Exploring Biochemistry: Insights from the Fifth Edition
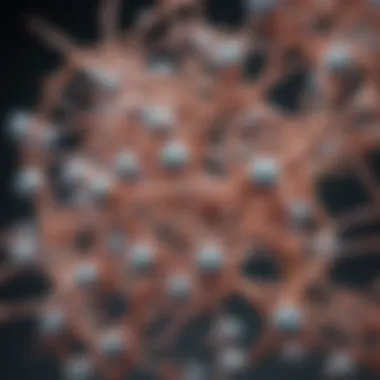
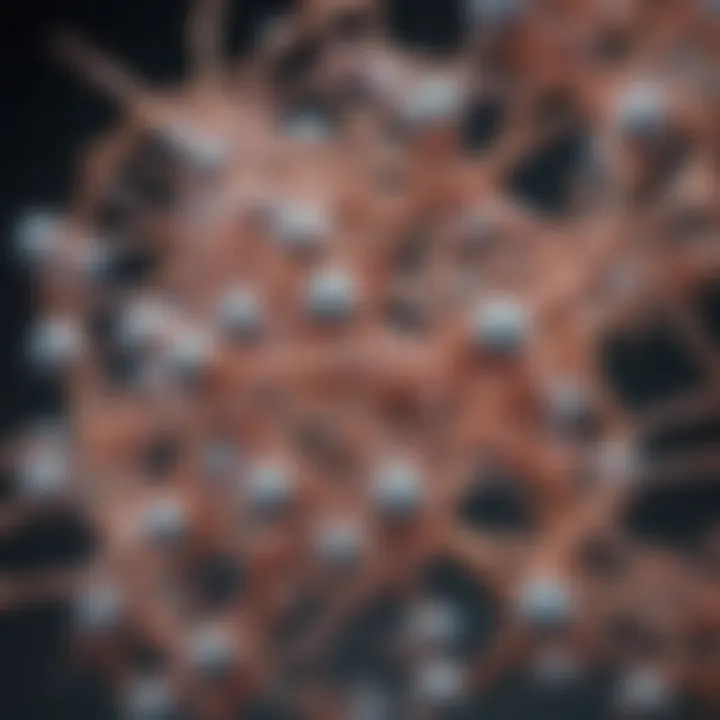
Intro
Biochemistry serves as a bridge between biology and chemistry, unraveling the complex chemical processes that dictate the functions of living organisms. The fifth edition of 'Principles of Biochemistry' has reaffirmed its position as a cornerstone resource for students, educators, and professionals alike. This article aims to highlight essential concepts, methodologies, and real-world applications presented in this latest edition. The synthesis of knowledge from diverse biochemical pathways enriches our understanding of life itself, guiding research and education in significant ways.
Research Highlights
Key Findings
The fifth edition of 'Principles of Biochemistry' marks a substantial advancement in the presentation and understanding of biochemical concepts. Among its key findings, notable topics such as enzyme kinetics, metabolic pathways, and molecular biology take precedence. The integration of updated research findings provides clarity and depth, appealing to an audience eager for precise information.
Some key findings include:
- Detailed exploration of enzyme mechanisms and their regulatory roles.
- Enhanced understanding of metabolic networks and their interconnectivity.
- Insights into molecular structures such as proteins and nucleic acids.
The book emphasizes that biochemical knowledge is not static; it constantly evolves with new discoveries and technologies.
Implications and Applications
The implications of the findings presented in the fifth edition are vast. Understanding biochemical principles is not only fundamental for academic endeavors but also for practical applications in research and industry. For example, advancements in drug design and biotechnology heavily rely on the biochemical principles elucidated in this text. The knowledge acquired facilitates the development of innovative therapeutic strategies and novel diagnostic tools.
Methodology Overview
Research Design
Research in biochemistry is characterized by a blend of experimental approaches and theoretical frameworks. The fifth edition details various research designs used in biochemistry, including both in vitro and in vivo studies. These designs provide frameworks through which researchers can explore biochemical phenomena comprehensively.
Experimental Procedures
The experimental procedures outlined in the fifth edition are methodical and rigorous. Important techniques such as chromatography, mass spectrometry, and PCR are discussed in detail. These methods aim to analyze and manipulate biological molecules, thus propelling biochemistry forward.
Experimental approaches include:
- Chromatography for separation and analysis of biochemical compounds.
- Mass spectrometry for molecular identification and characterization.
- Polymerase chain reaction for amplifying DNA sequences.
By highlighting these methods, the fifth edition serves as an essential guide for practitioners seeking to employ these techniques in their research.
Finale
The fifth edition of 'Principles of Biochemistry' provides a rich repository of knowledge essential for understanding biochemical processes. The insights gathered from this edition enhance educational efforts and contribute to ongoing scientific advancements. Through a thorough examination of research highlights and methodology, this article serves to reinforce the significance of biochemistry in both academic and applied contexts.
