Exploring the World of Biosensors and Their Applications
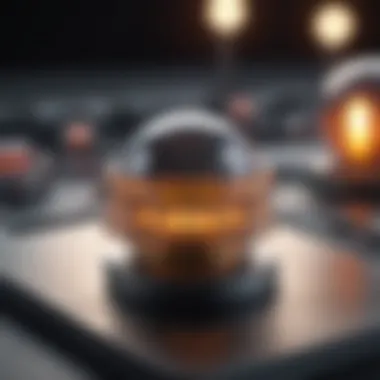
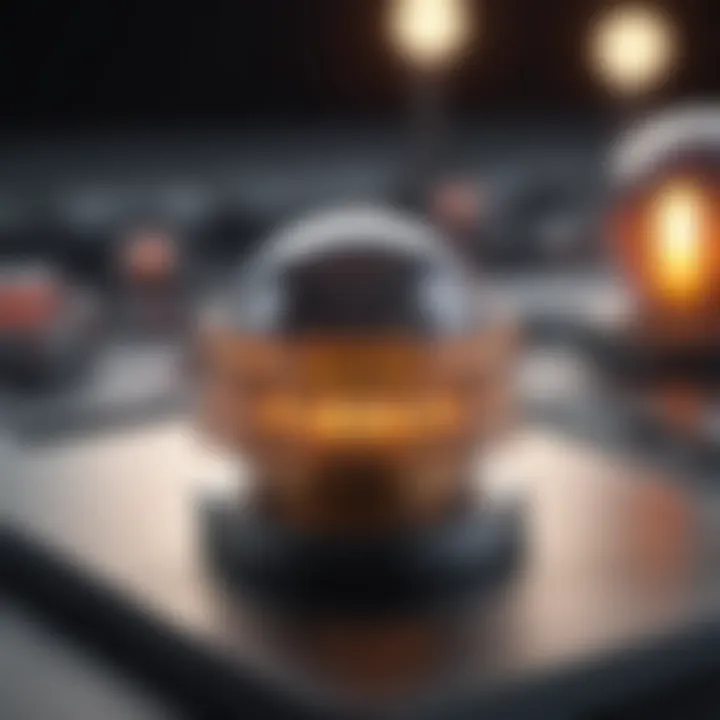
Intro
Biosensors represent a unique blend of biology and technology, offering innovative solutions in various fields, from medical diagnostics to environmental monitoring. They act as pivotal tools in decoding biological responses into measurable signals, which can be quantified and analyzed. With roots tracing back several decades, the evolution of these devices highlights their growing significance in the scientific community.
In the core of their operating principles lies the synergy between a biological component—often an enzyme or a receptor—and a physical transducer that converts the interaction into a readable signal. This process underpins the remarkable efficiency and specificity of biosensors when detecting specific analytes, such as glucose levels in diabetic patients or toxins in water sources.
With advances in nanotechnology and materials science, the capabilities of biosensors have expanded significantly. This exploration provides a window into the mechanisms, applications, and future trajectories of biosensing technology. Understanding these elements is crucial for researchers and professionals looking to harness the power of biosensors in their respective domains.
From academia to industrious settings, the implications of biosensor technology are profound. They not only enhance diagnostic precision but also pave the way for real-time monitoring of health and environmental conditions—essential in our ever-evolving world. As we embark on this comprehensive exploration, it’s vital to grasp the foundational aspects that make biosensors indispensable tools in today’s scientific toolkit.
Foreword to Biosensors
In today’s rapidly evolving landscape of science and technology, the significance of biosensors cannot be overstated. They serve as a powerful bridge between biological systems and analytical devices, providing crucial insights across various applications ranging from healthcare to environmental monitoring. By dissecting the components and functioning of these devices, this article aims to unpack their importance and amplify awareness among students, researchers, educators, and professionals alike.
Definition and Significance
Biosensors are analytical devices that utilize biological materials, such as enzymes, antibodies, or nucleic acids, to detect and measure a wide variety of substances – from glucose in diabetic patients to toxins in contaminated water. They convert biological responses into quantitative signals, often using a transducer that facilitates this transformation.
The significance of biosensors lies in their ability to provide real-time data with high sensitivity and specificity. For instance, in medical diagnostics, a biosensor can identify biomarkers associated with diseases, thus enabling timely interventions. Moreover, their portability and ease of use allow them to be employed outside traditional laboratory settings, widening their reach and applications.
Historical Overview
The journey of biosensors begins in the late 1960s when the concept of utilizing biological recognition elements for analytical measurement was first proposed. The use of enzyme electrodes marked a pivotal moment, allowing for the direct measurement of biochemical substances through electrochemical principles.
