Exploring EM Science Chemicals: Innovations and Implications
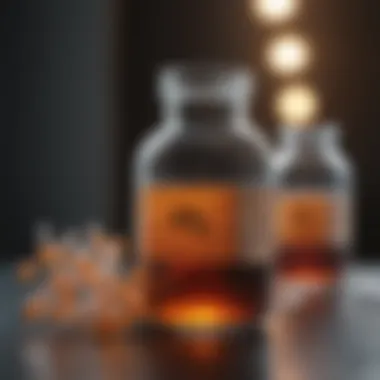
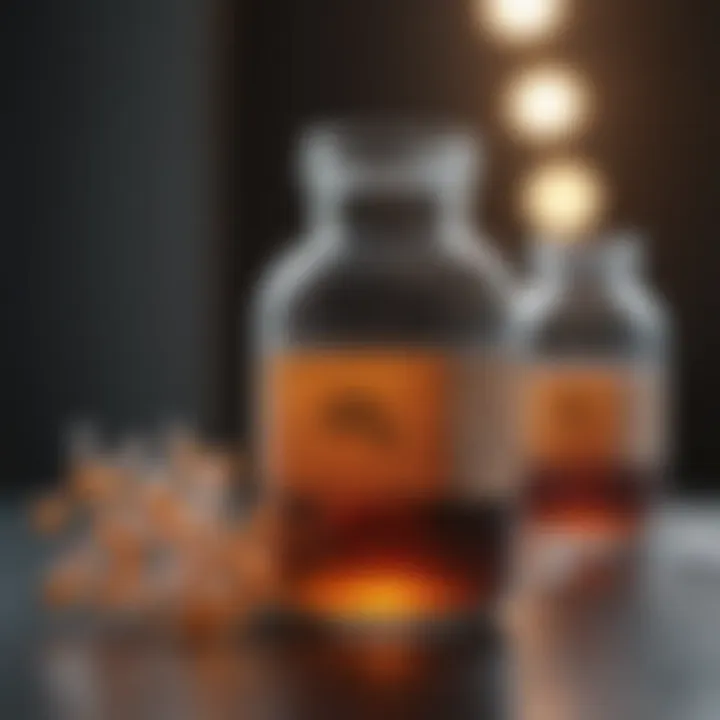
Intro
EM science chemicals play a significant role in various sectors, including research, industry, and environmental science. These chemicals are pivotal in driving innovation and enhancing our understanding of numerous scientific principles. Every study of EM science chemicals contributes to a body of knowledge that has wide-reaching implications. This exploration covers not just their definition, but also the critical aspects of their applications and innovations.
Understanding EM science chemicals can involve a complex interplay of disciplines such as chemistry, materials science, and environmental science. As research in these areas advances, so does the potential for new applications. In recent years, several breakthroughs have highlighted the importance of these substances in technological advancements and sustainability efforts.
To fully appreciate the impact of EM science chemicals, it is essential to consider both historical and contemporary perspectives. This dive into the subject aims to provide an expansive view of the implications of these chemicals, outlining how they shape research and industry today.
Research Highlights
Key Findings
The exploration of EM science chemicals has yielded several key findings:
- Innovative Applications: New applications in sectors like renewable energy, biotechnology, and environmental remediation have been discovered.
- Interdisciplinary Connections: The study of EM science chemicals reveals strong connections between disciplines, helping integrate knowledge across fields.
- Environmental Impacts: Understanding the implications on the environment is pivotal, as many EM science chemicals can have both beneficial and harmful effects.
"The deployment of EM science chemicals has the potential to revolutionize sectors while also posing significant environmental challenges if not managed correctly."
Implications and Applications
The implications of findings in EM science chemicals extend beyond theoretical knowledge:
- Industry Applications: Industries such as pharmaceuticals, agriculture, and materials engineering have begun to utilize these chemicals for enhancing product formulations and efficiencies.
- Sustainability Practices: As the world focuses on sustainable practices, the role of EM science chemicals in developing eco-friendly materials becomes increasingly vital.
- Public Health: Innovations in EM science chemicals help improve public health through the development of safer products and more effective treatments.