Prolusion to Biochemistry
Biochemistry is a vital field that bridges biology and chemistry, making it essential for understanding life at the molecular level. This article aims to explore the significant principles outlined in the latest edition of 'Principles of Biochemistry.' The understanding of biochemistry presents a depth of insight, which informs innovations and healthcare advancements. This field not only enriches academic learning but also informs practical applications that affect our daily lives and overall health.
Defining Biochemistry
Biochemistry is defined as the study of biological processes at the molecular and cellular level. It focuses on the chemical substances and vital processes occurring in living organisms. This includes studying molecules such as proteins, nucleic acids, carbohydrates, and lipids. Each of these biomolecules is crucial for life, serving functions from structural support to energy storage and transmission of genetic information.
In many ways, the study of biochemistry allows us to grasp how living systems operate. It examines how biochemical pathways fuel cellular functions and how these processes can be altered in disease states. A clear definition of biochemistry emphasizes the interdisciplinary nature of this science, revealing its influence across fields such as medicine, genetics, and environmental science.
Historical Context
To appreciate biochemistry, it is important to understand its historical development. The roots of biochemistry can be traced back to the early days of biology and chemistry. Key milestones include the discovery of enzymes and the elucidation of DNA structure. For example, the advent of methods to analyze biochemical pathways in the early 20th century marked a significant turning point in life sciences.
Moreover, the mid-1900s saw groundbreaking advancements, such as the Watson and Crick model of DNA. This established a framework to comprehend genetic information storage and transmission. Over the years, biochemistry has evolved, integrating emerging technologies and methodologies that deepen our understanding of complex biological processes. This historical perspective reveals how biochemistry has shifted from a descriptive science to a rigorous discipline that underpins modern biological research and applications.
"Biochemistry not only explains how living organisms function, but it also guides innovation in medical and environmental fields."
Through this overview of key definitions and historical context, we establish the groundwork for further exploration into the essential concepts and applications found in 'Principles of Biochemistry.' This examination will pave the way for understanding how biochemistry shapes our comprehension of life and informs numerous scientific advancements.
Key Concepts in Biochemistry
The exploration of key concepts in biochemistry serves as a fundamental pillar in understanding both the simplicity and complexity of biological systems. This section highlights important insights related to the structure and function of biomolecules and the intricacies of biochemical reactions. Each concept plays a role in unraveling the mechanisms that govern life. Understanding these principles allows readers to appreciate the nuances of biochemical processes and their implications in various scientific fields, including medicine, genetics, and biotechnology.
Structure and Function of Biomolecules
Biomolecules are the vital building blocks of life. Their structures relate directly to their functions, making it essential to grasp the attributes of proteins, nucleic acids, carbohydrates, and lipids.
Proteins
Proteins are large macromolecules pivotal to nearly every biological process. Their unique structure, consisting of amino acid chains folded into complex shapes, gives them specific functions. For instance, enzymes, a type of protein, act as catalysts in biochemical reactions, significantly increasing the rate at which these reactions occur.
A key characteristic of proteins is their ability to form diverse structures through various combinations of amino acids. This versatility is why proteins are a popular choice in biochemistry. However, the complexity of protein folding can present challenges, especially in the understanding of diseases related to protein misfolding, such as Alzheimer’s. Therefore, while proteins are immensely beneficial, comprehending their structure-function relationship remains critical in both research and clinical settings.
Nucleic Acids
Nucleic acids, including DNA and RNA, carry genetic information essential for life. Their specific arrangement of nucleotides allows for the encoding, storage, and retrieval of genetic data. The double helix structure of DNA is especially notable, since it facilitates accurate DNA replication and transcription into RNA.