Since then, there have been staggering advancements:
- In the 1980s, biosensors entered the realm of medical diagnostics; for example, the development of the first glucose biosensor revolutionized diabetes management.
- The 1990s saw enhancements in specificity with the advent of immunosensors, facilitating the detection of specific proteins in clinical samples.
- Fast forward to today, and we see cutting-edge technologies that incorporate nanotechnology and electronic systems, significantly enhancing the performance of biosensors.
The historical growth of biosensors is a testament to their potential. As we continue to march into the future, they stand poised to tackle some of the toughest challenges in healthcare, environment, and food safety, making their study all the more pressing and essential.
Principles Behind Biosensors
Understanding the principles behind biosensors is quintessential for grasping how they operate and lend themselves to a variety of applications across the scientific landscape. These principles form the bedrock of biosensor technology, highlighting not only the intricate dance between biology and technology but also the immense potential these devices hold for revolutionizing fields like medicine and environmental science.
Key elements—bioreceptors and transducers—offer insight into how biosensors detect and analyze biochemical processes. Bioreceptors specifically bind to target molecules, enabling the transducer to convert this binding event into an interpretable signal. By comprehending these components, we can appreciate the nuanced benefits of biosensing technologies, including their sensitivity, speed, and overall efficiency.
Bioreceptors and Their Role
Bioreceptors are specialized biological molecules that interact with specific analytes or targets. They play an integral role in the functionality of biosensors. Typically composed of proteins, enzymes, or nucleic acids, these receptors provide the selectivity needed for accurate detection. For example, an enzyme-based biosensor may utilize glucose oxidase as a bioreceptor, effectively sensing glucose levels in blood samples.
Highlighting the significance of bioreceptors, they not only identify the presence of target chemicals but do so with remarkable precision. This selectivity allows for the monitoring of various biological conditions and chemical substances, making biosensors invaluable tools in diagnostics and environmental monitoring.
Furthermore, bioreceptors’ performance hinges on factors like stability and binding affinity. A stable bioreceptor can reliably function over time, while a high binding affinity ensures that a minuscule amount of target material can still evoke a detectable response, thus elevating the sensor’s sensitivity.
Transducer Mechanisms
Transducers act as the critical link between the biological detection and the electronic readout. They convert the biochemical signal generated by the bioreceptor into an electrical signal, which can then be amplified and processed for analysis. Different types of transducers are acknowledged, each with unique characteristics that can suit various applications.
Electrochemical Transducers
Electrochemical transducers hinge on electrochemical reactions to provide quantitative measurements. They are particularly noted for their high sensitivity, making them a go-to option for biosensing applications such as blood glucose monitoring. One of the prominent features of electrochemical transducers is their ability to operate in complex biological environments, like blood serum, without losing accuracy. This factor renders them incredibly beneficial for medical diagnostics.
However, there are drawbacks. These transducers require careful calibration and can be sensitive to fluctuations in temperature and pH. Such constraints can affect performance, necessitating robust protocol and environmental controls to maintain reliability in results.
Optical Transducers
Optical transducers utilize light properties—absorption, fluorescence, or reflectance—to generate a measurable signal in response to analyte concentration. A key characteristic of optical transducers is their non-destructive nature, which allows for real-time monitoring of biochemical processes without altering sample integrity. Though popular for applications like food safety testing, they can be sensitive to environmental conditions like light exposure, which can skew results.
The unique feature of these instruments is their ability to analyze samples with minimal disruption, enabling continuous, in-situ measurements. Yet, the costs associated with high-quality optical components can be a limiting factor for some users.
Mass-Based Transducers
Mass-based transducers, such as quartz crystal microbalance, measure the mass change of a sensor surface caused by the binding of target molecules. An essential aspect of mass-based transducers is their extreme sensitivity to mass changes, making them advantageous for detecting low concentrations of analytes.
However, they often require stringent calibration and are generally less adaptable to variable environments than other transducer types. Such limitations can prevent their widespread adoption in certain situations.
In summary, each transducer type has features that render them either advantageous or less optimal for specific applications in biosensing. Understanding these mechanisms is fundamental to advancing biosensor technologies, emphasizing the delicate interplay between the bioreceptors and transducer characteristics that define their utility in real-world scenarios.
