Exploring Liposome Transfection: Mechanisms and Applications
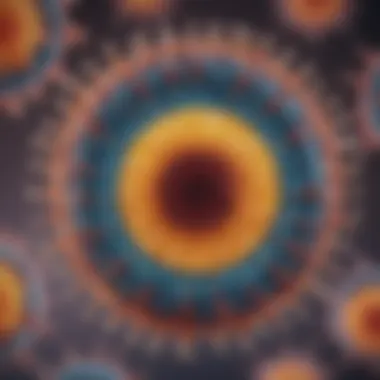
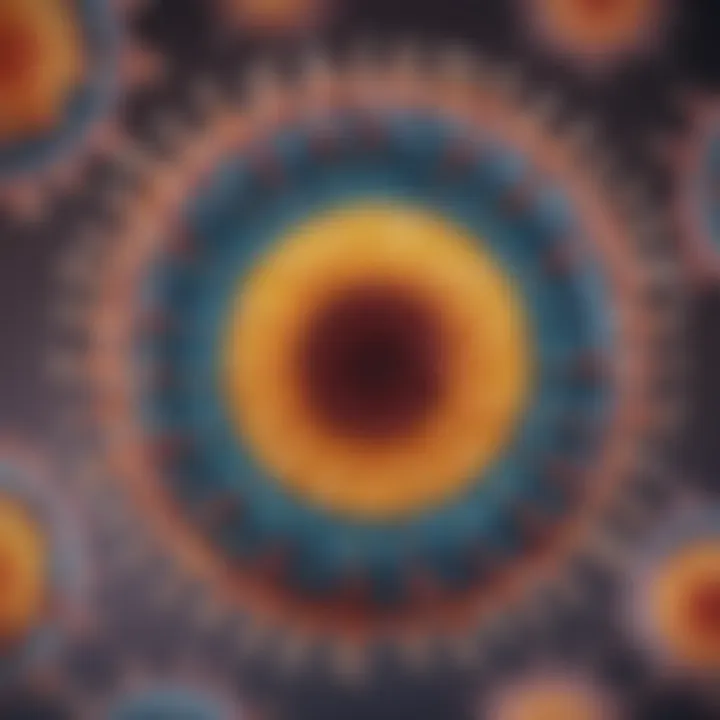
Intro
Liposome transfection has emerged as a pivotal technique in the field of molecular biology and biotechnology. It serves as a vital mechanism for introducing nucleic acids, such as DNA and RNA, into eukaryotic cells. This method leverages liposomes, which are small vesicles made of lipid bilayers, to encapsulate genetic material and facilitate its delivery to target cells.
The significance of liposome transfection cannot be overstated. As the demand for effective gene delivery systems increases, understanding how liposomes work, the different types available, and their applications in gene therapy and vaccine development becomes increasingly crucial. Moreover, challenges in optimizing transfection efficiency continue to drive research into advancing liposome design and functionality.
In this article, we will explore the intricate mechanisms behind liposome-mediated transfection, the various liposome types, and their potential applications. We will also delve into future directions in this area of research, considering how emerging technologies could enhance the effectiveness of liposome transfection.
Preface to Liposome Transfection
Liposome transfection plays a pivotal role in biotechnology and medicine. It serves as a delivery system for nucleic acids, allowing efficient introduction into eukaryotic cells. This method is critical in fields like gene therapy and vaccine development, where successful transfection can make a significant difference. Understanding the mechanisms and principles behind liposome transfection is essential in improving its outcomes and expanding its applications.
Definition of Liposomes
Liposomes are small spherical vesicles composed of phospholipid bilayers. Their ability to encapsulate various molecular payloads makes them valuable in transfection. The life of a liposome begins as a synthetic construct. When these liposomes come into contact with cellular membranes, they can facilitate the delivery of nucleic acids, such as DNA and RNA.
These structures can vary in size and composition, influencing their behavior and effectiveness. Their hydrophilic and hydrophobic properties allow for the incorporation of different types of molecules. Thus, liposomes are versatile tools in the field of molecular biology.