Methodology Overview
Research Design
Research on EM science chemicals employs a blend of observational studies, experimental methods, and theoretical modeling. A comprehensive approach is taken to capture the complexities involved in their behavior.
Experimental Procedures
The experimental procedures vary, but often include:
- Synthesis: Creating novel compounds with desired properties.
- Characterization: Using techniques such as spectroscopy and chromatography to analyze chemical properties.
- Testing: Evaluating the practical applications in laboratory environments and field tests.
Through these methodologies, researchers can gain insights that enhance our understanding and application of EM science chemicals.
Prelude to EM Science Chemicals
The field of EM science chemicals plays a significant role across various scientific disciplines. Their impact is seen in research labs, industrial processes, and even in environmental management. Understanding EM science chemicals lays the foundation for exploring more advanced topics in chemistry and related fields. This article seeks to introduce the fundamental concepts, historical background, and various applications of EM science chemicals.
Definition and Scope
EM science chemicals refer to a broad range of chemical substances utilized in various scientific applications. These include solvents, reagents, and compounds specifically formulated for research and industrial work. Each chemical has distinct properties that make it suitable for certain tasks, highlighting its versatility and importance.
The scope covers not only traditional uses in laboratories but also extends to innovative applications in technology, manufacturing, and environmental science. The significance of these chemicals cannot be understated, as they are essential for discoveries that drive scientific advancement.
Historical Context
The evolution of EM science chemicals is rich and intricate. Initially, the development of chemical substances was rudimentary, focusing largely on isolated compounds. However, as scientific methodologies advanced in the 20th century, particularly with the advent of modern chemistry, the formulation of EM science chemicals gained momentum.
Developments in analytical techniques such as spectroscopy and chromatography further allowed scientists to refine and explore the behavior of various chemicals. These tools enabled researchers to identify chemical properties and potential applications more effectively. Over time, this knowledge has underscored the importance of EM science chemicals in contemporary research, industry, and environmental initiatives.
"The growth in EM science chemicals has been driven by the need for more effective solutions in research and industrial settings, impacting societal progress overall."
The interplay of chemical science and technology continues to evolve, providing endless possibilities for future innovations. Understanding this historical context can shed light on current practices and guide future research in the field.
Fundamental Properties of EM Science Chemicals
The fundamental properties of EM science chemicals form the backbone of understanding their various applications and implications in different fields. By examining the chemical composition, physical properties, and behavior under various conditions, we can gain insights into how these substances can be manipulated and utilized effectively. A thorough grasp of these properties not only enhances scientific inquiry but also informs industrial practices and environmental strategies.
Chemical Composition
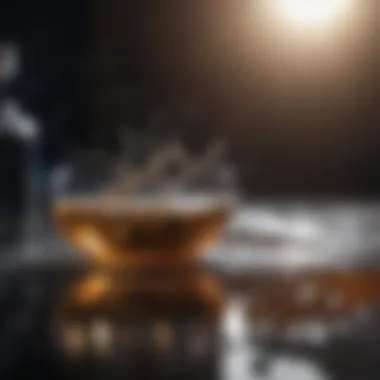
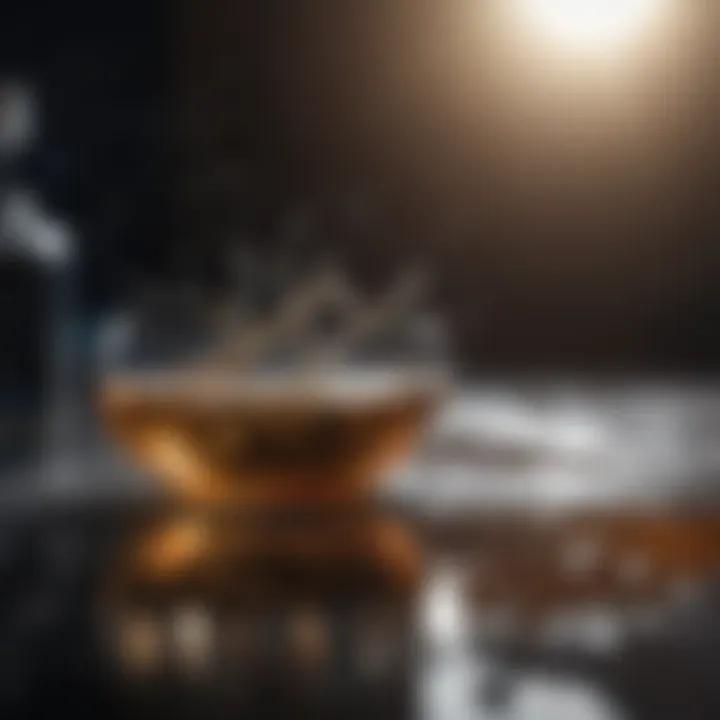
Chemical composition refers to the specific arrangement of atoms and molecules within a substance. EM science chemicals can encompass a broad array of compounds, including organic, inorganic, and polymeric materials. Their composition dictates reactivity, stability, and compatibility with other substances.
