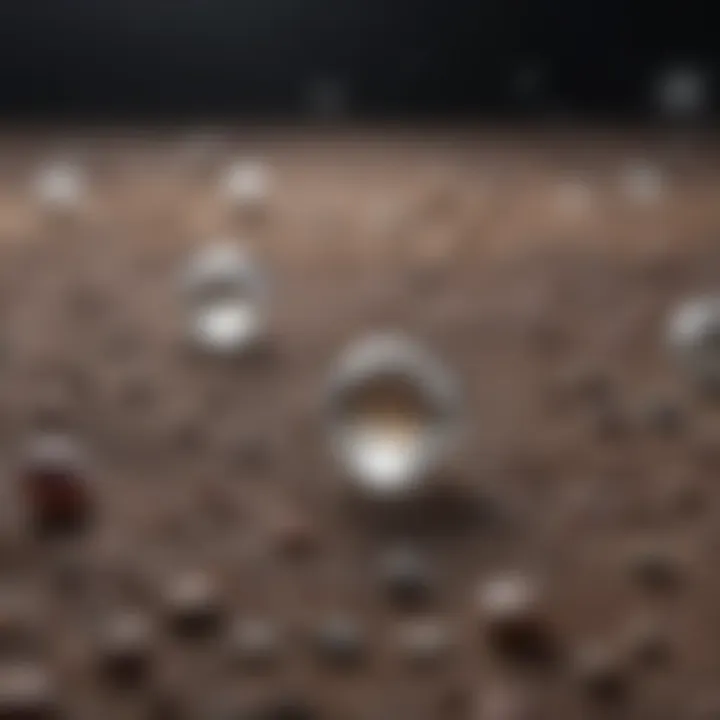
Now, what about magnesium’s role in all this? Well, magnesium ions are indispensable in stabilizing the ATP molecule. Without magnesium, ATP might just be a fleeting whisper, lacking the stability necessary for effective biological activity. Magnesium binds to ATP, forming a complex that facilitates the energy transfer process. This relationship is critical because it enhances the molecule's structural integrity, making it functional in energetic exchanges.
What stands out with magnesium’s influence on energy release is its ability to modulate enzyme activities involved in ATP use. For instance, many ATP-using enzymes, like kinases, will only operate optimally in the presence of sufficient magnesium. Thus, while ATP generates energy, magnesium fine-tunes the process. If magnesium levels drop, it's like trying to run a car on an empty tank; performance sputters and experiences delays.
Enzymatic Functions
Mg ATP as a Cofactor
The role of Mg ATP as a cofactor is pivotal in enzymatic functions throughout cellular metabolism. Cofactors are non-protein chemical compounds that assist enzymes in catalyzing reactions. In the case of Mg ATP, it serves as a crucial cofactor for numerous enzymes that facilitate metabolic reactions.
A critical feature of Mg ATP as a cofactor is its ability to affect enzyme activity. For many enzymes, the presence of Mg ATP alters the shape and charge distribution, making it easier for substrates to bind and undergo chemical transformations. This characteristic is not just beneficial; it ensures that metabolic processes occur at the right speed and volume necessary for sustaining life.
On the flip side, if there’s an excess or a deficiency of magnesium, the enzymatic reactions can be thrown off balance. This fluctuation underscores the delicate relationship between enzyme activity and cofactor availability, which can significantly affect cellular function.
Impact on Metabolic Pathways
Looking at the impact on metabolic pathways, Mg ATP’s involvement isn’t merely peripheral; it’s central. This molecule participates in various metabolic pathways, impacting how cells generate energy from nutrients. For instance, in glycolysis, Mg ATP is essential for initiating the breakdown of glucose, which is a primary energy source.
The striking feature of Mg ATP in metabolic pathways is its regulatory capacity. It signals other pathways, influencing how cells prioritize energy usage based on physiological needs. In times of energy demand, for instance, cells can shift resources accordingly, favoring pathways that will boost ATP production. However, this fluid balance can also be disrupted by stressors, leading to metabolic disorders when not properly managed.