Components of a Biosensor
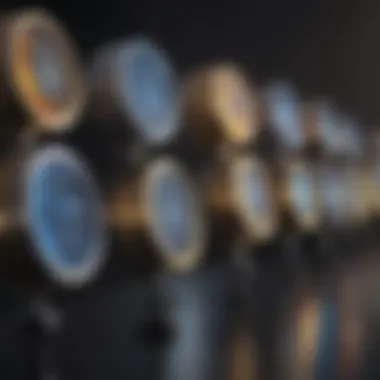
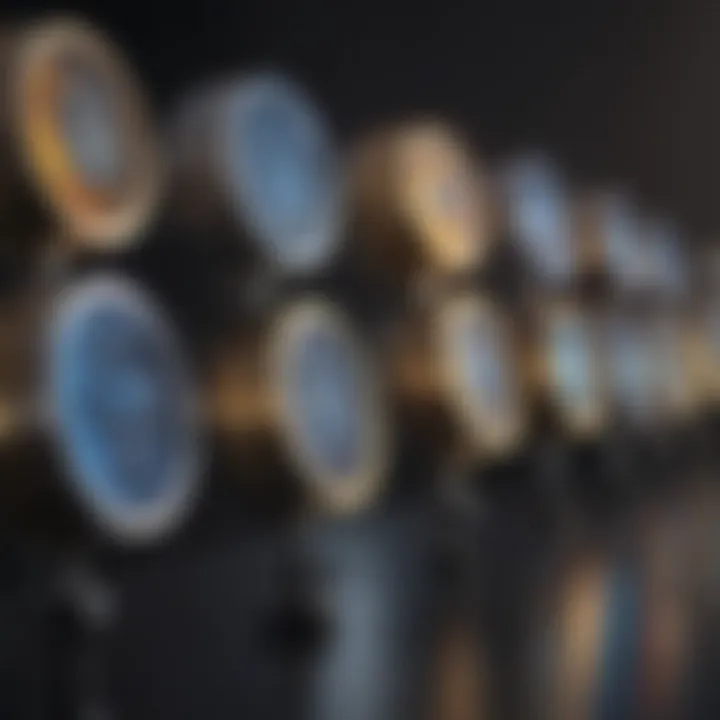
In the intricate arena of biosensors, components play a pivotal role. Each element contributes to ensuring that these devices can accurately detect, quantify, and analyze biological materials. Understanding these components gives insight into not just the functionality but also the potential applications and limitations of biosensor technology.
The Bioreceptor Material
The bioreceptor is arguably the heart of any biosensor. It serves as the sensing element that interacts specifically with the target analyte. Different types of bioreceptors, including enzymes, antibodies, nucleic acids, and whole cells, exhibit unique properties that determine their effectiveness for various applications. For instance, enzyme-based biosensors utilize the catalytic activity of enzymes for highly selective detection of substrates.
Key factors influencing bioreceptor selection include:
- Specificity: The ability to selectively bind to the target without interference from other substances.
- Stability: The longevity of the bioreceptor’s activity is crucial, particularly in real-world applications.
- Cost-Effectiveness: Some bioreceptor materials can be expensive to produce or maintain, dictating their commercial viability.
The selection of the bioreceptor material can heavily influence the sensitivity and performance of the entire biosensing system. A well-chosen bioreceptor enhances detection limits, making the biosensor suitable for environments where precise measurements are critical.
The Transducer Element
The transducer element acts as the bridge between the biological reactions occurring at the bioreceptor and the electrical signal that represents these actions. This component converts the biological response into a measurable output, allowing for data interpretation. Multiple types of transducers exist, each suited for different environments and applications.
Some common transducer mechanisms include:
- Electrochemical Transducers: These devices operate based on the current or potential changes induced by biochemical reactions. They are widely used in applications like glucose monitoring, making them a staple in diabetes management.
- Optical Transducers: Utilizing light, these transducers measure absorbance, fluorescence, or reflectance changes. They are often employed in biosensors catering to applications in biomedical research and environmental analysis.
- Mass-Based Transducers: This type measures changes in mass at the surface of a sensor, usually implemented in piezoelectric technologies. These are crucial in detecting minute changes and can be impactful in environmental biosensing.
Choosing the appropriate transducer is vital because it shapes how effectively the biosensor can convert biological signals into quantifiable data.
Amplifiers and Signal Processors
After the transducer converts the biological signal into a measurable format, amplifiers and signal processors are used to enhance and interpret this data. This stage is crucial, as weak signals can easily be masked by noise.
- Amplifiers boost the output signal, ensuring that even minor biological events can be detected. Without adequate amplification, many useful signals might simply go unnoticed.
- Signal Processors perform essential calculations and filtering on the amplified signal, shaping the data into a user-friendly format. This step often involves software algorithms that can differentiate between noise and meaningful signals, thus providing clarity in analysis.
By improving sensitivity and specificity during this stage, biosensors can deliver accurate readings that essential in medical diagnostics, industrial monitoring, and environmental assessments.
Highlighting the interplay among components is vital. A biosensor's efficacy hinges not only on singular elements but on how these components work together to create a seamless, dependable device.