- Understanding Elements and Compounds: Each EM science chemical contains unique elements, which determine its baseline characteristics. For example, compounds containing carbon — like polyethylene — exhibit different behaviors compared to silicon-based materials such as silicon carbide.
- Quantitative Analysis: The proportion of elements in a compound can significantly influence its application. Techniques like spectroscopy and chromatography are vital for analyzing these compositions, helping researchers identify and innovate with new materials.
- Influencing Properties: The arrangement of atoms can create compounds with desired traits. For instance, controlling the molecular structure of a chemical can tailor its strength, flexibility, or thermal conductivity for specific applications.
Physical Properties
Physical properties illuminate the observable characteristics of EM science chemicals, such as melting points, boiling points, density, and solubility. These traits are crucial for practical applications as they often guide selection based on performance requirements.
- Thermal Properties: The ability of a chemical to withstand or conduct heat affects its usability in various industries. Materials like polymethyl methacrylate, commonly used in optics, require specific thermal properties to avoid distortion under heat.
- Mechanical Properties: Tensile strength and elasticity are important for materials used in construction or product design. Knowledge of these traits helps engineers select appropriate materials for structures or products, ensuring safety and durability.
- Behavior with Solvents: Solubility plays a significant role in processes like drug formulation and chemical synthesis. Understanding which solvents dissolve certain EM science chemicals can optimize formulations for effective delivery in medical or industrial applications.
Behavior in Various Conditions
The behavior of EM science chemicals under different environmental conditions determines their functionality and applicability. Understanding these behaviors is essential for identifying suitable uses in real-world applications.
- Reactivity: Some chemicals are stable under normal conditions, while others may react vigorously with water or air. For instance, certain alkali metals react explosively with moisture. Knowing these behaviors aids in ensuring safety in both storage and application.
- Mechanical Stress: The physical resilience of materials when subjected to force can vary widely. Research on fatigue and fracture mechanics is crucial for developing materials that can withstand realistic operational stresses.
- Impact of Temperature and Pressure: Many chemicals exhibit altered properties under extreme heat or pressure. For example, polymers might soften when heated, while certain materials become brittle at low temperatures. Understanding these behaviors is fundamental for processes like manufacturing and material testing.
"The properties of EM science chemicals are not just numbers and measures; they represent the potential for innovation and practical application across a spectrum of disciplines."
In summary, mastering the fundamental properties of EM science chemicals enhances our comprehension of their roles across research, industry, and environmental contexts. This knowledge is crucial for driving forward innovations that meet current and future demands.
Applications of EM Science Chemicals
The applications of EM science chemicals are crucial for understanding their impact across various fields. This section highlights their significance in research, industry, and environmental science. EM science chemicals serve as essential tools that enable advancements and innovations, transforming scientific knowledge into practical applications. The focus on these applications underscores not only their versatility but also their undeniable contributions to society and technological progress.
In Research
Laboratory Research
Laboratory research involving EM science chemicals plays a significant role in advancing scientific understanding. Researchers utilize these chemicals to carry out experiments that can lead to groundbreaking discoveries. One key characteristic of laboratory research is its controlled environment, which allows for precise observations and measurements. This aspect makes laboratory research a favored choice for scientists aiming to validate hypotheses. It also enables the manipulation of variables, hence offering a clearer understanding of chemical behavior.