The Relationship Between Magnesium and ATP Synthesis
Understanding the connection between magnesium and ATP synthesis is crucial in the realm of biochemistry. This relationship stems not just from magnesium being a cofactor, but also as a facilitator in the intricate mechanics of ATP production. Magnesium ions play a pivotal role by stabilizing the negative charges of ATP, which affects how ATP interacts in various enzymatic processes. Without an adequate supply of magnesium, the synthesis and, consequently, the efficiency of ATP production can take a serious hit, indirectly influencing numerous metabolic pathways.
ATP Formation Mechanisms
Oxidative Phosphorylation
Oxidative phosphorylation is a central process in cellular respiration, where ATP is generated through the electron transport chain. This method stands out due to its efficiency in yielding large amounts of ATP per glucose molecule. The combination of the electron transport chain and ATP synthase relies heavily on the presence of magnesium ions.
The key characteristic of oxidative phosphorylation is that it utilizes a proton gradient, created by electrons moving through the chain, to drive ATP production. What sets it apart from other methods is its ability to produce up to 34 ATP molecules during aerobic respiration. This highlights why oxidative phosphorylation is often the go-to process for producing energy at a scale necessary for complex organisms.
Nonetheless, there’s a unique feature here: the role of magnesium in stabilizing ADP and inorganic phosphate during this process is essential. Without magnesium, ATP synthase cannot function optimally, limiting ATP production. However, this method does require oxygen, and thus, organisms that rely solely on anaerobic methods won’t benefit from oxidative phosphorylation.
Substrate-level Phosphorylation
Substrate-level phosphorylation is another means of producing ATP, but it operates quite differently from oxidative phosphorylation. This method occurs directly in the reaction pathways—most notably in glycolysis and the Krebs cycle—where a phosphate group is directly transferred to ADP from a phosphorylated intermediate.
What makes substrate-level phosphorylation appealing is its immediacy. It does not need a proton gradient or oxygen, making it accessible in anaerobic conditions. While it yields fewer ATP molecules per glucose when compared to oxidative phosphorylation (only about 2 to 4 ATP molecules), it provides a rapid response to energy needs, which can be critical in low-oxygen situations.
A notable feature of this method is that it does not rely on magnesium directly for reacting substrates. However, magnesium remains an important element within cellular metabolism, modulating enzyme activities that participate in these pathways, ensuring a delicate balance between ATP supply and demand.
Magnesium's Role in ATP Production
Magnesium-Induced Conformational Changes
Magnesium-induced conformational changes pertain to the alterations that occur in enzyme structures in response to magnesium binding. This phenomenon is important, especially in ATP metabolism, where many enzymes require magnesium to interact with ATP effectively. This interaction stabilizes the enzyme-substrate complex, making it easier for the reaction to proceed.
This characteristic of magnesium is vital because, without these conformational changes, enzymes that enable ATP synthesis (like ATP synthase or various kinases) may not function properly. The benefits are clear: with magnesium facilitating these changes, enzymatic reactions become more efficient, increasing overall ATP production. However, this dependency on magnesium means that fluctuations in magnesium levels can hinder enzyme performance, underscoring the need for balanced magnesium availability in biological systems.
Regulatory Effects of Magnesium Levels
Regulatory effects of magnesium levels have significant implications for ATP production and overall cellular health. Magnesium levels dictate many cellular activities, including those involved in ATP synthesis. An increase or decrease in magnesium ions can profoundly affect metabolic rates and energy production.
The main point here is that magnesium acts as a signaling molecule. When magnesium levels are optimal, energy pathways operate smoothly. But, too low magnesium levels may lead to impaired ATP synthesis directly, while excessively high levels can be toxic, disrupting cellular homeostasis. The unique aspect of magnesium's regulatory role is its ability to modulate several pathways at once, ensuring that various tissues maintain energy balance in response to physiological demands. However, too much dependency on magnesium regulation also presents a challenge—if magnesium levels dip, the downstream effects can quickly cascade into systemic failures.
"The binding of magnesium to ATP is not merely a stabilizing force but a form of communication within the cell's biochemical network."