Historical Context
The journey of liposomes in scientific research began in the 1960s. Researchers initially aimed to create lipid-based systems for drug delivery. Early studies demonstrated the ability of liposomes to encapsulate drugs and influence their release. As the understanding of liposomal mechanics deepened, their application in transfection emerged. Over the decades, advancements in formulation techniques have led to improved transfection efficiencies. With the rise of genetic engineering, liposomes became more relevant. Today, they are essential components in biotechnology and therapeutics.
Importance in Genetic Engineering
In genetic engineering, effective delivery of nucleic acids is paramount. Liposome transfection presents several advantages. Firstly, it has a relatively low toxicity profile compared to other methods. This is crucial because high toxicity can compromise cellular integrity and functionality.
Secondly, liposomes offer flexibility in carrying various types of nucleic acids and compounds. They can encapsulate either small or large molecules with relative ease.
"The effective delivery of nucleic acids significantly influences the success of genetic engineering and therapeutic interventions."
Through detailed exploration of liposome transfection, one can appreciate its importance and the potential it holds for advancing scientific research.
Mechanisms of Liposome Transfection
Understanding the mechanisms of liposome transfection is essential for leveraging this technology effectively in various applications, such as gene therapy and vaccine development. By comprehending how liposomes facilitate the delivery of nucleic acids into cells, researchers can optimize and enhance transfection efficiency. There are two primary mechanisms through which liposomes introduce nucleic acids into target cells: entry mechanisms and subsequent release of nucleic acids.
Entry Mechanisms
Endocytosis
Endocytosis is a key mechanism by which liposomes enter cells. It involves the engulfment of the liposome by the cell membrane, leading to the internalization of the liposome along with its cargo. This process is significant because it allows for the delivery of nucleic acids without destroying them in the extracellular environment. One major advantage of endocytosis is its versatility; it can occur through various pathways depending on the conditions and cell types involved. Additionally, it carries a key characteristic of being relatively efficient in many eukaryotic cells, making it a popular choice for genetic engineering applications.
However, endocytosis can also present challenges. For instance, once inside the endosome, liposomes must successfully escape to release their payload into the cytoplasm, which can sometimes be a complicated step.
Direct Fusion
Direct fusion is another pathway through which liposomes can introduce nucleic acids into cells. In this process, the lipid bilayer of the liposome merges with the cell membrane, allowing for the direct release of nucleic acids into the cytoplasm. This mechanism is advantageous because it bypasses some of the barriers associated with endocytosis, primarily by allowing for a more immediate release of the genetic materials. The immediate delivery can enhance the overall effectiveness of transfection, particularly when rapid expression of target genes is desired.
Nevertheless, direct fusion is not without its limitations. It may not be as efficient in certain cell types compared to endocytosis, and the technique typically requires specific lipid formulations to optimize the fusion process.
Release of Nucleic Acids
Endosomal Escape
After liposomes enter the cell via internalization, the next crucial step is endosomal escape. This mechanism ensures that the nucleic acids are freed from the endosomal compartment into the cytoplasm where they can exert their function. Endosomal escape can be considered vital because it determines the success of liposome-mediated transfection. One characteristic that highlights its importance is that the liposomes need to destabilize the endosomal membrane effectively. Various strategies exist to enhance endosomal escape, such as using pH-sensitive lipids or incorporating fusogenic agents. These strategies have made endosomal escape a focal point of research and optimization in liposomal formulations.
Nonetheless, the mechanism also has its drawbacks. Not every liposome can efficiently mediate endosomal escape, which frequently leads to suboptimal transfection outcomes. This aspect requires thorough investigation in tandem with formulation advancements.