One unique feature of laboratory research is the ability to replicate experiments. This reproducibility is vital for establishing reliability in results. However, there are downsides, such as the limitations on scalability. While laboratory findings can be profound, they may not always translate to practical applications in real-world scenarios.
Field Studies
Field studies provide a different perspective on the applications of EM science chemicals. These studies bring research into natural settings, allowing for observations in real-time. A notable characteristic of field studies is their ability to capture complex interactions within ecosystems. This makes them invaluable for understanding the broader implications of EM science chemicals on the environment.
Field studies also feature an advantage in terms of ecological validity. Findings often resonate more with real-world situations. They help bridge the gap between theoretical research and practical applications. However, they may face challenges, such as less control over variables and external factors that can influence outcomes.
In Industry
Manufacturing Processes
In the industrial realm, EM science chemicals are pivotal in manufacturing processes. These chemicals enhance production efficiency and product quality. One significant element of manufacturing processes is the application of EM science chemicals in the formulation of materials, where they improve strength, durability, and other characteristics. This makes them a prominent choice for companies aiming to optimize their operations.
A unique aspect of these processes is their adaptability. EM science chemicals can be tailored to meet specific manufacturing requirements. However, challenges arise, including regulatory compliance and potential environmental impacts. Industries must navigate these issues while striving to innovate.
Pharmaceuticals
Pharmaceutical applications of EM science chemicals are particularly noteworthy. These chemicals are crucial for drug development and formulation. They contribute to the efficacy and safety of medical products. One essential characteristic of pharmaceuticals is their reliance on EM science chemicals for creating effective medications tailored to specific health conditions. Their integration into drug formulations is popular for researchers and pharmaceutical companies alike.
The unique feature of pharmaceuticals is the rigorous testing and regulations they undergo. This ensures safety and effectiveness, which is vital for public health. However, the complexity and cost of bringing new drugs to market can be significant barriers for research and development.
In Environmental Science
Pollution Control
Pollution control represents a vital application of EM science chemicals in environmental science. These chemicals are employed to mitigate the effects of pollutants on ecosystems. One key characteristic of pollution control efforts is their focus on developing solutions to reduce harmful substances in the environment. This makes them essential tools for environmental agencies.
A unique feature of pollution control measures is their integration with technology. For instance, chemical treatments can enhance wastewater management systems. This is an advantage, enabling more efficient treatment processes. Nonetheless, reliance on chemical solutions can raise concerns about their long-term effects on the environment.
Restoration Efforts
Restoration efforts in environmental science utilize EM science chemicals to rehabilitate ecosystems affected by human activity. These efforts aim to restore habitats, improve biodiversity, and promote ecological balance. One notable characteristic of restoration efforts is their collaborative nature, often involving a variety of stakeholders.
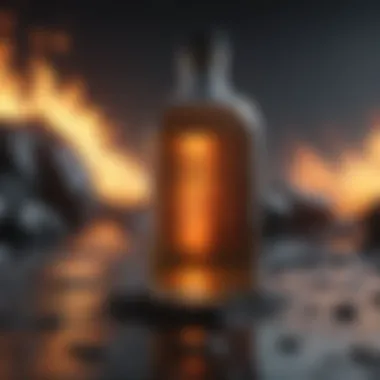
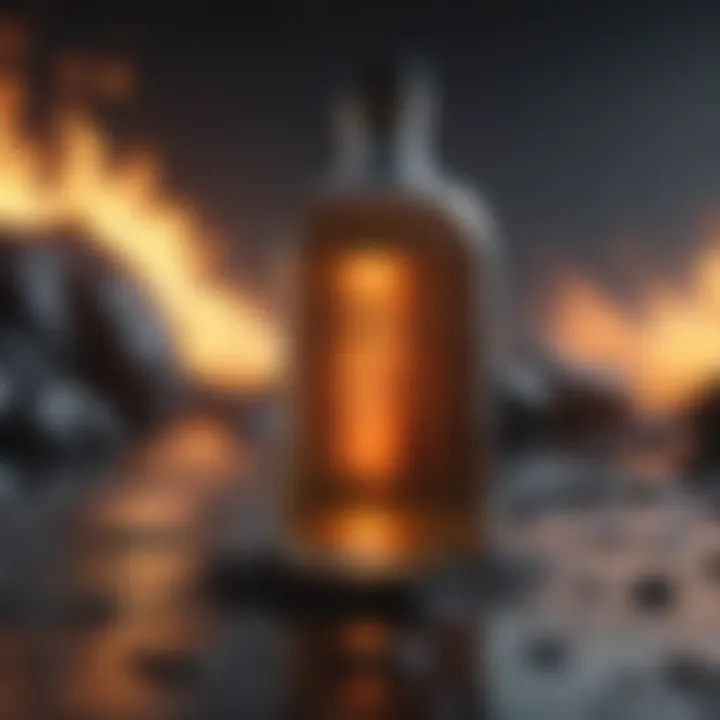
The unique feature of restoration projects is the use of EM science chemicals to repair damage caused by pollution or habitat loss. This can lead to impressive results in regenerating degraded areas. However, challenges include ensuring sustainability and effectively monitoring the outcomes of such projects.