Physiological Implications of Mg ATP Deficiency
The role of magnesium adenosine triphosphate (Mg ATP) reaches far beyond mere energy supply; it is central to maintaining physiological balance. When Mg ATP levels dwindle, the body starts to feel the pinch. This section sheds light on the significant ramifications of Mg ATP deficiency, illustrating not just the symptoms that emerge but also potential long-term health threats that could arise from sustained deficiencies.
Health Consequences
Common Symptoms of Deficiency
When magnesium concentrations drop, a variety of symptoms may surface. The common symptoms of deficiency are often not immediately connected to magnesium levels, making them insidious. Feeling fatigued is often the first clue. As ATP levels dip, the energy reservoirs necessary for daily functioning dwindle. Muscle cramps, spasms, and even abnormal heart rhythms can occur.
- These symptoms manifest varying levels of discomfort and interruption in daily life. People report feelings of tiredness or an odd sense of weakness.
- Notably, insomnia can also arise; when your body lacks magnesium, it might struggle to rest effectively, perpetuating the cycle of exhaustion.
"Magnesium impacts a range of physical processes, hence its deficiency can lead to widespread symptoms."
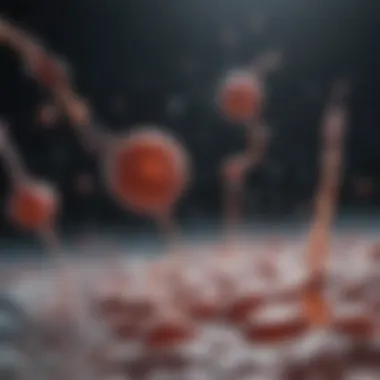
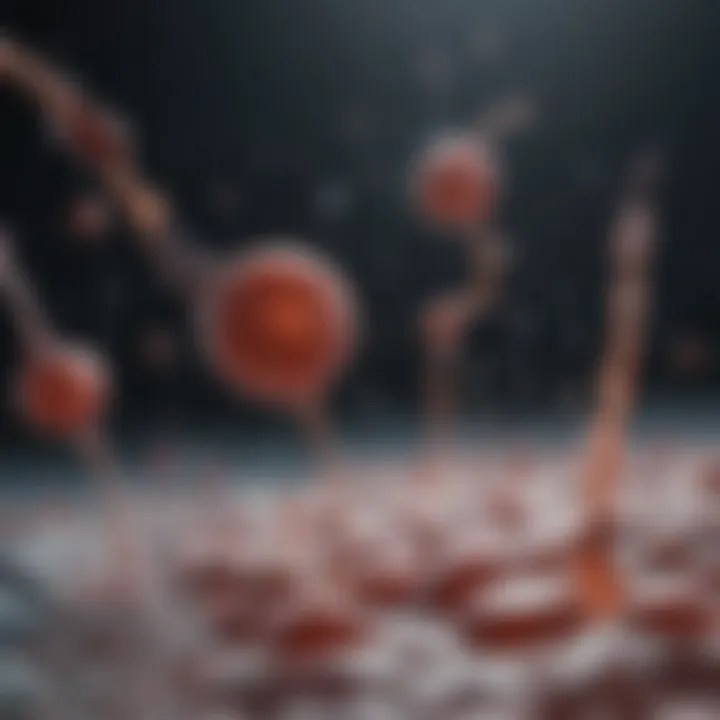
The unique feature of recognizing these common symptoms lies in how they can often be mistaken for unrelated issues. For instance, a person might chalk their fatigue up to a busy lifestyle without realizing it’s a symptom of underlining magnesium deficiency.
Long-term Health Risks
Delving into long-term health risks, the potential repercussions of Mg ATP deficiency take a more concerning turn. Prolonged magnesium deficiency can predispose individuals to chronic conditions such as high blood pressure, diabetes, and osteoporosis. These health issues stem from the ongoing lack of energy management and impaired cellular functioning due to insufficient Mg ATP.
- For one, there’s an observable connection between low magnesium levels and an increased risk of cardiovascular diseases. Insufficient Mg ATP is linked with lowered regulation of vascular tension.