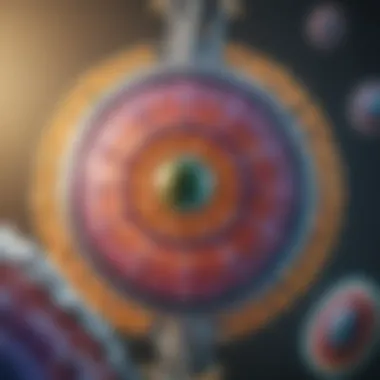
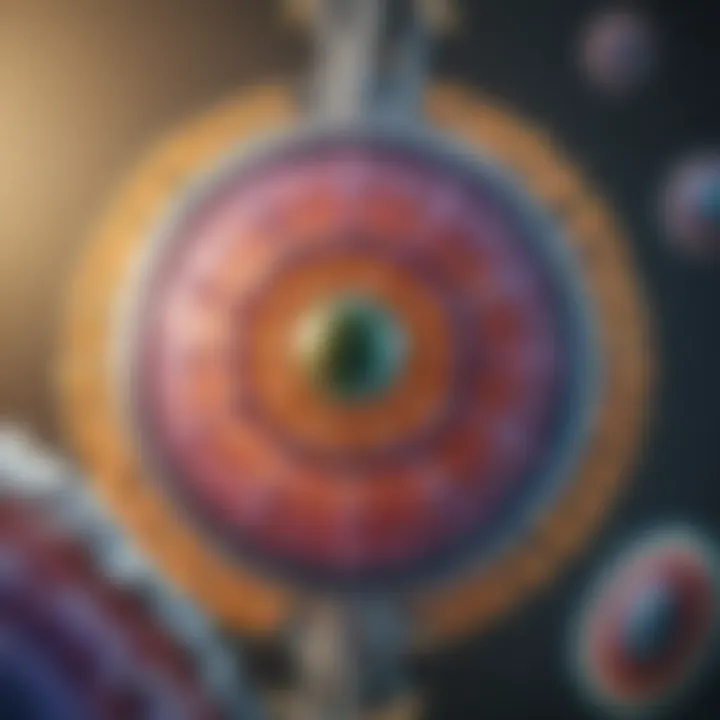
Cytoplasmic Release
Cytoplasmic release is the final step in transporting nucleic acids for functional application. Once nucleic acids have escaped from the endosome, they enter the cytoplasm where translation or transcription can occur. The ability of the system to achieve effective cytoplasmic release can determine the overall success of the delivery mechanism. A key characteristic is that once in the cytoplasm, the nucleic acids must remain stable and intact to assure functionality. The nucleic acids can be RNA or DNA, and different approaches may be necessary for their protection.
On the downside, the cytoplasmic release mechanism can be vulnerable to degradation by cellular enzymes. Thus, developing resistant liposomal formulations can enhance the likelihood of successful genetic expression. Considering both endosomal escape and cytoplasmic release mechanisms lays the groundwork for future innovations in liposome design and transfection efficiency.
Types of Liposomes Used in Transfection
Understanding the types of liposomes is fundamental in the context of liposome transfection, as each type plays a distinct role and has unique attributes. The composition, charge, and structure of these liposomes can significantly influence their efficacy in delivering nucleic acids into target cells. Researchers aim to choose the appropriate liposome type based on the specific requirements of their experiments or therapeutic applications. The evaluation of different liposome types helps optimize transfection efficiency and minimize potential side effects. The following sections will explore the specific characteristics and applications associated with various liposome categories.
Cationic Liposomes
Cationic liposomes are positively charged liposomes. This positive charge is a key property that allows them to interact effectively with the negatively charged components of the cell membrane. Due to this interaction, cationic liposomes are particularly valuable in gene delivery. They facilitate endocytosis, enhancing the uptake of nucleic acids. One major benefit of these liposomes is their ability to condense nucleic acids into small particles, which simplifies their delivery. However, the positive charge can also lead to cytotoxic effects if not carefully optimized. Balancing the charge is crucial to ensure that the transfection is effective without being harmful.
Anionic Liposomes
Anionic liposomes possess a negative charge. This property can present challenges for gene delivery, as they may not interact as readily with cell membranes compared to cationic variants. However, anionic liposomes can still play a role in specific contexts. For example, they are often used in formulations where a neutral or negative surface charge is required to avoid toxicity or immune responses. Their role in controlled drug release systems has made them relevant in research, especially when combined with other delivery methods.