"The effective application of EM science chemicals is pivotal for driving change across different sectors, influencing everything from research breakthroughs to environmental sustainability."
In summary, the applications of EM science chemicals are extensive and impactful. They play a vital role in research, industry, and environmental efforts, advancing knowledge and practical solutions to real-world problems.
Impacts of EM Science Chemicals on Society
The role of EM science chemicals extends beyond mere application in research or industry. Their impacts ripple throughout society, affecting health, the economy, and ethical considerations. This section seeks to unpack those impacts in detail, emphasizing their importance in multiple facets of daily life and future advancements.
Health Implications
Health is arguably one of the most significant areas influenced by EM science chemicals. From pharmaceuticals to agricultural practices, chemicals play a dual role. On one hand, they help in developing life-saving medicines. For instance, compounds derived from EM science chemicals are fundamental in creating antibiotics and vaccines. On the other hand, the misuse or overexposure to certain chemicals can have detrimental health effects.
- Pharmaceutical Applications: Medications like ibuprofen and antibiotics are produced through chemical processes that rely heavily on EM science chemicals. This has led to increased life expectancy and improved quality of life.
- Toxic Exposure Concerns: Some chemicals, if not managed properly, can lead to health concerns such as cancer or respiratory diseases. The balance between utilizing chemicals for health benefits and monitoring their safety is crucial.
"While EM science chemicals advance medical science, their safety must be prioritized to prevent adverse health effects."
Economic Considerations
The economic impact of EM science chemicals is multifaceted. Industries that depend on these chemicals contribute significantly to local and global economies. Their applications in manufacturing, pharmaceuticals, and agriculture yield enormous financial benefits, yet this is coupled with challenges.
- Job Creation: The production and innovation surrounding EM science chemicals create numerous jobs across various sectors. From research scientists to factory workers, the chemistry industry offers diverse employment opportunities.
- Market Dynamics: The demand for innovative chemicals can drive market growth, leading to further investment and exploration. However, fluctuations in market demand or regulations can pose economic challenges.
- Cost of Regulation: Ensuring safety and compliance with regulations leads to additional costs for companies. Balancing safety with profitability is a persistent challenge in the industry.
Safety and Ethics
Safety and ethical considerations surrounding EM science chemicals are vital in maintaining a societal framework based on trust and accountability. Transparent practices must be upheld to ensure safe usage, sustainability, and ethical sourcing.
- Sustainability Practices: Increasing awareness of environmental issues has led to demand for greener chemical production. This involves adopting principles of green chemistry, which focus on reducing waste and energy usage.
- Ethical Sourcing: Ethical implications also revolve around the sourcing of materials required for chemical production. Fair trade practices must be evaluated to guarantee that chemicals do not exploit vulnerable populations.
- Public Transparency: Clear communication regarding the risks and benefits of EM science chemicals must be prioritized. Public understanding fosters trust and allows individuals to make informed decisions.
