Exploring Operon Oligonucleotides: Genetic Insights
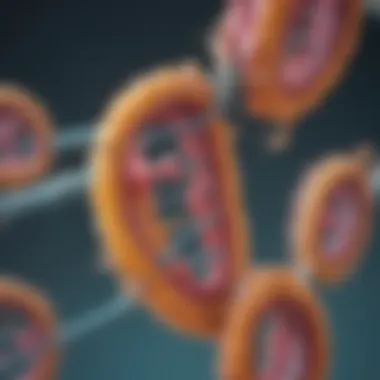
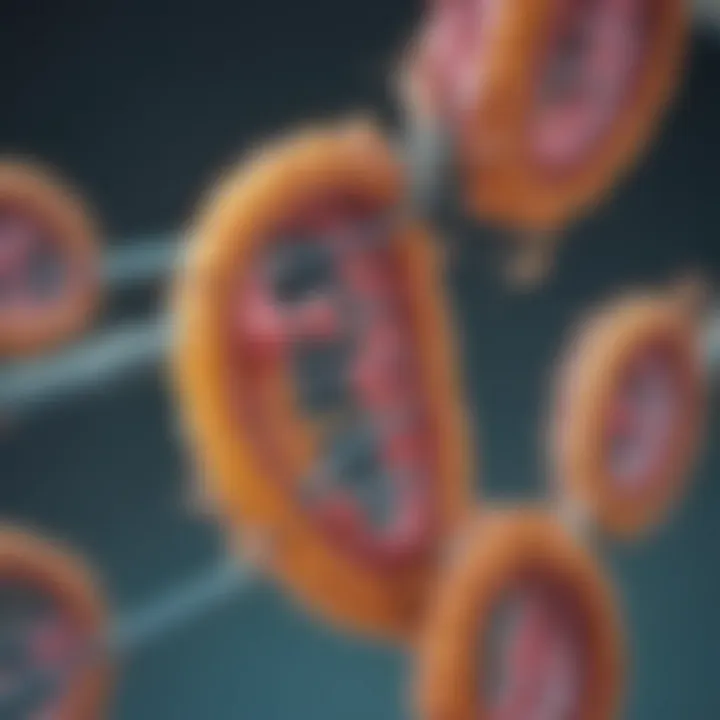
Intro
Operon oligonucleotides represent a crucial intersection of molecular biology and biotechnology. Understanding their role is essential for advancing genetic research. This article delves into the fundamental aspects of operons and oligonucleotides, illustrating their importance in genetic regulation. Moreover, it highlights the methodologies used to explore these concepts, providing insights into their applications in modern science.
Research Highlights
Key Findings
The study of operons and oligonucleotides has unveiled several significant findings:
- Operon Structure: Operons are groups of genes regulated together. They play vital roles in bacterial gene expression and can influence various biological pathways.
- Role of Oligonucleotides: Oligonucleotides are short sequences of nucleotides that can modulate the expression of operons. They can function as primers or probes, thus serving crucial roles in genetic manipulation.
- Applications in Biotechnology: The combination of operon understanding and oligonucleotide functionality has led to numerous applications. These range from gene therapy to the development of genetic circuits in synthetic biology.
Implications and Applications
The implications of operon oligonucleotides extend across several domains, such as:
- Genetic Engineering: Tools like CRISPR-Cas9 rely on the targeted action of oligonucleotides on operons, enabling precise modifications in the genome.
- Medical Diagnostics: Oligonucleotide probes allow for the detection of specific RNA or DNA from operons, which aids in early disease diagnosis.
- Colony Development: In synthetic biology, operons orchestrate the expression of multiple genes simultaneously, contributing to the development of engineered organisms.
"Understanding how oligonucleotides interact with operons can redefine our approach to genetic regulation and molecular biology."
Methodology Overview
Research Design
Studying operon oligonucleotides involves distinct research design methodologies, including:
- In Vitro Studies: Scientists often conduct experiments in controlled environments, mimicking cellular conditions to observe interactions.