Neutral Liposomes
Neutral liposomes do not have a net charge. This characteristic allows them to avoid strong electrostatic interactions with cell membranes, reducing the likelihood of triggering immune responses. Neutral liposomes can be particularly useful in therapeutic applications where the safety and biocompatibility of the delivery system are paramount. They can encapsulate nucleic acids and deliver them without excessive cytotoxicity. Additionally, neutral liposomes can enhance the stability of formulations, making them suitable for various storage and handling conditions.
Multilamellar vs. Unilamellar
Liposomes can be classified into two main types based on their structure: multilamellar and unilamellar liposomes.
- Multilamellar Liposomes: These vesicles consist of multiple lipid bilayers. They can encapsulate larger quantities of nucleic acids. Their structural complexity may make them beneficial in specific applications requiring high payloads. However, the release of encapsulated materials can be less controlled compared to unilamellar liposomes.
- Unilamellar Liposomes: These liposomes contain a single lipid bilayer. They provide a more uniform release profile, which is advantageous in therapeutic contexts where controlled delivery is needed. Unilamellar liposomes are often preferred when targeting specific cells or tissues, as their simpler structure allows for more precise functionalization with targeting ligands.
Overall, the choice between multilamellar and unilamellar liposomes depends on the specific application and the desired characteristics of the transfection agent.
"Selecting the right type of liposome is essential for effective nucleic acid delivery and can greatly impact the success of gene therapies and vaccine developments."
Factors Influencing Liposome Transfection Efficiency
Liposome transfection is a widely used method for delivering nucleic acids into eukaryotic cells. Understanding the factors that influence transfection efficiency is critical. Higher efficiency ensures effective gene transfer, which is vital in applications such as gene therapy and vaccine development. A myriad of elements come into play, each affecting how well liposomes perform their intended function.
Liposome Composition
The lipid composition of liposomes plays a central role in their ability to facilitate transfection. The ratio of cationic, neutral, or anionic lipids contributes to their stability, interaction with cell membranes, and overall performance. Cationic liposomes, for example, exhibit enhanced transfection efficiency due to their positive charge, which promotes binding to negatively charged cell membranes. The inclusion of specific lipids can also enhance the membrane fusion properties, further supporting the release of nucleic acids into the cytoplasm. An understanding of which lipids work best can guide researchers to optimize formulation for specific applications.
Size and Charge of Liposomes
The size and charge are crucial parameters. Liposome size typically affects cellular uptake; smaller liposomes can enter cells more easily compared to larger ones. However, if they are too small, they may lack stability. The charge of liposomes is equally important. Cationic liposomes are known to exhibit higher transfection rates. However, they can also induce toxicity, which limits their use. Striking a balance between size and charge is essential for maximizing transfection efficiency while minimizing adverse effects.
Cell Type Compatibility
Different cell types respond variably to liposome-mediated transfection. Factors like membrane composition and presence of specific receptors influence how efficiently liposomes can merge with target cells. For example, certain types of cancer cells may have characteristics that allow more effective uptake of liposome-encapsulated nucleic acids. It is essential to consider the compatibility of liposomes with the target cell types to enhance the chances for successful transfection.
Properly addressing these factors is key to advancing liposome transfection technologies.
In summary, the efficiency of transfection is determined by a combination of liposome composition, size and charge, and compatibility with target cells. Optimizing these variables leads to significant improvements in liposomal delivery systems, ultimately enhancing their application in gene therapy and other biomedical fields.
Applications of Liposome Transfection
Liposome transfection is a vital technology in modern biology with a range of applications. Its ability to deliver nucleic acids efficiently into cells has made it popular in several fields, particularly in gene therapy, vaccine development, and research within biotechnology. Each application harnesses the unique advantages that liposome-mediated delivery provides, making it a cornerstone of contemporary scientific initiatives.