Types of Biosensors
Understanding the types of biosensors is crucial in grasping how these devices harness biological components to detect specific substances. Each type of biosensor has its own operational principles and applications, which leads to a diverse array of uses in fields such as medicine, environmental monitoring, food safety, and more. Their significance lies not only in their ability to detect and quantify specific analytes but also in their optimized functionality that caters to different monitoring needs. The following sections delve into four major categories of biosensors: enzyme-based, immunosensors, DNA biosensors, and cell-based biosensors.
Enzyme-Based Biosensors
Enzyme-based biosensors are among the earliest and widely utilized biosensors. Their mechanism primarily revolves around the catalytic properties of enzymes, which respond to specific substrates. When an enzyme interacts with its target, it produces a measurable signal, often an electrical signal that correlates with the concentration of the analyte.
A major advantage is their high specificity. For instance, glucose oxidase is commonly used in glucose biosensors, notably in diabetes management. With these biosensors, individuals can monitor their glucose levels quickly and easily, aiding in effective blood sugar management.
Factors such as temperature and pH can influence enzyme activity, which brings attention to the need for careful calibration and environmental control in practical applications. This consideration affirms the necessity for ongoing development and refinement in enzyme stability to maintain their efficacy over time.
Immunosensors
Immunosensors leverage the specificity of antibodies to detect antigens, allowing them to recognize pathogens or biomarkers with high precision. The binding of an antibody to its specific antigen produces a signal that can be measured and quantified. This characteristic makes immunosensors invaluable in medical diagnostics, particularly for detecting diseases at early stages.
For example, immunosensors are widely employed in the detection of infectious diseases, such as HIV, where they measure the presence of antibodies in a patient’s sample. They’re not only effective in diagnosis but also in monitoring therapeutic effectiveness and viral load.
Despite their many advantages, immunosensors can face challenges related to cross-reactivity or interference from other compounds in biological samples. Accurate results hinge on refining the antibody selection and ensuring the purity of reagents used in the process.
DNA Biosensors
As we move into the realm of molecular biology, DNA biosensors have carved out a pivotal role. Utilizing nucleic acid hybridization principles, these biosensors can detect specific sequences of DNA. When target DNA molecules bind to a probe, they generate a measurable change in signal, which can be quantified.
The precision in identifying genetic sequences makes DNA biosensors critical in areas like genetic testing, disease diagnosis, and even forensic science. For instance, researchers are using these biosensors to diagnose genetic disorders by detecting specific mutations in a patient’s DNA. Their sensitivity allows for the detection of single nucleotide variations, which can indicate a genetic predisposition to certain diseases.
However, they do come with their own set of limitations, such as the potential for false positives and the necessity for highly controlled conditions to accurately assess results. Ensuring specificity and minimizing background noise remain areas of active research.
Cell-Based Biosensors
Cell-based biosensors harness living cells as bioreceptors, providing a dynamic and context-sensitive approach to biosensing. By utilizing cells that respond to specific stimuli, these biosensors are capable of detecting a wide range of substances, from nutrients to toxic compounds. The unique advantage here is their ability to respond to the complex interplay of biological systems, generating real-time insights into cellular activities.
These biosensors find significant application in drug testing environments, where the toxicity of new compounds can be assessed through cellular responses. Additionally, they play a vital role in environmental monitoring, helping scientists gauge the health of ecosystems by monitoring how living organisms react to pollutants.
The reliability of cell-based biosensors can be influenced by various factors like cell viability and culture conditions. Innovating ways to enhance their stability while accurately reflecting physiological responses could pave the way for broader applications in the future.
"Biosensors turn biological interactions into measurable quantities, making them essential for research and diagnosis across multiple fields."