- Computational Models: Bioinformatics tools are deployed to predict how oligonucleotides will behave in relation to operons, guiding experimental efforts.
Experimental Procedures
Common experimental procedures applied in this field include:
- PCR Amplification: Utilizing operon-specific primers to amplify DNA sequences.
- Gel Electrophoresis: Separating oligonucleotides to analyze size and purity.
- Sequencing: Confirming the desired genetic modifications at the operon level.
As research progresses, the methodologies will evolve, further illuminating the complex dynamics of operon oligonucleotides.
Overview of Operons
Operons are a fundamental aspect of genetic regulation in prokaryotic organisms. Understanding operons is crucial for comprehending how genes are expressed and regulated in cells. They play a vital role in ensuring that genes coding for related functions are expressed in a coordinated manner, thus allowing efficient cellular responses to environmental changes. In the context of this article, operons serve as a significant focal point when discussing their relationship with oligonucleotides and their applications in biotechnological innovations.
Definition of Operons
An operon consists of a group of genes that are transcribed together under the control of a single promoter. This arrangement allows for the coordinated expression of genes that often have related functions. In bacteria, for example, an operon can enable the rapid production of proteins needed for specific metabolic pathways when conditions are favorable. This means that cells can efficiently allocate resources and energy to produce proteins only when necessary. It represents a unique mechanism for gene regulation, allowing organisms to adapt and thrive in various environments.
Key Components of Operons
Operons comprise several key components, each playing a specific role in the expression of genes:
- Promoter: This sequence of DNA is where RNA polymerase binds to initiate transcription.
- Operator: Located between the promoter and the genes, the operator acts as a regulatory sequence where repressor proteins can bind, blocking transcription.
- Structural Genes: These are the genes that are co-transcribed. They usually code for proteins that work together in a pathway.
- Regulatory Genes: These genes produce proteins (often repressors or activators) that modulate the activity of the operon by binding to the operator region.
Understanding these components is essential for grasping how gene expression is finely tuned in response to internal and external cues.
Types of Operons
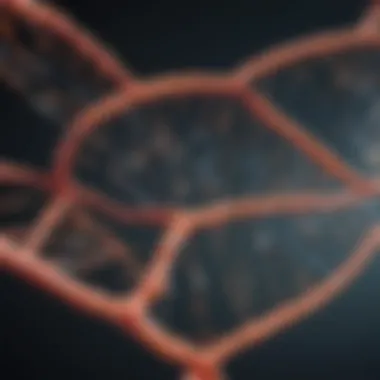
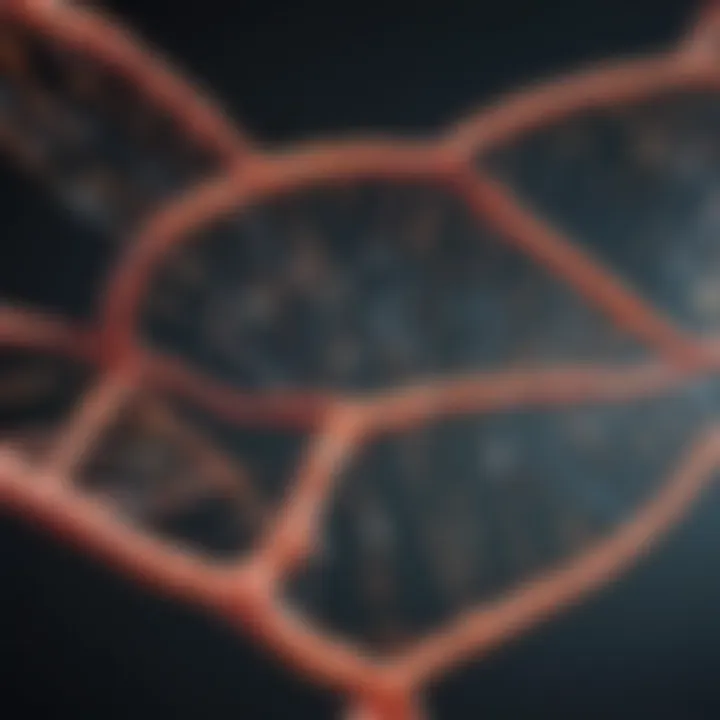
Operons can be categorized into several types, each types reflecting different regulatory mechanisms and responses to environmental signals:
- Inducible Operons: These are normally off but can be turned on in response to an external signal, such as the presence of substrate. A classic example is the lac operon in E. coli, which is activated by lactose.