Gene Therapy
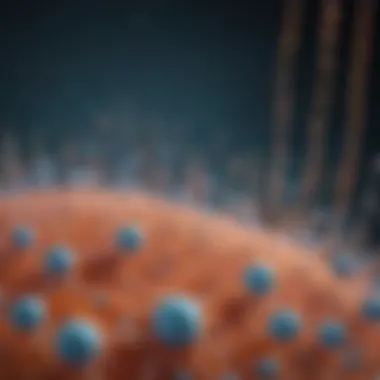
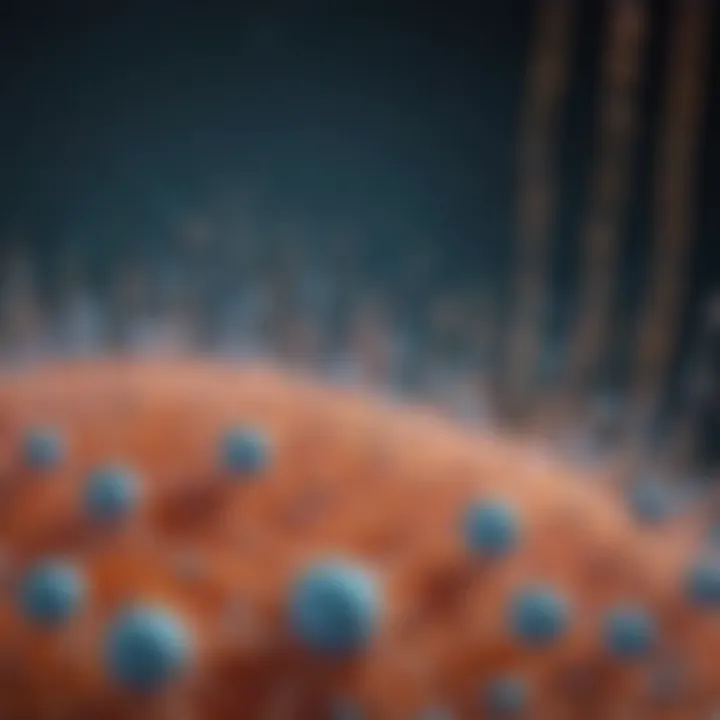
Gene therapy represents one of the most promising applications of liposome transfection. It aims to treat or prevent diseases by introducing genetic material into a patient's cells. This method can correct defective genes or provide new information to help combat diseases such as cancer or inherited disorders. One crucial element of liposome transfection in this context is its efficacy in delivering both DNA and RNA into target cells.
The use of cationic liposomes in gene therapy is particularly noteworthy. These liposomes carry a positive charge, which facilitates interactions with the negatively charged nucleic acids, promoting efficient encapsulation. Furthermore, liposomes can enhance cellular uptake, ensuring that therapeutic genes reach their intended destinations. The versatility and effectiveness of liposome transfection mechanisms make it a popular choice for gene therapy, where precision is essential.
Vaccine Development
Vaccine development has also greatly benefited from advancements in liposome transfection technology. Liposomes serve as vehicles to deliver antigens or genetic material that can elicit an immune response. The ability to harness liposomes in this area is noteworthy, especially with the rise of new vaccine technologies like mRNA and DNA vaccines.
mRNA Vaccines
mRNA vaccines are a specific application of liposome transfection that has gained attention, especially during the COVID-19 pandemic. These vaccines utilize lipid nanoparticles to deliver synthetic mRNA into cells. The key characteristic of mRNA vaccines is their capacity to instruct cells to produce proteins that mimic pathogens, thereby preparing the immune system for future encounters with actual viruses.
The unique feature of mRNA vaccines is their rapid development and the flexibility that comes with modifying the mRNA sequences. This allows for quick responses to emerging infectious diseases. Furthermore, since they do not use live pathogens, mRNA vaccines minimize the risk of infection, making them a beneficial choice for safe vaccine solutions. However, the stability of mRNA in storage and transport remains a challenge that researchers continue to address.
DNA Vaccines
DNA vaccines represent another promising approach that utilizes liposome transfection technology. These vaccines introduce plasmid DNA encoding specific antigens into the host's cells, stimulating an immune response. A notable characteristic of DNA vaccines is their stability, allowing for easier storage and transport compared to other vaccine types.
The unique feature of DNA vaccines is their capability to produce a broad range of immune responses since they can encode multiple antigens. This characteristic makes DNA vaccines versatile in targeting various pathogens. However, there are limitations, including lower transfection efficiency and possible concerns about integrating into the host genome, which require continued research and optimization.