- Moreover, the long-term effects can compound over time, leading to a reduction in the quality of life—a slow but steady downward slope.
Similar to common symptoms, the unique feature of long-term health risks is they often develop insidiously. What starts as minor fatigue can snowball into something much more serious, effectively wreaking havoc on an individual’s overall well-being. Thus, one can argue that preventative awareness is paramount in addressing potential Mg ATP deficiency.
Impact on Disease Processes
Exploring the implications of low Mg ATP levels extends into more specific disease processes, where the role of magnesium is highlighting even further.
Magnesium's Role in Metabolic Disorders
Here, magnesium assumes a critical role in metabolic disorders. It’s often overlooked how magnesium cofactor functions are integral in metabolic reactions, including those related to glucose and insulin sensitivity. Consequently, low Mg ATP levels can lead to insulin resistance, making one susceptible to conditions like Type 2 diabetes.
- A key characteristic of metabolic disorders associated with deficient magnesium levels is a downtrend in metabolic flexibility. The body’s ability to switch from burning its fat reserves to utilizing carbohydrates diminishes, leading to ineffective energy use.
- The advantage of focusing on magnesium's role emphasizes addressing the underlying metabolic pathways. By improving Mg ATP levels, individuals can potentially enhance their metabolic health.
Influence on Cardiovascular Health
As one of the most critical areas affected, the influence on cardiovascular health cannot be overstated. Magnesium’s necessity for heart health is supported by its role in regulating heart rhythm and vascular tone. When Mg ATP levels falter, so too does heart function. Low magnesium is linked to increased incidents of arrhythmias and hypertension, as the heart struggles to maintain normal electrical activity without adequate fuel.
- The key characteristic that makes this aspect notable is the relationship between magnesium status and cardiovascular outcomes. Studies have demonstrated that sufficient magnesium contributes to reductions in blood pressure, thus mitigating risks of heart disease.
- The unique feature in analyzing cardiovascular health reflects how magnesium supplementation could be a preventative strategy against cardiovascular diseases. This interaction thus intensifies the argument for attention on dietary magnesium intake, especially in at-risk populations.
Research Developments and Future Directions
The study of magnesium adenosine triphosphate (Mg ATP) reflects the dynamic nature of research in biochemistry and cell biology. As scientists delve deeper into cellular processes, the role of Mg ATP continues to emerge as a cornerstone of various biological phenomena. The relevance of this topic garners significant interest, not just for its theoretical implications but also for practical applications in medicine and health.
Recent Studies on Mg ATP
Investigating Magnesium's Role in Cellular Aging
In recent years, scientists have been probing into how magnesium influences aging at a cellular level. This area of study has gained traction due to the pivotal function Mg ATP performs in fundamental metabolic processes. As cells age, the efficiency of energy metabolism can decline, potentially leading to various age-related ailments. Magnesium's role becomes critical in maintaining ATP production, thus influencing cellular longevity.
One key characteristic of exploring magnesium's role in cellular aging is the emerging link between magnesium homeostasis and oxidative stress. Studies suggest that magnesium deficiency can exacerbate oxidative damage, leading to faster cellular aging.
Investigating magnesium in this context offers a promising pathway for developing strategies to mitigate age-related health issues. A notable benefit is that it opens the door to nutritional interventions or supplements aimed at improving magnesium levels and, consequently, ATP availability. However, a challenge lies in accurately measuring cellular magnesium levels in living organisms, which can complicate research outcomes.
Exploring Therapeutic Applications
Another promising area involves exploring therapeutic applications of Mg ATP. Research is focusing on how Mg ATP can be harnessed in treating various conditions. For instance, it is being investigated in relation to metabolic disorders such as type 2 diabetes and heart disease. This direction signifies a favorable intersection of basic science and clinical application, making it a popular choice among researchers.