Recent Innovations Related to EM Science Chemicals
Recent innovations in EM science chemicals reveal their growing importance in various fields, driven by the need for more efficient, sustainable, and versatile chemicals. As scientific understanding evolves, so too does the innovation surrounding the synthesis and analysis of these chemicals. This section explores key advancements in synthesis techniques and analytical methods that are shaping the future of EM science chemicals, highlighting their implications for research, industry, and environmental management.
Synthesis Techniques
Nanotechnology Applications
The application of nanotechnology in the synthesis of EM science chemicals represents a significant step forward in chemical science. This technology involves manipulating matter at extremely small scales, typically at the nanoscale level, to develop new materials with unique properties. One key characteristic of nanotechnology applications is their ability to enhance reactivity and selectivity, making them highly effective for specific chemical reactions.
Nanotechnology is a popular choice due to its potential to innovate traditional synthesis methods. For instance, the use of nanoparticles can improve catalyst efficiency, leading to lower energy consumption during chemical reactions. A unique feature of this approach is the increased surface area of nanoparticles, which enhances their interaction with reactants.
However, the advantages are countered by some disadvantages, such as potential environmental and health risks associated with certain nanoparticles. Thus, careful consideration and thorough assessment are crucial when integrating nanotechnology into the field.
Green Chemistry Principles
Green chemistry principles emphasize designing chemical processes that reduce waste and minimize toxicity. This aspect is vital for developing EM science chemicals that align with sustainable practices. The key characteristic of green chemistry is its focus on safety and environmental health.
Green chemistry is viewed as a beneficial choice in this context because it promotes the use of renewable resources and encourages the development of safer alternatives to hazardous substances. A unique feature of this approach is its application in process design, where the aim is to create chemical processes that avoid the creation of waste altogether.
Despite its advantages, green chemistry may face challenges, including higher initial costs or the need for new types of equipment. Nonetheless, its integration into EM science represents a crucial forward step toward sustainability in chemical research and application.
Analytical Methods
Spectroscopy
Spectroscopy is a powerful analytical method used to identify and characterize EM science chemicals. This technique involves studying the interaction between matter and electromagnetic radiation. One key characteristic of spectroscopy is its versatility, capable of providing information about molecular structure, composition, and concentration.
This method is a popular choice for analyzing EM science chemicals due to its non-destructive nature, allowing for the analysis of samples without altering them. A unique feature of spectroscopy is its ability to provide real-time data, which is invaluable in both research and industrial applications.
However, while spectroscopy is highly effective, it may require specialized equipment and training for accurate interpretation, which can be a barrier for some researchers and institutions.
Chromatography
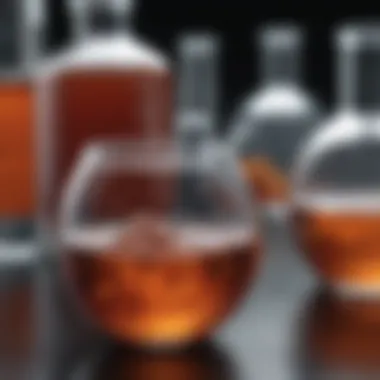
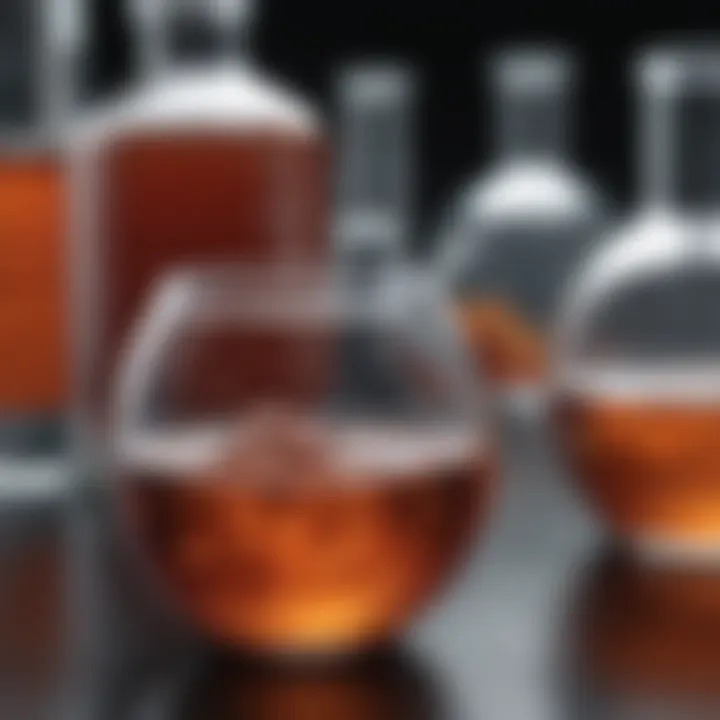
Chromatography is another critical analytical technique extensively used in the analysis of EM science chemicals. This method separates components in a mixture, facilitating the identification and quantification of individual substances. Its key characteristic lies in its effectiveness in isolating compounds based on their interaction with stationary and mobile phases.