- Repressible Operons: In contrast, repressible operons are usually active but can be switched off when a specific metabolite is present. For instance, the trp operon, involved in tryptophan synthesis, is repressed when tryptophan levels are high.
- Constitutive Operons: These operons maintain a constant level of expression regardless of environmental conditions.
By studying these different types, researchers can gain insights into the complex network of gene regulation and the mechanisms that bacteria employ to optimize their metabolism. Each operon type has particular implications that can inform various biotechnological approaches utilizing oligonucleotides, as the understanding of operon behavior can direct research toward precise genetic manipulations.
Prolusion to Oligonucleotides
Oligonucleotides are short sequences of nucleotides, critical in the realms of molecular biology and genetics. They play an essential role in various biological processes and biotechnological applications. Understanding this subject provides insight into their use in gene regulation, genetic engineering, and therapeutics. Given their importance, exploring their structure, classification, and synthesis holds substantial value for researchers, educators, and students.
Definition of Oligonucleotides
Oligonucleotides are typically defined as sequences of RNA or DNA that range from around 2 to several dozen nucleotides long. These small fragments serve as fundamental building blocks in genetic research and laboratory practices. Their simplicity is misleading, as they perform complex functions. For instance, they can function as primers in polymerase chain reactions or as probes in hybridization techniques. In molecular biology, clarity in definition is vital, as it underscores their diverse applications and importance.
Classification of Oligonucleotides
There are various classifications of oligonucleotides, each serving unique purposes:
- DNA Oligonucleotides: These are deoxyribonucleic acid sequences used mostly for amplification and sequencing.
- RNA Oligonucleotides: Ribonucleic acid sequences often used in techniques such as RNA interference.
- Modified Oligonucleotides: These include chemically altered versions which enhance stability and specificity. For example, phosphorothioate oligonucleotides offer increased resistance to nucleases.
This classification highlights not only the functional diversity of oligonucleotides but also emphasizes the technological advancements made in their design and application.
Synthesis of Oligonucleotides
The synthesis process of oligonucleotides is crucial and typically involves solid-phase synthesis. This method involves building a nucleotide chain on a solid support, where each nucleotide is sequentially added. The process can be automated, allowing for the rapid production of oligonucleotides in large quantities. The efficiency and precision of this synthesis govern how these molecules are applied in research.
The sequence is crafted carefully, ensuring effective performance in its intended application. Moreover, advancements in synthesis technology have increased the fidelity and yield of produced oligonucleotides. This innovation has allowed researchers to push the boundaries of genetic engineering and other fields, leading to increased possibilities in therapeutic designs and genetic manipulations.
"Oligonucleotides serve as pivotal tools in the understanding and manipulation of genetic material."
Mechanisms of Gene Regulation
Gene regulation is fundamental for controlling cellular processes and ensuring proper gene expression. The mechanisms involved determine how and when genes are activated or silenced. This has significant implications for understanding various biological functions, evolution, and the development of therapeutic interventions. Specifically, the interplay between operons and oligonucleotides is crucial to gene regulation, providing insights into many biological processes.
Role of Operons in Gene Expression
Operons are groups of adjacent genes that are regulated together. They play a vital role in prokaryotic gene expression by allowing coordinated expression of multiple related genes in response to specific signals. For example, the lac operon, found in E. coli, regulates the enzymes involved in lactose metabolism. When lactose is present, the operon is activated, leading to the production of enzymes necessary for its breakdown.
The operon model highlights a few key aspects about gene regulation:
- Coordinated Control: Instead of regulating each gene individually, operons enable simultaneous regulation, which is highly efficient for prokaryotes.