Research and Biotechnology
Beyond gene therapy and vaccines, liposome transfection is a critical tool in research and biotechnology. Researchers utilize this technology to explore gene function, study cellular processes, and develop new therapeutic approaches. The ability to manipulate genetic material effectively enables scientists to conduct high-throughput screening and create genetically modified cell lines for various applications.
In summary, the applications of liposome transfection extend widely across various fields. From its role in transformative therapies to vaccine innovation, this method catalyzes significant advancements in biomedical research. The continued development of liposome technology promises to address existing challenges, enhancing its efficacy and expanding its applications even further.
"The versatility of liposome transfection is integral to modern biomedical techniques, making it an essential area of study for future innovations."
By understanding the nuances of these applications, researchers can leverage liposome technology to its fullest potential, ensuring progress in health and disease management.
Challenges in Liposome Transfection
The field of liposome transfection is not without its difficulties. Understanding the hurdles that researchers face is essential for developing more effective transfection methods. Significant challenges include toxicity concerns, stability issues, and difficulties in delivering larger nucleic acids. Each of these aspects can impact the overall efficiency and success rate of liposome-based transfection in various applications.
Toxicity Concerns
Toxicity associated with liposomal formulations is a critical consideration. While liposomes have improved the delivery of nucleic acids, their potential cytotoxic effects can limit their use in clinical applications. The formulation of cationic liposomes is particularly noteworthy in this context. These liposomes can disrupt cell membranes, leading to unintended damage and inducing apoptosis. The balance between transfection efficiency and cellular safety is delicate.
Key points include:
- Dose-dependent toxicity: Higher concentrations of liposomes may enhance transfection but can also increase cell death.
- Cell type variability: Different cell lines show varying degrees of sensitivity to liposomal agents. What works for one cell type may cause harm to another.
- Formulation additives: Certain components may reduce toxicity. Researchers are experimenting with polymers or alternative lipids to minimize adverse effects while maintaining effectiveness.
Stability of Liposomes
Stability is another pressing challenge in liposome transfection. Liposomes can be prone to aggregation, which can diminish their ability to deliver payloads. In an environment that promotes fusion or collapse, achieving a stable formulation is crucial. Instability can result from factors such as temperature fluctuations, pH changes, and the ionic strength of solutions.
Considerations include:
- Storage conditions: Maintaining appropriate storage conditions is essential for preserving the structure and function of liposomes.
- Formulation composition: Different lipid compositions affect the stability. Phospholipids with longer acyl chains can offer better stability compared to those with shorter chains.
- Freeze-drying: Lyophilization can enhance shelf-life but necessitates protective agents to prevent damage during rehydration.
Delivering Large Nucleic Acids
The delivery of larger nucleic acids, such as plasmids, presents significant hurdles. Traditional liposomal systems often struggle to efficiently encapsulate and deliver larger constructs, which are gaining prominence in gene therapy and related fields. The physical size and the electrostatic nature of large nucleic acids complicate their interaction with liposomal carriers.
Points of concern include:
- Charge interactions: The negative charge of nucleic acids may limit their association with liposomes, especially cationic ones, necessitating innovative designs for improved binding.
- Encapsulation efficiency: Current liposome systems may not adequately encapsulate larger nucleic acids, leading to lower transfection rates.
- Alternative strategies: Future research may explore hybrid systems or different transfection vectors that can better accommodate larger nucleic acids while enhancing delivery efficiency.