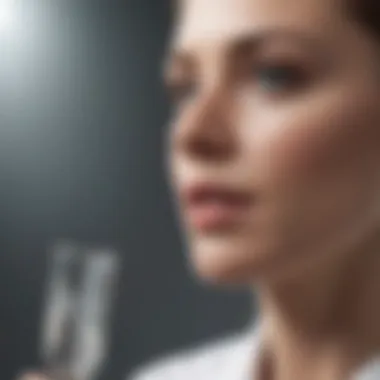
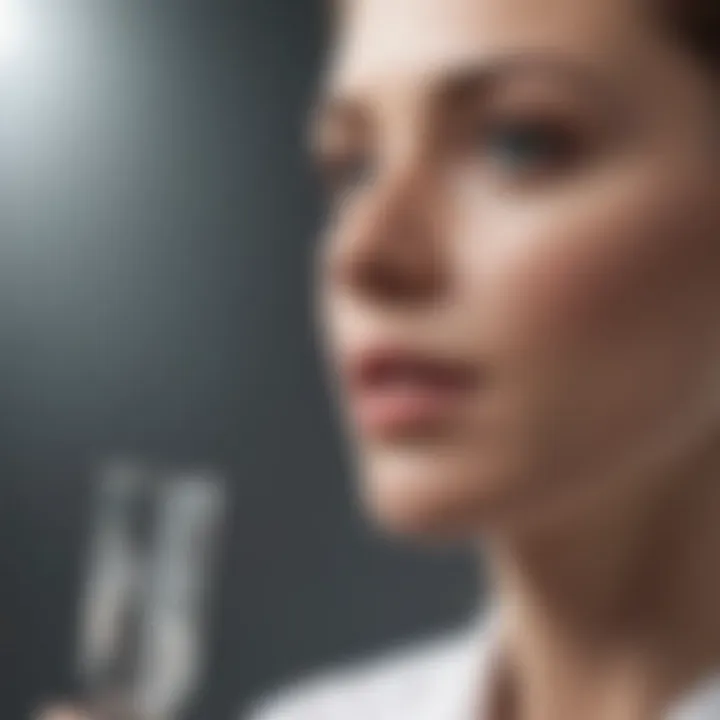
In summarizing the discussion on types of biosensors, each one shows how biological principles transform into practical tools that can assist scientists, medical professionals, and environmentalists. Their diversity in approach and function resonates through various applications, underlining their importance in modern scientific inquiry.
Applications of Biosensors
Biosensors are at the forefront of several industries, playing crucial roles in a multitude of applications that range from healthcare to environmental monitoring. Their ability to provide real-time data and precise measurements makes them indispensable tools in various fields. In this section, we will explore four key applications of biosensors, namely medical diagnostics, environmental monitoring, food safety testing, and bioprocess control. This exploration will highlight specific benefits and considerations relevant to each area, offering a comprehensive insight into the profound impact of biosensors in everyday life.
Medical Diagnostics
In the realm of medical diagnostics, biosensors have become essential for rapid and accurate detection of diseases. They can detect specific biomarkers present in bodily fluids, such as blood or saliva, that indicate the presence of a disease. For instance, glucose biosensors are widely used by patients with diabetes to monitor their blood sugar levels.
Advantages include:
- High accuracy: Biosensors often show exceptional specificity and sensitivity, making them reliable for clinical use.
- Speed: Many biosensors deliver results in a matter of minutes, which is crucial in emergency situations.
- Minimally invasive: Many devices require only small samples, making them patient-friendly.
However, there are challenges too. The design stability and reproducibility must be maintained to avoid false results. While many biosensors currently focus on prevalent diseases, there's room to innovate for more rare conditions as well.
Environmental Monitoring
Biosensors are equally crucial in environmental monitoring, offering valuable insights into pollution levels and ecological health. They can identify contaminants in water, soil, and air with unparalleled precision. For example, biosensors that detect heavy metals or pesticides in agricultural runoff provide essential data for sustainable practices.
Key Benefits:
- Real-time data: They allow for continuous monitoring, which helps in swift regulatory compliance.
- Cost-effective: Compared to traditional methods, these technologies can reduce operational costs significantly.
- Simplicity in use: Many biosensors are designed for ease of use, requiring less specialized training for monitoring teams.
Yet, challenges persist, such as the need for extensive calibration and the potential for interference from simultaneous environmental variables. Improvements in sensor technology are likely to address these limitations.
Food Safety Testing
In food safety, biosensors play a pivotal role in ensuring that contaminants are detected before consumption. From pathogens to chemical residues, the breadth of what biosensors can detect is vital for industry compliance. For instance, using biosensors for Salmonella detection can drastically reduce the risk of foodborne illnesses.
Considerations include:
- Rapid testing: Swift results support faster decision-making in food manufacturing and distribution.
- Versatility: They can be tailored to detect various contaminants, making biosensors adaptable to changing food safety standards.
- Consumer confidence: Utilizing reliable biosensors in food production can enhance public trust.
On the flip side, the challenge lies in the integration of biosensors within the existing food safety frameworks. Regulatory acceptance of new technologies often requires significant time and resources.
Bioprocess Control
Biosensors also find their importance in bioprocess control within biomanufacturing and pharmaceutical applications. Here, they monitor critical parameters such as pH, temperature, and metabolite levels to optimize the production process of bio-products.
Benefits of bioprocess biosensors include:
- Enhanced yield: Real-Time monitoring allows for adjustments that can significantly boost productivity.
- Lower operational costs: Automation reduces the need for manual oversight, leading to cost efficiency.
- Quality assurance: Persistent monitoring ensures the consistency and reliability of product quality.