This method is advantageous because it allows for high-resolution separation, making it an essential tool in both research and quality control in industries. A unique feature of chromatography is its adaptability; various types include gas chromatography and high-performance liquid chromatography, tailored for different applications.
Yet, chromatography can be resource-intensive and may require complex setups for certain types of analyses. Despite these challenges, it is an indispensable technique for ensuring the purity and efficacy of EM science chemicals.
"The ongoing research in synthesis techniques and analytical methods will shape the future landscape of EM science chemicals, enabling greater innovation in various fields, including pharmaceuticals and environmental science."
Through investigating recent innovations in synthesis techniques and analytical methods, this section highlights how these advancements not only contribute to the fundamental understanding of EM science chemicals but also enhance their practical applications in diverse fields.
Interdisciplinary Connections with EM Science Chemicals
The study of EM science chemicals does not exist in isolation from other scientific disciplines. Instead, it serves as a nexus, bridging concepts and methodologies across biology, physics, and earth sciences. Understanding these interdisciplinary connections is essential as they demonstrate the broader implications of EM science chemicals in various domains. Each discipline offers unique perspectives and tools that enhance research and application outcomes, leading to innovations that can address complex challenges in science and industry.
In Biology
Biology benefits significantly from EM science chemicals, particularly in biochemical research, drug development, and environmental monitoring. Chemical compounds enable researchers to unravel biological mechanisms at a molecular level, assisting in the study of cellular processes and physiological responses. For instance, the use of EM science chemicals in molecular biology has led to advancements in techniques such as PCR and CRISPR, which are pivotal in genetics.
Additionally, in pharmacology, the design and synthesis of new compounds rely on a deep understanding of EM science chemicals. These compounds can act as potential therapeutic agents, offering new treatments for various diseases. The interplay between biology and EM science chemicals is not merely academic; it has tangible impacts on healthcare and wellness.
In Physics
Physics intersects with EM science chemicals primarily through the principles of material science and spectroscopy. Understanding the chemical properties of materials informs their physical behavior and interactions under different conditions. For example, innovations in semiconductor technology are fundamentally rooted in the application of EM science chemicals, leading to better electronic devices.
Spectroscopy, a crucial tool in both physics and chemistry, utilizes the properties of EM science chemicals to identify substances and understand their behavior. This relationship has implications in fields ranging from nanotechnology to quantum physics, enabling scientists to explore and manipulate materials at the atomic level. The collaboration creates an interdisciplinary dialogue that leads to significant advancements in both fields.
In Earth Sciences
The relevance of EM science chemicals in earth sciences is multi-faceted, particularly in areas such as geochemistry and environmental science. EM science chemicals play a vital role in understanding soil composition, mineralogy, and the behavior of pollutants in ecosystems. Techniques employed to analyze chemical reactions in various environmental contexts can help in developing effective pollution control measures and assessing natural resource availability.
Furthermore, the application of EM science chemicals in climate studies enhances our understanding of atmospheric chemistry and the interactions between various environmental factors. Modeling the impacts of chemicals in these studies can provide insights essential for conservation and restoration efforts addressing environmental degradation. This interdisciplinary approach encourages holistic problem-solving, integrating knowledge from various scientific fields to tackle pressing global challenges.