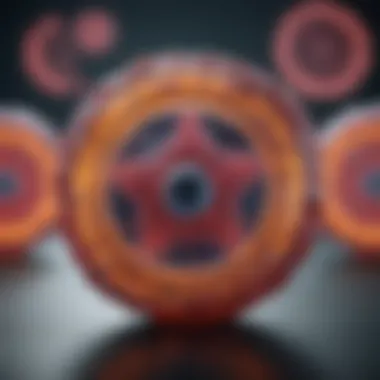
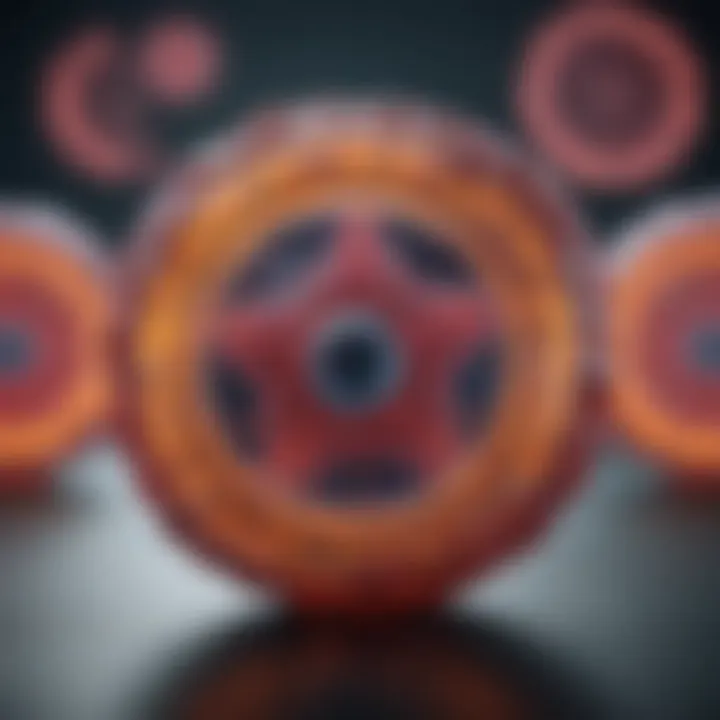
Addressing these challenges will be crucial for advancing the practical applications of liposome transfection, enabling safer and more effective gene therapies and biotechnological innovations.
Recent Advances in Liposome Transfection Technologies
Recent advances in liposome transfection technologies have significantly impacted the efficacy and versatility of nucleic acid delivery systems. As research progresses in the area of gene therapy and vaccine development, understanding these advancements is crucial. Enhanced transfection techniques improve cellular uptake of nucleic acids. They also ensure that delivered molecules reach the intended targets without unwanted toxicity. Thus, these advancements are key to optimizing therapeutic outcomes in biomedical applications.
Functionalization Techniques
Functionalization refers to modifying liposomes to improve their targeting capabilities and interaction with cells. This process is important. By attaching specific ligands or molecules to the liposome surface, researchers can enhance the selectivity of transfection. For instance, ligand-receptor interactions allow liposomes to bind more effectively to certain cell types. This specificity can lead to increased uptake and lower doses needed for effectiveness.
Some techniques used for functionalization include:
- PEGylation: The attachment of polyethylene glycol (PEG) to liposomes to enhance circulation time and evade immune detection.
- Targeted Ligands: Incorporation of antibodies or peptides that bind to receptors on specific cells, improving targeting efficiency.
- pH-Sensitive Modifications: Designing liposomes that release their payload in response to the acidic environment of endosomes can enhance gene delivery capabilities.
The benefits of such functionalization are manifold. Increased delivery efficiency and reduced systemic toxicity extend the therapeutic range of liposome-based interventions.
Nanoparticle Integration
Nanoparticle integration with liposome transfection technologies represents another significant innovation in this field. By combining nanoparticles with liposomes, researchers can enhance the overall performance of transfection vehicles. Nanoparticles may act as carriers for various nucleic acids or even assist in the process of cellular uptake.
The integration often leads to:
- Improved Stability: Nanoparticles can protect nucleic acids from degradation before they reach their target cells.
- Enhanced Efficacy: The combined systems offer synergistic effects, improving the overall transfection rates compared to liposome technology alone.
- Versatile Applications: Integration can facilitate the delivery of different kinds of therapeutics, including RNA, DNA, and even small molecules.
"Innovative integration of nanoparticles with liposomes has opened new pathways for targeted therapy and efficient delivery systems."
These advancements position liposome transfection as a versatile tool in modern biotechnology, promising safe and effective therapeutic strategies in various fields.