Nevertheless, integrating biosensors into existing bioprocess systems can pose challenges, particularly in regards to sensor drift and calibration over time. Overcoming these obstacles is crucial for the widespread adoption of biosensing technologies in this domain.
In summary, biosensors are transforming various industries by improving diagnostic accuracy, monitoring environmental changes, ensuring food safety, and enhancing bioprocess controls. Their growing versatility and real-time capabilities open the door for further innovations.
The ongoing advancements in biosensors suggest their integration into more applications will broaden, paving the way for advancements not yet imagined.
Advantages of Utilizing Biosensors
Biosensors have become crucial instruments in various fields due to their ability to offer rapid detection of specific substances. Their unique advantages make them a popular choice for researchers and practitioners. Understanding these merits is not merely an academic exercise; it underscores their significance in practical applications, ranging from healthcare to environmental monitoring. Here, we break down the core benefits that biosensors bring to the table.
High Sensitivity
One of the standout features of biosensors is their high sensitivity, which allows them to detect minute concentrations of analytes. This capability is vital in medical diagnostics and environmental sciences, where early detection can be the difference between life and death. For instance, in glucose monitoring, even a slight change in blood sugar can signal serious health issues. Furthermore, high sensitivity ensures that biosensors can perform effectively in complex sample matrices that might interfere with other analytical methods.
The technology employs bioreceptors, designed to bind specifically with the target substances, thus amplifying the signal even from trace amounts. This specificity means that biosensors often outshine traditional methods in detecting low-level contaminants, making them invaluable for ensuring food safety and monitoring pollutants in water sources.
High sensitivity in biosensors not only enhances detection capabilities but also minimizes the risk of false negatives, crucial for timely interventions in health and environmental sectors.
Rapid Response Time
Another compelling advantage is the rapid response time of biosensors. In high-stakes situations, timely information is paramount. For instance, a biosensor that indicates the presence of pathogens in drinking water can do so in real-time, allowing for immediate action to prevent waterborne diseases. The speed at which biosensors operate comes from their design, which often integrates direct transduction mechanisms that lead to rapid signal generation.
This quick feedback loop is particularly beneficial in dynamic environments, such as hospitals, where quick diagnostics can dictate treatment plans. Similarly, in food safety testing, a fast response can prevent affected products from reaching consumers, thereby safeguarding public health. Such efficiency is unattainable with conventional practices, which may require extensive sample preparation and analysis time.
Cost-Effectiveness
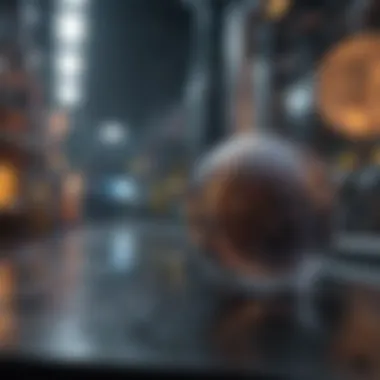
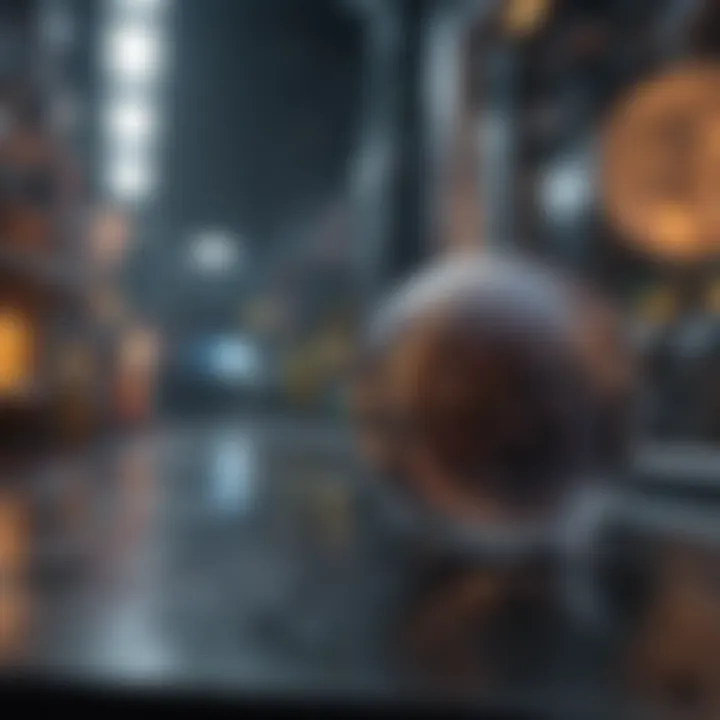
Finally, biosensors present a cost-effective solution for many analytical challenges. While the initial investment might seem daunting, the long-term savings are noteworthy. Biosensors generally require fewer reagents and less time compared to traditional testing methods, leading to reduced operational costs.