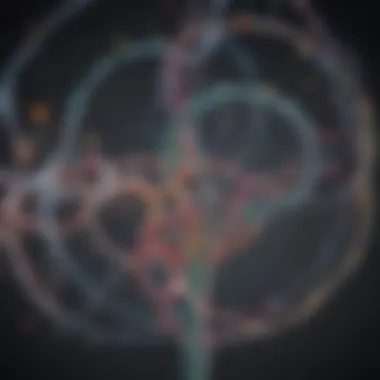
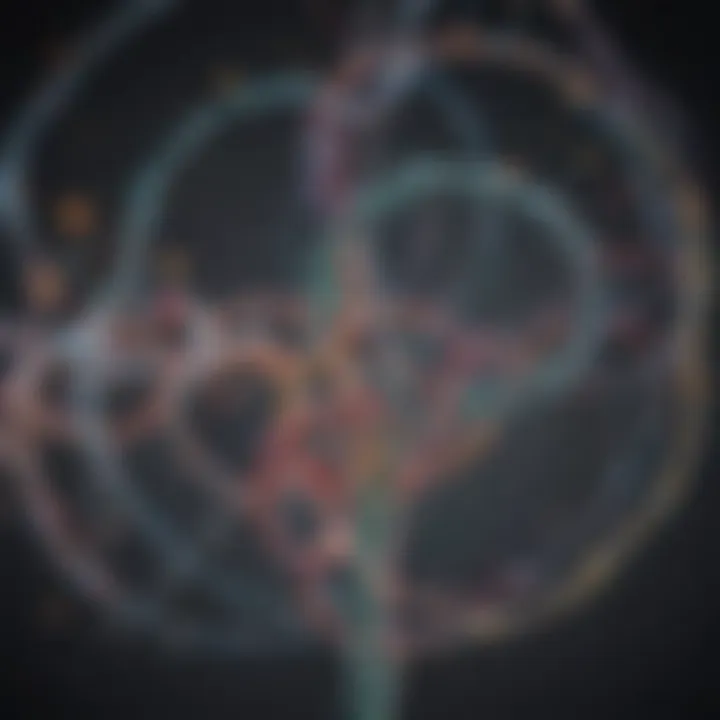
This unique feature makes nucleic acids indispensable in biochemistry, especially in fields such as genetics and molecular biology. However, their stability can be influenced by environmental conditions, which is a disadvantage when considering their practical applications in technologies like gene editing. Thus, understanding nucleic acids' roles contributes significantly to advancements in biotechnology and therapeutic developments.
Carbohydrates
Carbohydrates serve as primary energy sources and structural components in living organisms. Their composition, mainly comprising carbon, hydrogen, and oxygen, allows them to form various structures, from simple sugars to complex polysaccharides. This characteristic is crucial since carbohydrates can be quickly broken down to release energy when needed.
Their role as energy stores and their presence in cell membranes as signaling molecules make carbohydrates a beneficial topic in biochemistry. However, their advantages come with considerations regarding metabolism and its implications for health, particularly in the context of diabetes and obesity. Thus, carbohydrates require careful analysis in both nutritional biochemistry and health sciences.
Lipids
Lipids, including fats, oils, and phospholipids, play critical roles in energy storage and cellular structure. They are hydrophobic molecules, which means they do not mix well with water. This unique property is what allows lipids to form biological membranes, creating boundaries essential for cell function.
Lipids are important in understanding how energy is stored and utilized in organisms. They are also popular in biochemistry due to their involvement in metabolic pathways and signaling. However, high levels of certain lipids in the body can lead to health issues, such as cardiovascular diseases, highlighting the need for balance in lipid consumption.
Biochemical Reactions
Biochemical reactions encompass the transformative processes that drive metabolism and cellular functions. Each component—enzyme kinetics, metabolic pathways, and thermodynamics—illuminates a different aspect of these reactions.
Enzyme Kinetics
Enzyme kinetics examines the rates of enzyme-catalyzed reactions and provides insights into enzyme behavior under various conditions. This understanding is crucial for developing drugs and understanding metabolic disorders. The key characteristic of enzyme kinetics is the Michaelis-Menten equation, which describes the rate of enzymatic reactions concerning substrate concentration.
Enzyme kinetics is a beneficial focus because it allows researchers to model and predict reaction dynamics. However, it can be complex due to factors like enzyme inhibitors affecting reaction rates, which adds layers of consideration when applying this knowledge to pharmacology and biotechnology.
Metabolic Pathways
Metabolic pathways are interconnected series of chemical reactions occurring within a cell. They are essential for maintaining cellular functions, like energy production, and biosynthesis. Each pathway’s regulated nature is vital for homeostasis.
The organization of metabolic pathways makes them a popular subject in the article since they provide a framework for understanding the flow of energy and materials in living systems. Still, complexity arises when pathways overlap, leading to potential points of failure in metabolic regulation and implications for disease.
Thermodynamics in Biochemistry
Thermodynamics plays an essential role in biochemistry, as it deals with heat and energy transfer in chemical reactions. Understanding these principles helps comprehend how energy changes influence biochemical processes. The laws of thermodynamics highlight key concepts such as energy conservation and entropy, making them crucial in metabolic studies.
Thermodynamics is beneficial because it establishes a foundational framework for describing energy transfers in biochemical reactions. However, the mathematical nature of thermodynamics can complicate its application in biological systems, such as predicting reaction spontaneity without considering biological contexts. Thus, a nuanced understanding of thermodynamics is important for effectively applying these principles in biochemistry.
Advancements in Biochemical Techniques
The field of biochemistry continuously evolves, and advancements in biochemical techniques play a significant role in this progress. This section discusses various methods that have transformed the way biochemical analysis and research are conducted. These techniques are critical for researchers and practitioners, making them invaluable in understanding biological processes at a molecular level. They facilitate discoveries that contribute to health, environmental science, and various industrial applications.