Future Directions in Liposome Transfection Research
The exploration of future directions in liposome transfection research is essential. As the field continues to evolve, new designs and applications emerge that could significantly enhance gene delivery effectiveness. This section discusses the innovative approaches in liposome designs and the promising potential of personalized medicine. These factors could reshape the landscape of molecular therapy, providing enhanced treatment options for various medical conditions.
Innovative Liposome Designs
The development of innovative liposome designs represents a pivotal movement in liposome transfection approaches. Recent advancements focus on modifying the liposomal structure to improve targeting specificity and reduce adverse effects. For instance, researchers have started to incorporate targeting ligands on liposome surfaces. This allows selective binding to specific cells, such as cancerous or infected cells, thereby increasing transfection efficiency.
Moreover, creating stimuli-responsive liposomes adds a new dimension to their functionality. These liposomes can release their nucleic acid cargo in response to specific internal or external stimuli like pH changes or light exposure. Such designs improve the controlled release of therapeutic agents, which is critical in reducing side effects and maximizing treatment efficacy.
Additionally, exploring hybrid liposomes that integrate nanotechnology can lead to enhanced stability and delivery capabilities. For example, blending traditional lipids with polymeric materials can provide better protection for the nucleic acids during circulation in the bloodstream, which is a significant challenge in current gene therapy practices. These advancements indicate a shift toward more sophisticated delivery mechanisms that are tailored to specific therapeutic needs.
Potential for Personalized Medicine
The potential for personalized medicine through liposome transfection is an area of growing interest. Personalized medicine aims to tailor treatments to individual patients based on their genetic makeup, lifestyle, and specific disease characteristics. Liposome transfection can play a critical role in this paradigm by facilitating the delivery of gene therapies designed for individual responses.
Consider the advancements in genomic sequencing technologies. With these tools, practitioners can identify specific mutations in a patient's cells. Subsequently, custom liposomes could be designed to deliver corrective gene therapies that directly address these unique mutations. This targeted approach can lead to better outcomes than conventional methods, where treatments are often standardized and not personalized.
Furthermore, the integration of liposome transfection systems with biomarker discovery can enhance decision-making in treatment strategies. Biomarkers can predict response to therapies, and when coupled with liposomal delivery systems, personalized treatment plans can be formulated to suit ongoing patient responses.
Culmination
In this section, we encapsulate the significant insights garnered throughout our exploration of liposome transfection. By examining the varying mechanisms and applications of this technique, we have established that liposome transfection is not merely a method but a critical technological advancement in the fields of genetic engineering and biomedicine.
Summary of Key Points
Several pivotal aspects emerge from the preceding discussions on liposome transfection:
- Mechanisms of Entry: We discussed how liposomes can utilize endocytosis and direct fusion to facilitate the introduction of nucleic acids into cells. Understanding these mechanisms is crucial for optimizing transfection efficiency.
- Varieties of Liposomes: The different types of liposomes—cationic, anionic, neutral, multilamellar, and unilamellar—highlight the versatility of this technology, providing tailored solutions for diverse experimental needs.
- Applications: Liposome transfection has far-reaching implications in gene therapy and vaccine development, underscoring its potential to revolutionize treatment methods, especially in mRNA and DNA vaccine formats.
- Challenges: Addressing toxicity, stability, and the delivery of larger nucleic acids remains essential to improving liposome-mediated transfection protocols.
- Future Directions: Continuous innovation, including functionalization techniques and nanoparticle integration, will drive the future of this technology, potentially shaping personalized medicine.
Final Thoughts on Liposome Transfection
Considering the discussed elements, liposome transfection represents a cornerstone of genetic manipulation techniques. Its importance cannot be overstated as it bridges the gap between theory and practical applications in medicine and biotechnology. As research progresses, the refinement of this technique will likely reveal new modalities for treatment and preventive strategies against diseases. This has profound implications for health technology, personal medicine, and overall therapeutic frameworks.
Engaging with liposome transfection offers insights that extend into broader realms of molecular biology and therapeutic developments. The rigorous exploration of its mechanisms and applications lays the groundwork for potential breakthroughs in numerous biomedical areas.