- Response to Environment: Operons respond effectively to environmental changes, allowing organisms to adapt quickly.
- Repressor-Activator Dynamics: Regulatory proteins can enhance or inhibit the operon's function based on the cellular context, affecting gene expression levels.
Interaction of Oligonucleotides with Operons
Oligonucleotides, short sequences of nucleotides, can influence the expression of genes within operons. They participate in several regulatory mechanisms. For example, RNA interference (RNAi) employs oligonucleotides to silence gene expression within operons. By binding to messenger RNA (mRNA), oligonucleotides can prevent translation and reduce protein production.
Moreover, synthetic oligonucleotides are used in research and biotechnology to manipulate genes in operons. This manipulation can lead to:
- Gene Knockdown: Reducing the expression of specific genes within an operon can help in studying gene function.
- Gene Editing: Technologies such as CRISPR rely on guide RNAs, which are oligonucleotides, to target and modify specific genes.
Therefore, understanding the interaction provides tools for novel approaches in genetic modifications.
Regulatory Elements and Their Functions
In the context of operons, regulatory elements are sequences of DNA that interact with proteins to control gene expression. These elements include promoters, operators, and enhancers. Each regulatory component has a crucial role:
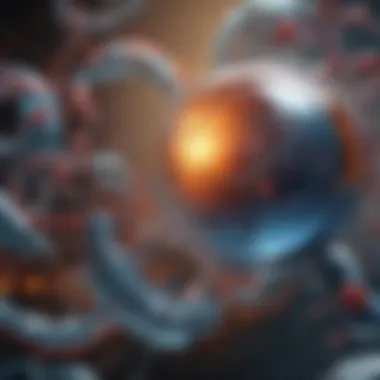
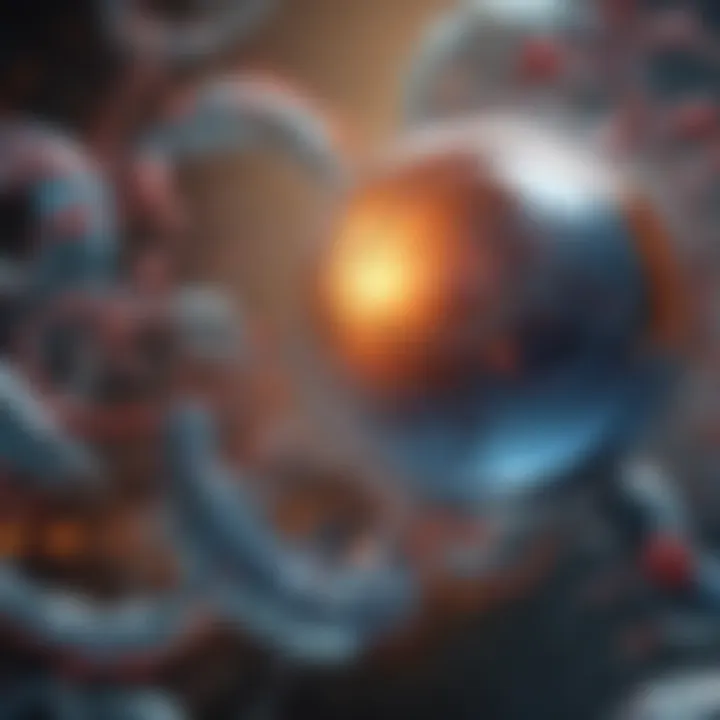
- Promoters: Sites where RNA polymerase binds to initiate transcription. Their strength significantly impacts how often the associated genes are expressed.
- Operators: Sequences that bind repressor proteins. Their interaction prevents RNA polymerase from transcribing the genes.
- Enhancers: These can enhance the activity of a promoter even when they are located far away from the targeted gene.
The exact arrangement of these elements can create unique regulatory profiles, thereby impacting cellular function and adaptability. The relationship between these elements and oligonucleotides provides further insight into fine-tuning genetic expression in response to various stimuli.