Analytical Methods
Analytical methods provide the tools necessary for the separation, identification, and quantification of biomolecules. Each technique has distinct characteristics, advantages, and limitations, but they all contribute to a broader understanding of biochemical disciplines.
Chromatography
Chromatography is a vital technique widely used for purification and analysis of complex mixtures. It allows researchers to separate components based on their interactions with a stationary phase and a mobile phase. One key characteristic of chromatography is its versatility; it encompasses various methods, including gas chromatography and liquid chromatography.
The unique feature of chromatography lies in its ability to resolve components that are otherwise difficult to separate. For example, in protein analysis, high-performance liquid chromatography (HPLC) can achieve resolution in mixtures of molecules with similar properties. However, there are disadvantages, such as the potential for loss of sensitive or unstable compounds during the procedure.
Spectroscopy
Spectroscopy involves measuring the interaction of molecules with electromagnetic radiation. This technique provides insights into the structural and functional properties of biomolecules. Its main advantage is the ability to analyze compounds in various environments almost non-destructively.
Infrared spectroscopy and nuclear magnetic resonance (NMR) spectroscopy are popular choices in biochemistry. The unique aspect of spectroscopy is the detailed information it can provide regarding molecular structure and dynamics. However, it sometimes requires specialized equipment and skilled personnel to interpret complex data properly, posing challenges for its widespread application.
Electrophoresis
Electrophoresis is a technique that separates charged particles in a gel or liquid medium through an electric field. One of its primary uses is the analysis of nucleic acids and proteins based on size and charge. Its key characteristic is the straightforward application, where researchers can visualize results directly.
The unique aspect of electrophoresis lies in its ability to provide a quick snapshot of sample integrity and purity. Techniques like SDS-PAGE allow researchers to assess protein purity rapidly. Despite its benefits, electrophoresis may not provide quantitative data as effectively as other methods, limiting its utility in some applications.
Molecular Biology Techniques
Molecular biology techniques have revolutionized research in biochemistry. They include methods for manipulating DNA, RNA, and proteins, facilitating advancements in genetics, medicine, and biotechnology.
Polymerase Chain Reaction (PCR)
Polymerase Chain Reaction (PCR) amplifies specific sequences of DNA, making it invaluable for genetic analysis. The technique's key characteristic is its exponential amplification of target DNA, allowing even trace amounts to be analyzed effectively.
A unique feature of PCR is its flexibility. Researchers can adapt it for various applications like diagnostics, cloning, and forensic analysis. The disadvantage may include contamination risks, which require careful design and handling to prevent erroneous results.
CRISPR Technology
CRISPR technology has emerged as a revolutionary tool for genetic editing. Its primary contribution is the ability to target specific genes for modification with precision. The key characteristic of CRISPR is its simplicity and efficiency compared to earlier techniques, which required complex systems for gene editing.
A unique aspect of CRISPR lies in its potential for broad applications, including agricultural improvement and treatment of genetic diseases. However, ethical implications and off-target effects remain as considerations that warrant further exploration as its use expands.
Gene Cloning
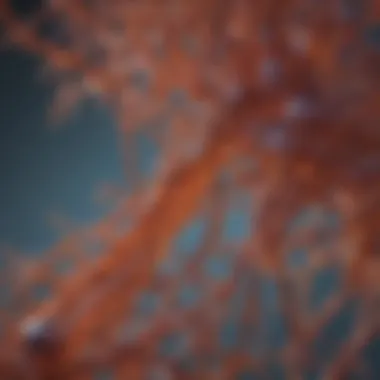
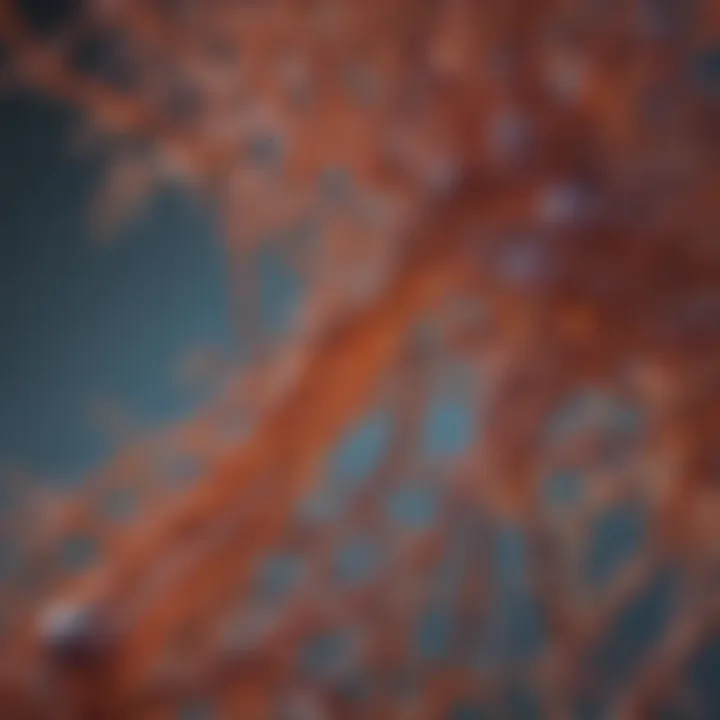
Gene cloning involves creating copies of specific DNA fragments, usually for research or therapeutic purposes. This method's key characteristic is its ability to produce large quantities of a desired gene or protein for study.