Moreover, the ability to perform on-site testing diminishes the need for elaborate laboratory setups, lowering overhead costs. For example, point-of-care biosensors in medical settings cut down not only on time but also on labor expenses associated with sample transport and lab analyses. Government agencies and companies are increasingly recognizing that investing in biosensor technology can lead to more economical and efficient operations.
In summary, the notable advantages of utilizing biosensors lie in their high sensitivity, rapid response time, and cost-effectiveness. These benefits underscore the growing relevance of biosensors in a variety of fields, paving the way for more innovative applications in the future.
Challenges in Biosensor Development
The journey of developing effective biosensors is riddled with hurdles that can stall progress in this innovative field. Despite the immense potential of biosensors in areas like medical diagnostics and environmental monitoring, several technical and regulatory challenges need to be addressed. These challenges are critical not only for the biosensor's adoption but also for its long-term reliability and functionality.
Stability and Sensitivity Issues
Stability and sensitivity are two sides of the same coin when it comes to biosensor performance. Stability refers to how well a biosensor can maintain its performance over time under various conditions. If we think about it, it’s like a watch that loses time; if it cannot keep accurate time, it becomes useless. For biosensors, fluctuations in temperature, humidity, and exposure to light can degrade their functionality.
This is particularly concerning for portable biosensors used in medical settings, where they must produce accurate readings consistently. The sensitivity aspect is equally important. A biosensor must be highly sensitive to detect minute concentrations of target analytes. Failure to do so can lead to false negatives, which can have serious consequences, especially in clinical applications.
To tackle these issues, researchers are focusing on developing more stable bioreceptor materials and enhancing the design of transducers. This could involve using advanced nanomaterials that offer increased stability and higher surface area, effectively boosting sensitivity.
Interference from Environmental Factors
Environmental interference represents another significant obstacle in the biosensing arena. Biosensors are often deployed in varying environments that may contain substances capable of interfering with their readings, much like how background noise can disrupt a delicate performance. Factors such as prevalent chemicals, pH variations, or even temperature swings can lead to unreliable data.
It’s crucial to account for these variables during the development phase. For example, immunosensors used for detecting pathogens must differentiate between a target pathogen and similar biological materials that might trigger a response. Techniques like signal processing and filtering may be vital for minimizing such interference, but they add complexity to sensor design.
Adopting effective shielding methods or using selective surface coatings on electrodes can help mitigate these interferences. This demands additional research but is fundamental for ensuring the biosensor functions effectively in real-world conditions.
Regulatory and Standardization Challenges
When it comes to adopting biosensors widely, regulatory and standardization challenges loom large. The biosensing landscape is laced with diverse technologies and applications, which can complicate the establishment of uniform regulatory frameworks. Each biosensor might need to prove its reliability through rigorous testing, akin to how a new drug faces clinical trials before it hits the market.
Navigating through this maze can be a daunting task. Different countries have their own regulatory bodies, which could lead to inconsistent standards. For instance, while the FDA has specific guidelines for medical biosensors, similar regulations in the EU may vary considerably.
Additionally, the rapid pace of biosensor innovation often outstrips the ability of regulatory agencies to adapt policies. This can result in significant delays for market approval, stalling potentially life-saving technologies from reaching those who need them. To address these hurdles, it is essential for developers and regulatory bodies to engage in dialogue early in the development process, allowing for clearer pathways towards approval.
Biosensor development represents a critical intersection of technology and public health. Overcoming these challenges is not just about improving products but ensuring safety and effectiveness in diverse applications.
By addressing these challenges head-on, the biosensor community can innovate responsibly while paving the way for robust and reliable sensing solutions.
Future Directions in Biosensing Technology
As we stand on the brink of unprecedented advancements in scientific technology, the future directions in biosensing technology promise to push the boundaries of what we consider possible. In this rapidly evolving landscape, several key elements warrant our attention. Each of these directions not only contributes to the development of more sophisticated biosensors but also paves the way for practical applications that can have a significant impact on healthcare, environmental monitoring, and food safety.
Integration with Nanotechnology
The union of biosensing and nanotechnology is a game changer. When we think about nanotechnology, we typically think about things at a scale so small that it’s almost mind-boggling—particles that are measured in nanometers. This precise manipulation of materials can lead to the development of biosensors that are faster, more sensitive, and capable of detecting a wider range of biomolecules. One of the significant benefits of integrating nanotechnology into biosensors is the enhancement of their performance.
For instance, gold nanoparticles have shown great promise for use in electrochemical sensors. When you pair these particles with biological elements, the result is not just an increase in sensitivity, but also the ability to operate in variable conditions. It’s like having a Swiss Army knife that can perform numerous tasks with impeccable skill.