The therapeutic potential hinges on magnesium's role in stabilizing ATP, which is vital for cellular functions. It has been observed that adequate magnesium levels can enhance insulin sensitivity and improve cardiovascular health, providing key insights into potential treatment avenues. The unique feature of this research is its dual focus: not only does it examine disease treatment, but it also emphasizes prevention through dietary and lifestyle modifications. While the potential is vast, challenges remain, particularly in clinical settings where consistent magnesium supplementation protocols are yet to be established.
Potential Areas of Inquiry
New Technologies in Research
The advent of new technologies is paving the way for more nuanced research into Mg ATP. Cutting-edge techniques, such as CRISPR genome editing and advanced imaging methods, provide platforms that allow scientists to explore the intricate details of magnesium’s role in ATP synthesis and overall cellular function. These technologies enhance the reliability of research outcomes, leading to a more sophisticated understanding of Mg ATP dynamics.
The characteristic of being able to visualize changes in real-time makes this approach particularly beneficial. It allows researchers to witness the immediate structural or functional effects of magnesium on ATP in live cells. However, the complexity of these technologies may require significant training and investment, which could slow down broader adoption in the research community.
Interdisciplinary Approaches to Study Mg ATP
An intriguing emerging trend is the interdisciplinary approach towards studying Mg ATP. This approach integrates perspectives from biochemistry, molecular biology, and even fields like nutrition science and pharmacology. By bridging these disciplines, researchers aim to develop a holistic understanding of magnesium's role.
This methodology is beneficial as it encourages collaboration among experts from various fields, fostering innovation and comprehensive exploration of Mg ATP. The unique feature of this approach lies in its capacity to combine theoretical knowledge with practical applications, tracking how dietary magnesium impacts ATP levels and overall health. Nonetheless, one major challenge could involve reconciling varying terminologies and methodologies from different fields, potentially complicating communication across disciplines.
"Understanding Mg ATP not only illuminates its biochemical significance but also offers avenues for practical health interventions, shaping the future of clinical nutrition and therapies."
Culmination
Understanding the multifaceted role of magnesium adenosine triphosphate (Mg ATP) in biological systems is not just an academic endeavor; it carries profound implications for both basic research and practical applications. As the article highlights, Mg ATP serves as a cornerstone in cellular energy transfer, enzymatic interactions, and the regulation of numerous biochemical pathways. These aspects underscore its centrality in sustaining life processes, making its study essential in the realm of biochemistry and medical research.
The relationship between magnesium and ATP is particularly intriguing. Magnesium ions influence ATP's stability and functionality, ensuring that energy can be efficiently harnessed and utilized by cells. This synergy speaks volumes about how tightly knitted these components are within the grand tapestry of biological systems. Furthermore, recognizing the consequences of Mg ATP deficiencies can aid in diagnosing and treating various health issues, ranging from metabolic disorders to cardiovascular problems.
Summary of Key Points
- Essential Role of Mg ATP: It highlights Mg ATP's function in energy transfer, serving as a mediator of vital processes that keep cellular activities running smoothly.
- Magnesium's Influence: The interaction between magnesium and ATP is crucial for numerous biochemical activities, affecting cellular signaling and metabolic pathways.
- Health Implications: Magnesium deficiency can lead to serious health consequences, emphasizing the need for awareness and potential therapeutic interventions.
"The dynamics of magnesium adenosine triphosphate in cellular processes not only illuminate fundamental biological questions but also pave the way for novel therapeutic strategies."
The Future of Mg ATP Research
Looking ahead, research into Mg ATP holds promise for uncovering new insights that might transform our understanding of biological systems. Emerging technologies, such as CRISPR and advanced imaging, are poised to reveal the intricacies of Mg ATP interactions within cells. Such exploration can lead to breakthroughs in identifying disease mechanisms and developing targeted therapies.
There is also a growing interest in interdisciplinary approaches, combining fields like biochemistry, nutrition, and systems biology to study the interrelated roles of magnesium and ATP more comprehensively. Understanding the broader impacts of Mg ATP on health will be crucial as researchers tackle complex challenges, such as aging and chronic diseases, through innovative strategies. Optimizing magnesium levels in clinical settings might become a critical component in enhancing patient care and outcomes, making this line of inquiry both timely and necessary for future health advancements.