Challenges and Future Perspectives
The exploration of EM science chemicals presents numerous challenges and future perspectives that are crucial for the continued advancement of the field. Understanding these aspects helps address barriers while maximizing the benefits that EM science chemicals can offer across various domains. Identifying challenges allows researchers and practitioners to develop strategies that can lead to improved practices and innovations. Therefore, examining the current issues and envisioning future directions is instrumental for making meaningful progress.
Research Limitations
Research in EM science chemicals often faces limitations that can hinder progress. One significant limitation is the availability of data on various chemicals' long-term effects, particularly regarding their environmental impacts. Lack of extensive research on specific chemicals can result in gaps in understanding their behavior in different conditions. These gaps create uncertainty when developing regulations or applications in sensitive fields like environmental science. Additionally, funding for comprehensive studies is often limited. Researchers might struggle to secure the necessary support for long-term experiments or multifaceted studies that require significant resources. These constraints can delay advancements and leave unanswered questions that pose risks.
Policy and Regulation Issues
Policy and regulation play a critical role in controlling the use of EM science chemicals. However, the complexity of chemical interactions with biology and ecology makes it challenging to create effective regulations. Policymakers must balance innovative use with ensuring public safety and environmental sustainability. Furthermore, existing regulations can lag behind the rapid pace of discoveries. This gap can lead to the use of chemicals without adequate oversight, posing risks of unintended consequences. Another key issue is the inconsistency in regulations across different regions. This creates confusion for industries working internationally. A unified approach is needed to streamline compliance while promoting innovation in chemical applications.
Future Research Directions
Looking forward, several research directions could significantly impact the field of EM science chemicals. One area is the use of artificial intelligence and machine learning for chemical synthesis and analysis. These technologies can enhance accuracy and efficiency, allowing scientists to explore more compounds and their effects. Another promising direction is the integration of green chemistry principles. Research focused on creating sustainable chemicals that minimize environmental harm while maintaining efficacy is crucial. Finally, interdisciplinary collaborations will likely become more essential. Combining insights from biology, physics, and environmental science will lead to a more holistic understanding of EM science chemicals and help drive innovative applications.
"Interdisciplinary collaboration accelerates the pace of discovery, creating a fertile ground for new ideas."
The End
The conclusion of this article serves a crucial role in encapsulating the intricate discussions around EM science chemicals. It is not merely an ending; it synthesizes the information presented throughout the sections.
Summary of Key Points
In our exploration of EM science chemicals, several key points emerged:
- Definition and Scope: EM science chemicals encompass a broad array of substances utilized in various scientific fields. Their applications extend from research to industry, with significant effects on environmental science.
- Applications: These chemicals are pivotal in laboratory research, industrial manufacturing, and pollution control efforts. Without them, advancements in many scientific fields would face considerable limitations.
- Impacts on Society: The implications of EM science chemicals extend beyond mere utility. They raise health considerations, economic factors, and touch on ethical concerns regarding safety in use and disposal.
- Recent Innovations: Innovations in synthesis techniques and analytical methods continue to push the boundaries of what is possible with these chemicals, enhancing their effectiveness in practical applications.
- Challenges: Despite their potential, there are various challenges such as research limitations and regulations that impact their use and development.
By summarizing these points, the conclusion ensures that the reader leaves with a concise understanding of the core elements discussed, reinforcing the significance of EM science chemicals in today's world.
Final Thoughts
In the broader context, the exploration of EM science chemicals invites a deeper appreciation of their role across scientific disciplines. As research progresses, understanding the implications and innovations related to EM science chemicals becomes increasingly vital.
The future of these chemicals lies not only within the boundaries of traditional sciences but also in their interdisciplinary applications. Scholars must remain aware of how these substances can influence societal health, economic development, and environmental sustainability.
Ultimately, the conversation surrounding EM science chemicals must continue to evolve. Ongoing research, robust dialogue among stakeholders, and informed policy-making will ensure that the benefits derived from these chemicals are maximized while minimizing potential risks. By actively engaging in these discussions, the scientific community can pave the way for innovative solutions that align with society's growing needs.