Understanding these mechanisms is not only critical for basic biology but informs biotechnological advancements and therapeutic interventions.
Oligonucleotides in Biotechnological Applications
Oligonucleotides play a pivotal role in various biotechnological applications, having transformed the landscape of molecular biology and genetic research. Their utility stems from their ability to specifically bind to nucleic acids, enabling precise manipulation of genetic material. This section elaborates on the significance and diverse applications of oligonucleotides in the biotechnological realm, spotlighting crucial elements, benefits, and key considerations.
Oligonucleotides as Molecular Tools
Oligonucleotides serve as versatile molecular tools. They are primarily used as primers in the Polymerase Chain Reaction (PCR). PCR is a fundamental technique that amplifies specific DNA sequences, which is essential for various diagnostics and research applications. The specificity of oligonucleotide primers ensures that the correct gene is targeted in the amplification process.
Furthermore, oligonucleotides function as probes in assays like Southern and Northern blotting. They can hybridize with desired DNA or RNA sequences, allowing for the detection and quantification of specific genetic material. Their application extends to gene silencing through small interfering RNA (siRNA) and antisense oligonucleotides, which inhibit gene expression at the mRNA level, further showcasing their diverse functionality.
Applications in Genetic Engineering
The integration of oligonucleotides in genetic engineering has yielded transformative advancements. They are employed in the process of designing and constructing recombinant DNA molecules. By utilizing oligonucleotides to facilitate precise insertions, deletions, or modifications of genes, researchers can tailor genetic constructs for specific studies or therapeutic purposes.
A notable application is CRISPR-Cas9 technology, where synthetic guide RNAs (gRNAs) play a crucial role. These gRNAs, which are a type of oligonucleotide, guide the Cas9 nuclease to exact locations on the DNA, enabling targeted gene editing with high precision. This methodology has opened new avenues in creating genetically modified organisms and addressing genetic disorders.
Oligonucleotide-Based Therapeutics
Oligonucleotide-based therapeutics represent an exciting domain in medicine. With a focus on treating various diseases, these therapeutics leverage the unique properties of oligonucleotides to modulate gene expression, thereby influencing disease pathways.
One prominent example is the use of antisense oligonucleotides in treating genetic disorders like Duchenne Muscular Dystrophy. By targeting specific mRNA transcripts, these oligonucleotides can facilitate exon skipping, allowing for the production of functional proteins.
In addition to antisense oligonucleotides, ribozymes and aptamers are being explored for their therapeutic potential, showcasing the wide-ranging impact of oligonucleotides in healthcare. As research progresses, the scope of oligonucleotide-based therapeutics continues to expand, raising hopes for innovative treatments in various medical fields.
Oligonucleotides are not just tools; they are foundational elements that redefine genetic research and therapeutic avenues.
Overall, the applications of oligonucleotides in biotechnology are substantial and continue to evolve. Their precision, versatility, and growing therapeutic potential have positioned them as essential components in contemporary biological research and medical interventions.
Experimental Techniques for Analyzing Operons and Oligonucleotides
Understanding operons and oligonucleotides requires reliable experimental techniques. These techniques can accurately assess gene expression and interactions. Researchers find these methods crucial for uncovering the complexities of genetic regulation. Their applications are both profound and numerous, influencing fields from synthetic biology to personalized medicine. This section highlights key techniques that play an important role in the research and applications relevant to these genetic components.
PCR and Its Relevance
Polymerase Chain Reaction (PCR) is a foundational technique in molecular biology. It allows for the amplification of specific DNA sequences. This ability to create millions of replicas from a small DNA sample is invaluable.
The relevance of PCR in studying operons and oligonucleotides is significant. Researchers can use it to investigate the expression levels of genes controlled by operons. PCR helps narrow down the specificity of oligonucleotides as tools for targeting genes. The technique provides precision in understanding the function of operons through quantitative data.