A significant advantage of gene cloning is its wide applicability across various fields, including biotechnology and medicine. However, gene cloning can encounter challenges related to vector choice and insertion efficiency, which can impact the success of the process.
Understanding Metabolism
Metabolism is a core concept in biochemistry that involves the entirety of biochemical reactions within living organisms. Understanding metabolism is crucial for students, educators, and professionals because it provides insights into how organisms obtain and utilize energy. This understanding is instrumental in several fields such as medicine, nutrition, and environmental science. Importantly, metabolic processes are not isolated—they are interconnected and intricately regulated, reflecting the complexity of life.
Metabolism can be broadly classified into two categories: catabolism and anabolism. Catabolic reactions break down molecules, releasing energy stored in chemical bonds. These reactions often involve the degradation of macromolecules such as carbohydrates, proteins, and lipids into simpler units. In contrast, anabolic processes use energy to construct complex molecules from simpler ones, playing a fundamental role in growth and maintenance of cellular structures. This duality of metabolism illustrates how organisms balance energy requirements with the need for growth and repair, presenting a dynamic arena for biochemical study.
Furthermore, the study of metabolism informs clinical research, particularly in the understanding of metabolic disorders. Conditions such as diabetes or obesity arise from imbalances in these metabolic pathways. By comprehending the underlying biochemical principles, interventions and treatments can be designed to correct these imbalances, enhancing patient outcomes.
Metabolism is not just about energy production; it is a web of interconnected pathways that sustain life and health.
Catabolism and Anabolism
Catabolism and anabolism are two essential components of metabolism. Catabolism refers to the processes that break down complex molecules, releasing energy that can be used for cellular activities. Enzymatic reactions in catabolism typically occur in stages, where large substrates are progressively broken down. For instance, in cellular respiration, glucose is metabolized through glycolysis, the citric acid cycle, and oxidative phosphorylation to produce ATP.
On the other hand, anabolism encompasses the synthesis of complex molecules from simpler ones, requiring energy input. This allows cells to build proteins, nucleic acids, and lipids, which are vital for cellular functions and overall growth. Often, anabolic reactions are driven by ATP, which is generated during catabolic processes.
The balance between catabolic and anabolic pathways is tightly regulated. Hormonal signals such as insulin and glucagon play critical roles in managing this equilibrium, influencing how energy flows within the body. An imbalanced metabolism can lead to various health issues, emphasizing the importance of studying these processes in both educational and practical settings.
Bioenergetics
Bioenergetics is a sub-discipline of biochemistry that deals with the study of energy flows through living systems. It considers how energy is transferred, conserved, and utilized during metabolic activities. Energy transformations are central to all biological processes, making bioenergetics a foundational aspect of understanding metabolism.
The concept of Gibbs free energy is significant in bioenergetics, as it determines the spontaneity of reactions. Understanding free energy changes helps in predicting reaction behaviors during metabolism, allowing researchers to assess which reactions can occur under given conditions. Moreover, bioenergetics investigates the efficiency of energy conversions in cellular processes and the impact of environmental factors on these dynamics.
The integration of bioenergetics with other biochemistry fundamentals equips students and educators to approach complex biological systems. It opens avenues for researching energy-related diseases, improving energetics in sports medicine, and optimizing cellular processes in biotechnology. These aspects further underline the relevance of bioenergetics in modern scientific inquiry and practical applications.
Biochemistry and Human Health
Biochemistry has a vital connection to human health. It shapes our understanding of how biological molecules interact to maintain life and function. This section emphasizes how biochemical principles influence health, disease, and the body’s response to various stimuli.
Nutritional Biochemistry
Nutritional biochemistry focuses on how food is metabolized and utilized by the body. Understanding the molecular basis of nutrition is essential for addressing health issues related to diet, metabolism, and overall well-being.
Key elements in this area include:
- Micronutrients and Macromolecules: Each nutrient, such as proteins, carbohydrates, and fats, plays a crucial role in bodily functions. For instance, proteins serve as building blocks for tissues, while carbohydrates are a primary energy source.
- Metabolic Pathways: The biochemical pathways that convert food into energy and biomolecules reflect individual nutritional needs. This knowledge can guide dietary choices and interventions.