- Enhanced Sensitivity: Nanomaterials can increase the surface area of biosensors, allowing for better interaction with target molecules.
- Miniaturization: Smaller devices make monitoring more convenient and less intrusive for patients in medical applications.
- Cost Reduction: With the potential for mass production of nanomaterials, the overall costs of biosensor systems could significantly decrease.
Advancements in Wearable Biosensors
Another significant direction is the evolution of wearable biosensors. These devices seamlessly blend technology into everyday life. Imagine being able to monitor your health metrics in real time without the need for intrusive methods. Wearable biosensors can track various biomarkers, from glucose levels to hydration status, and provide feedback directly to the user or healthcare providers.
The design of these biosensors is becoming increasingly sophisticated. New materials such as flexible electronics and skin-adhesive sensors are paving the way for devices that are not only functional but also comfortable to wear. Key points about these advancements include:
- Real-time Monitoring: Patients and users can gain instant insights about their health, empowering proactive management.
- Data Integration: Many of these devices can sync with smartphones or cloud services, making it easy for users to track their health data over time.
- Personalization: Wearable sensors can be adjusted to the specific needs of an individual, creating tailored health management plans.
Artificial Intelligence and Machine Learning Applications
Artificial Intelligence (AI) and Machine Learning (ML) are transforming the way we approach biosensing. These technologies can significantly enhance data interpretation, offering a deeper understanding of complex biological signals. Incorporating AI into biosensors enables real-time data analysis and decision-making capabilities that were previously unattainable.
For instance, with advanced algorithms, we can train systems to recognize specific patterns associated with diseases. Imagine a wearable biosensor that not only monitors your heart rate but also uses AI to analyze this data and predict potential health issues before they arise. This could be a breakthrough for preventive healthcare approaches.
- Predictive Analytics: AI can help forecast health issues based on historical data patterns, enabling timely interventions.
- Automated Data Interpretation: Machine learning can streamline the process of analyzing vast amounts of data, making it easier to identify anomalies or concerning trends.
- Enhanced User Experience: AI can improve user interfaces by providing more intuitive and user-friendly experiences in biosensing applications.
In a nutshell, the future landscape of biosensing technology holds vast potential. By aligning developments in nanotechnology, wearable devices, and artificial intelligence, we could see profound changes that enhance not just our understanding of biology but also the way we interact with our environments—ultimately leading to healthier lives and a better world.
"Technology has the power to change the narrative of health care; biosensors at the intersection of biology and engineering are the storytellers for this new chapter."
The continuous evolution of these technologies stands as a beacon of hope for numerous sectors including health, environmental science, and food safety, giving rise to a future that is informed, connected, and healthier.
Closure
In wrapping up our exploration of biosensors, it's paramount to underscore the relevance of this subject in today's scientific landscape. The discussion has traversed the intricacies of biosensing technology, illustrating how they are not merely tools, but pivotal players in addressing some of the pressing concerns across diverse fields, from healthcare to environmental monitoring.
Recap of Key Points
Biosensors, as we have seen, encompass various elements and applications:
- Definition and Mechanisms: They employ biological materials to detect chemical substances, showing remarkable sensitivity and specificity.
- Types: Enzyme-based, immunosensors, DNA biosensors, and cell-based biosensors each serve unique functions tailored to specific detection needs.
- Applications: Their relevance spans across medical diagnostics, environmental monitoring, food safety testing, and even bioprocess control, emphasizing their versatility.
- Advantages: High sensitivity, rapid response times, and cost-effectiveness position them as vital tools in modern science.
- Challenges: Despite their benefits, challenges like stability issues, environmental interferences, and the need for regulatory standards persist, necessitating ongoing attention.
- Future Directions: Advancements incorporating nanotechnology, wearable biosensors, and AI applications hint at exciting prospects ahead.
The Importance of Continued Research
The importance of continued research into biosensors cannot be overstated. With the world continually evolving, new pathogens and environmental challenges arise. Research plays a crucial role in:
- Innovation: Finding novel materials and methods to enhance biosensor performance helps keep pace with the rapidly changing landscape of technological needs.
- Enhancing Sensitivity and Specificity: Continuous investigation is essential to improve detection limits and minimize false positives and negatives.
- Real-world Applications: As researchers explore real-world scenarios, they can gather data that leads to practical applications and real-life solutions.
As we advance into a future where biosensors could augment our understanding and management of health and environmental challenges, it's clear that the synergy between biology and technology will only grow stronger. Thus, fostering a culture of research and development in biosensing technologies will pave the way for innovative solutions that not only function optimally but also contribute significantly to societal well-being.