Sequencing Technologies
Next-generation sequencing (NGS) has transformed genetic research. This technology can sequence entire genomes or specific regions of interest rapidly and accurately. For operons, sequencing technologies allow for a comprehensive analysis of genetic elements and their regulatory sequences.
Applications of these sequencing techniques include:
- Identifying operon structures: Researchers can elucidate how genes are clustered and controlled within operons.
- Analyzing mutations: Understanding variations can provide insight into operon functionality and associated diseases.
- Oligonucleotide design: High-throughput sequencing informs the design of sophisticated oligonucleotides for gene editing and therapies.
In Situ Hybridization Methods
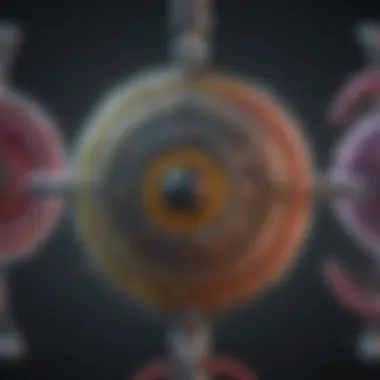
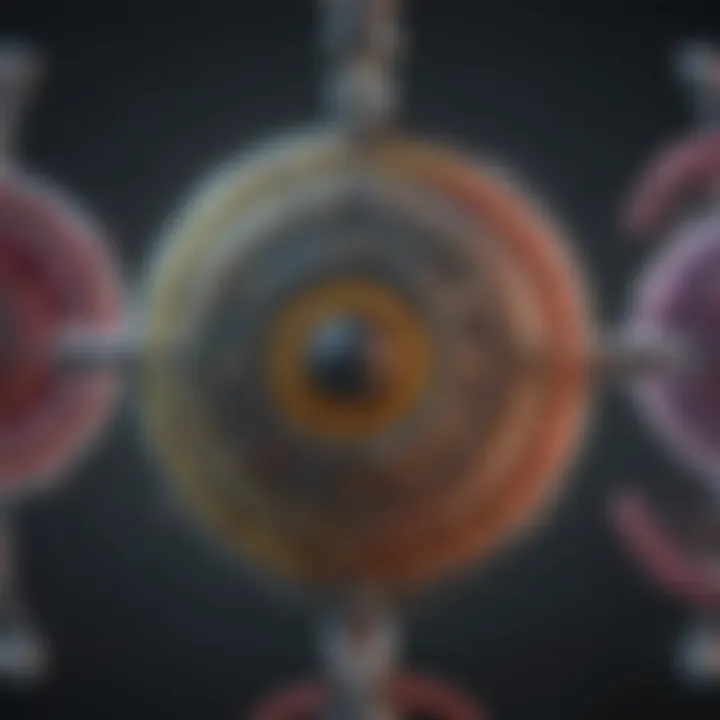
In situ hybridization (ISH) is another critical technique for analyzing operons and oligonucleotides. This method enables visualization of specific nucleic acid sequences within fixed tissues or cells. Using labeled probes, ISH provides spatial context for the expression of genes within operons.
Advantages of ISH include:
- Understanding gene localization: Researchers can investigate where and when specific genes are expressed in an organism.
- Analyzing operon interactions: ISH techniques help in exploring how different genes in an operon influence one another in various biological conditions.
- Oligonucleotide applications: It is useful in assessing the efficiency of therapeutic oligonucleotides in tissues.
These experimental techniques are essential for enhancing our understanding of operons and oligonucleotides. Their impact is felt across many areas of research and practical applications in biotechnology.
The Future of Operon and Oligonucleotide Research
The exploration of operons and oligonucleotides is ever-evolving, revealing new insights about genetic mechanisms. Understanding the future of this research domain is crucial. It can lead to advancements in biotechnology, medicine, and genetic engineering. As scientists uncover more about how operons function and how oligonucleotides can be used to manipulate them, the potential applications become limitless.
Future studies can enhance gene therapy approaches. This will address genetic disorders more effectively. Moreover, the relationship between operons and oligonucleotides can refine techniques for developing custom RNA and DNA sequences. Researchers can create more targeted experiments, improving precision in gene editing methods like CRISPR.