- Health Implications: Deficiencies or excesses in nutrients can lead to serious health problems, such as obesity, diabetes, and heart disease. Nutritional biochemistry informs public health policies aimed at improving diet quality.
Biomarkers and Disease
Biomarkers offer valuable insights into disease states and can aid in diagnosis, prognosis, and treatment decisions. Their study involves analyzing biochemical changes that occur in response to disease.
Cancer Biochemistry
Cancer biochemistry examines the biochemical alterations that accompany cancer progression. It highlights the significance of abnormal metabolic pathways and the role of oncogenes and tumor suppressor genes. The key characteristics of cancer biochemistry include its focus on:
- Cell Signaling Pathways: Cancer cells often exhibit altered signaling pathways that can promote uncontrolled growth. Understanding these changes is crucial for developing targeted therapies.
- Metabolic Dysregulation: Many cancers have unique metabolic profiles. By studying these traits, researchers can identify potential therapeutic targets.
- Research Relevance: Cancer biochemistry is a prominent area of study due to the pressing need for effective treatments. It aids in the formulation of personalized medicine strategies that tailor therapy based on a patient's specific cancer biochemistry.
Cardiovascular Health
Cardiovascular health is deeply intertwined with biochemical processes, such as lipid metabolism and blood coagulation. Understanding these aspects can significantly contribute to heart disease prevention and management.
- Cholesterol Metabolism: High levels of low-density lipoprotein (LDL) cholesterol are a major risk factor for heart disease. Biochemical studies focus on how dietary fats and other factors influence cholesterol levels.
- Inflammation: Chronic inflammation plays a key role in cardiovascular diseases. Biochemical markers of inflammation can help in assessing risk and response to treatment.
- Preventive Strategies: Knowledge from cardiovascular biochemistry aids in public health strategies aimed at reducing heart disease incidence, such as dietary recommendations and lifestyle changes.
Metabolic Disorders
Metabolic disorders, such as diabetes or obesity, arise from disruptions in normal biochemical processes. This area is critical for developing effective treatment and management strategies.
- Insulin Resistance: Understanding the biochemistry of insulin signaling is vital for addressing type 2 diabetes. Interventions targeting this pathway can help restore normal glucose metabolism.
- Genetic Factors: Many metabolic disorders have a genetic basis. Biochemical research can uncover these links, leading to better diagnostic tools and therapies.
- Societal Impact: The rise in metabolic disorders globally poses a significant public health challenge. Addressing these issues through biochemistry not only improves individual health but also alleviates economic burdens.
"The understanding of biochemical processes is essential for advancing human health outcomes and addressing global health challenges."
This exploration of biochemistry and human health reveals the profound implications of biochemical research in enhancing health, preventing disease, and understanding the complexities of metabolic pathways. Each subfield, from nutritional biochemistry to the study of metabolic disorders, provides a crucial framework for addressing contemporary health issues.
Applications of Biochemistry
Biochemistry plays a pivotal role in modern science and medicine. The application of biochemical principles stretches across various fields, offering insights that enhance both understanding and improvement of health and technology. Recognizing the importance of biochemistry helps in bridging gaps in knowledge and addressing real-world challenges.
One significant area of application is biotechnology and genetic engineering. These fields combine biochemistry with advanced technology to manipulate biological systems at the molecular level. Such innovations lead to advancements in agriculture, healthcare, and environmental management. For instance, genetically modified organisms (GMOs) often showcase improved nutritional profiles and enhanced resistance to pests, showing direct impacts on food security.
In addition, pharmaceutical applications utilize biochemistry for drug development. Biochemistry allows scientists to understand disease mechanisms at a molecular level, which is crucial for designing effective therapies. The integration of biochemical techniques in drug discovery processes not only accelerates the development timeline but also optimizes drug efficacy and safety.
"The influence of biochemistry on real-world applications cannot be overstated. It serves as a foundation that supports various scientific disciplines and practical implementations.”
Moreover, the benefits of these applications are vast:
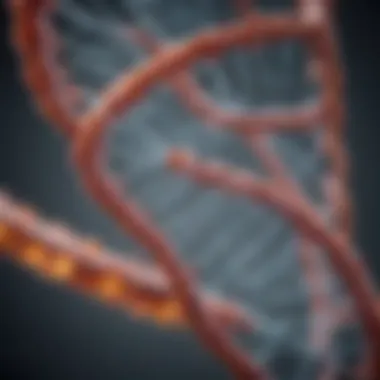
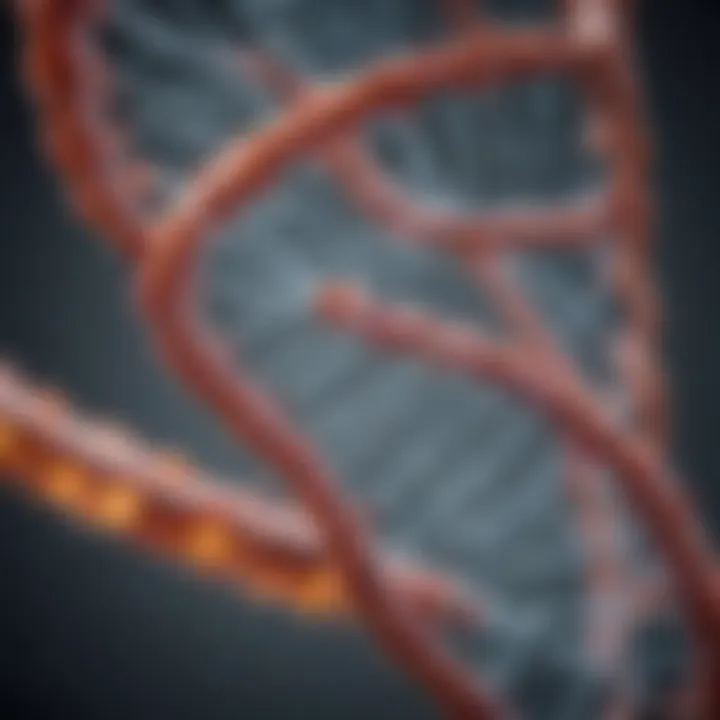
- Increased efficiency in drug production and vaccine development.
- Improved diagnostic tools that leverage biochemical markers for early disease detection.
- Development of sustainable agricultural practices through biotechnological advances.
As we explore specific applications in biotechnology and pharmaceuticals, it should be noted that these fields maintain high ethical considerations. Discussions around the moral implications of genetic modifications and the safety of new drugs are vital in steering responsible advancements in biochemistry.
Biotechnology and Genetic Engineering
Biotechnology harnesses the principles of biochemistry to develop technologies and products that improve lives and the health of our planet. This field encompasses everything from agricultural innovations to medical breakthroughs. Genetic engineering, as a subset of biotechnology, focuses specifically on altering the genetic material of organisms.
Utilizing techniques like CRISPR and recombinant DNA technology, scientists can edit genes with precision. This allows for targeted outcomes, such as disease resistance in crops or the production of insulin in bacteria. These advancements serve not just the pharmaceutical industry, but also address broader global challenges like food scarcity and climate change.
Key benefits of biotechnology and genetic engineering include:
- Enhanced crop yields: Organisms can be engineered for better growth traits.
- Disease prevention: Genetically modified plants can resist pests, reducing chemical pesticide use.
- Medical advancements: Personalized medicine is becoming more feasible through genetic insights.
However, these advancements are not without concerns. Societal acceptance, regulatory frameworks, and ethical considerations around living modified organisms spark ongoing discussions. Accountability and adherence to best practices must remain a requirement as the field progresses.
Pharmaceutical Applications
Pharmaceutical applications of biochemistry thrive on understanding life processes at the molecular level. Drug discovery, development, and production are deeply rooted in biochemical knowledge. A pivotal aim is to create more targeted therapies with fewer side effects.
A vital aspect includes the development of biopharmaceuticals, which are drugs produced using biotechnology. These include therapies derived from proteins, nucleic acids, and living cells.
Among the most significant areas of pharmaceutical applications are:
- Monoclonal antibodies: Used in treating various diseases, including cancers and autoimmune disorders.
- Vaccines: Advances in biochemistry have led to safer and more effective vaccines.
- Gene therapy: This innovative approach addresses genetic conditions by correcting defective genes.
The impact of these applications significantly alters patient treatment paradigms. Drug screenings now utilize biochemical assays for preliminary evaluations, vastly improving the efficiency of the drug discovery process. As biochemistry continues to evolve, leveraging new technologies, the potential for innovative drugs becomes greater.
In summary, the applications of biochemistry extend well beyond the laboratory. They hold the potential to solve critical challenges in health, agriculture, and environmental sustainability.
Ethical Considerations in Biochemistry
Ethical considerations in biochemistry are crucial as the field impacts many aspects of society, from healthcare to environmental practices. A clear understanding of ethics in biochemistry helps guide research efforts, ensuring integrity and respect for life. As researchers innovate, ethical dilemmas often arise regarding the use and manipulation of biological materials, which necessitates a robust framework of ethical standards.
Research in biochemistry frequently involves human subjects or animal testing. This aspect of biochemistry demands strict adherence to ethical guidelines to protect participants from harm and to ensure that their rights and well-being are prioritized. It also necessitates transparency regarding the methods and intentions behind the research. Such principles draw on previous regulations, like the Belmont Report, which outlines respect, beneficence, and justice as core tenets.
The ethical landscape also shifts with advancements in technology. For instance, the development of CRISPR technology allows for precise gene editing, yet it raises concerns about potential misuse or unintended consequences on living organisms. Thus, as we ponder biochemical innovations, the necessity of ethical reflection becomes even more pronounced.