In this context, the benefits of ongoing research in this field are multifaceted, affecting not only scientific advancement but also societal implications concerning healthcare and agriculture.
Emerging Trends in Operon Research
There are a few emerging trends worth noting in operon research. One prominent trend is the integration of genomics and bioinformatics. Researchers now analyze vast amounts of genetic data to determine operon structure and function. This approach allows for identifying novel operons across diverse organisms.
Another trend is the increasing focus on synthetic biology. Scientists are attempting to engineer operons to create synthetic genetic circuits. These circuits can perform specific functions, offering innovative solutions to biological problems. Companies like Amgen and Genentech are leading efforts in this area, designing engineered organisms for industrial applications.
Furthermore, the use of CRISPR technology presents a notable advancement in operon research. This allows researchers to investigate gene interactions within operons more efficiently. It also enables the modification of operon sequences to observe biological outcomes, contributing to our understanding of gene regulation.
Innovative Techniques in Oligonucleotide Design
Oligonucleotide design is evolving alongside operon research. One promising technique is the use of high-throughput sequencing. This methodology allows researchers to rapidly design and test a large number of oligonucleotides. As a result, scientists are generating more effective tools for gene editing and regulation.
In addition, circular RNA (circRNA) has emerged in the oligonucleotide landscape. CircRNA can be engineered to express unique sequences that can modulate gene expression. Researchers are beginning to explore these constructs in operon studies, aiming for advancing the understanding of RNA biology.
Next-generation synthesis technologies are also reshaping the oligonucleotide design process. These advancements reduce time and cost, allowing for quick production of customized oligonucleotides. Thus, they facilitate experiments that leverage the operon's potential as a target in genetic research.
Ethical Considerations
The research into operons and oligonucleotides raises several ethical concerns. As genetic manipulation becomes more mainstream, it is vital to address potential misuse. The ability to alter gene expression in organisms poses risks if not regulated properly.
One major concern is the impact of genetically modified organisms (GMOs) on ecosystems. Researchers must consider the long-term consequences of releasing modified species into the environment. Ethical discussions involve the balance between innovation and biodiversity preservation.
Another ethical consideration includes human gene editing. While the potential to cure genetic diseases through operon manipulation is promising, it opens debates about designer genes. Misuse in this area may lead to social inequalities based on genetic enhancements.
Overall, these ethical discussions are essential as they influence regulatory frameworks. Establishing guidelines ensures research in operon and oligonucleotide domains evolves responsibly.
Epilogue
Understanding operon oligonucleotides is crucial due to their profound implications in genetic regulation and biotechnological advancements. This article highlights how operons function as units of gene expression and regulation, while oligonucleotides serve as essential tools for manipulating these processes.
Summarizing Key Insights
Recent research has uncovered several critical insights regarding operon oligonucleotides.
- Role in Gene Regulation: Operons allow for coordinated expression of functionally related genes. The presence of oligonucleotides enhances this regulation by fine-tuning gene expression.
- Biotechnological Applications: Oligonucleotides have paved the way for innovative applications in genetic engineering and therapeutics, demonstrating their versatility.
- Research Methodology: Techniques such as PCR and sequencing have become integral in studying operons and oligonucleotides. These methodologies allow for a deeper understanding of gene functions and interactions.
Overall, this complex relationship offers immense potential for further discoveries in genetics.
Implications for Future Research
The future of operon and oligonucleotide research is promising.
- Emerging Trends: Researchers should focus on developing more effective oligonucleotide designs. This could lead to enhanced gene editing technologies and more targeted therapeutic approaches.
- Ethical Considerations: With advances in biotechnology, ethical implications become increasingly important. Future studies should address these considerations to ensure responsible research practices.
- Integration of Technologies: Combining various methodologies could yield synergies, improving the understanding of genetic regulation and operon dynamics.
As this field evolves, continued investigation into the interactions between operons and oligonucleotides will unlock new avenues for innovation in genetics and biotechnology.