"The integrity of biochemistry hinges not just on scientific facts, but on the ethical framework that supports them."
Furthermore, engaging with social and ethical implications is essential when considering the applications of biochemistry in society. Innovations, such as genetically modified organisms (GMOs), spark debate among consumers and scientists alike. Awareness and communication of the benefits and risks can help foster public trust.
In summary, the importance of ethical considerations in biochemistry ensures that research is conducted responsibly. It promotes trust in scientific endeavors, encouraging collaboration between academia, industry, and the public. As researchers navigate complex moral landscapes, they must remain vigilant, maintaining focus on the broader implications of their work.
Ethics in Research
Ethics in research forms the backbone of trustworthy biochemistry. When scientists engage in research, they must consider the impact of their work at every stage. From the initial design to the final publication, ethical implications are paramount. These principles guide researchers in obtaining informed consent from participants, adhering to regulations, and maintaining transparency throughout the process.
The potential benefits of ethical research extend beyond compliance; they enhance credibility and foster collaboration. Therefore, researchers must create an atmosphere of respect and responsibility, ensuring that the results can be reliably used to inform medical and scientific communities.
Some key aspects of ethics in research include:
- Informed Consent: Researchers must ensure that participants fully understand the study's purpose and risks before participating.
- Data Integrity: It is essential to maintain accuracy and honesty in reporting research findings.
- Minimizing Harm: All efforts should be made to prevent physical or psychological distress to participants involved in the research.
- Accountability: Researchers must hold themselves responsible for the ethical implications of their work.
Biochemical Innovations and Society
The relationship between biochemical innovations and society is intricate and often contentious. As breakthroughs such as biopharmaceuticals or synthetic biology emerge, they promise substantial benefits but also invite ethical scrutiny. Innovations can lead to improved treatment options for diseases or enhanced food production, impacting millions.
However, with great power comes great responsibility. Society must grapple with the implications of such advancements. Concerns about genetic privacy, food security, and the potential for inequality in access to treatments are all critical considerations. Therefore, public discussions and regulations must evolve concurrently with scientific progress.
Some of the most pressing topics include:
- Access to Treatments: Are new medications developed from biochemistry accessible to all sectors of society?
- Environmental Impact: Do innovations in biochemistry promote sustainable practices, or do they potentially harm ecosystems?
- Public Perception: How does public understanding of biochemistry influence acceptance of innovations?
The End
The conclusion section serves as a vital component of this article by synthesizing the key insights gained from exploring both foundational concepts and advancements in biochemistry. It is important as it encapsulates the essence of the discussions that have transpired throughout the previous sections. By summarizing these points, the conclusion allows for a clearer understanding of how biochemistry is interwoven into the fabric of modern sciences.
One of the primary benefits of reflecting on the principles of biochemistry lies in acknowledging its applications. From the study of metabolic pathways to the development of novel therapies, biochemistry holds significance across varied domains including healthcare and environmental science. The advancements in biochemical techniques enhance our capability to manipulate biological systems to our advantage, addressing both current and future challenges.
Additionally, ethical considerations in research cannot be overlooked. As biochemistry continues to evolve, maintaining an ethical framework to guide experimentation and application becomes paramount. These ethical aspects contribute to the credibility and reliability of scientific endeavors in biochemistry.
Through this concluding section, we highlight that the exploration of biochemistry is not just an academic pursuit; it is a critical discourse that impacts real-world issues. The continuous evolution of this field, juxtaposed with ethical considerations, solidifies its relevance. Future scholarly works in this domain must build on these foundational principles, ensuring that the knowledge gained is applied responsibly and effectively.
Future Directions in Biochemistry
Looking ahead, the future directions in biochemistry encompass several emerging areas poised to redefine our understanding of biological processes. The integration of multidisciplinary approaches is essential as researchers seek to unravel the complexities of life at the molecular level. Key areas to watch include:
- Synthetic Biology: Engineering biological systems to create novel functions offers immense potential in drug development and environmental remediation.
- Personalized Medicine: Advancements in genomics and proteomics are paving the way for treatments tailored to individual genetic profiles, thus improving efficacy and minimizing adverse effects.
- Biochemical Data Science: Leveraging bioinformatics and machine learning to analyse complex biochemical datasets yields new insights and enhances predictive capabilities in drug discovery.
Research efforts must emphasize collaboration across various scientific fields. Interdisciplinary teams can tackle the complex challenges that arise in biochemistry, ultimately bringing about innovative solutions.
In summary, future directives in biochemistry should embrace the inherent complexity of living systems while fostering ethical practices. These directions will not only guide research but also ensure the relevance of biochemistry in addressing societal needs.
The future of biochemistry holds the promise of significant breakthroughs that can fundamentally alter our understanding of life and health.