The Role of Mitochondria in Cellular Energy and Health
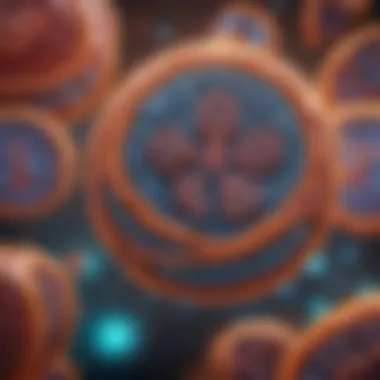
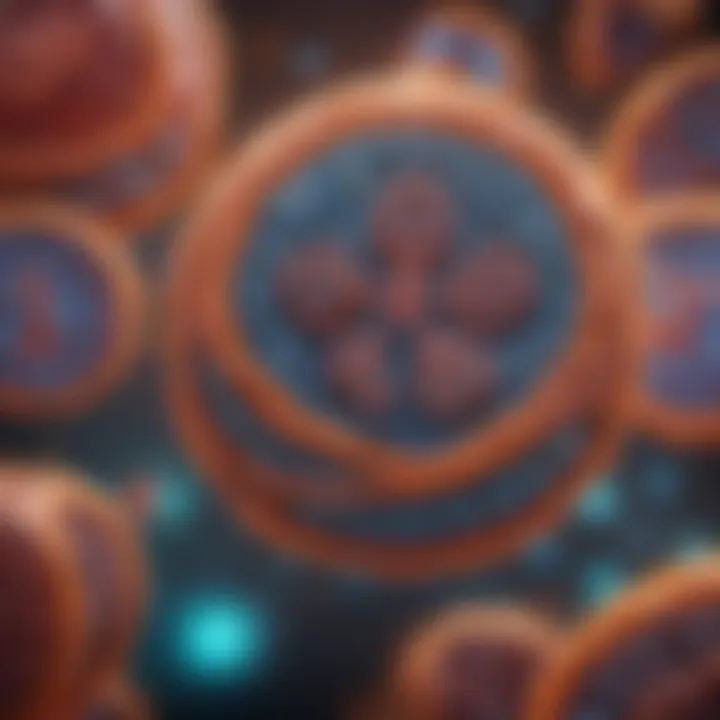
Intro
Mitochondria represent an essential component of cellular life. These organelles are often referred to as the powerhouses of the cell due to their primary role in energy production. They generate adenosine triphosphate (ATP) through a process known as oxidative phosphorylation. This process is critical for sustaining the energy needs of cells in various tissues and organs.
However, the function of mitochondria is not limited to ATP production. They are heavily involved in several metabolic processes, cellular signaling, and even programmed cell death, known as apoptosis. Understanding the various roles of mitochondria can provide significant insights into their impact on health and the potential therapeutic interventions for their dysfunction.
Mitochondrial dysfunction has been linked with numerous diseases, including metabolic disorders, neurodegenerative diseases, and the aging process itself. Therefore, gaining a deeper understanding of mitochondrial biology is vital for both researchers and healthcare professionals. The following sections will explore these themes in detail.
Foreword to Mitochondria
Mitochondria are essential organelles, often known as the powerhouses of cells. Their primary function is to produce energy in the form of adenosine triphosphate (ATP), which is vital for many cellular functions. However, their roles extend far beyond just energy production. They are involved in a variety of metabolic processes, such as the production of important molecules and the regulation of cellular signals. This multifaceted nature makes mitochondria a critical subject of study in cellular biology and medicine.
The significance of understanding mitochondria lies in their implication in various health conditions. Mitochondrial dysfunction has been linked to diseases such as diabetes, neurodegenerative disorders, and aging. As our knowledge of mitochondrial functions grows, so does the potential for developing targeted therapies aimed at mitigating these conditions. Therefore, the insights gained from studying mitochondria can have profound impacts on medical research and treatment approaches.
Defining Mitochondria
Mitochondria are double-membraned organelles found in the cytoplasm of eukaryotic cells. Their structure consists of an outer membrane and a highly folded inner membrane, known as cristae. The space between the membranes is called the intermembrane space, while the interior fluid-filled space is referred to as the matrix. Mitochondria contain their own DNA, which is distinct from the cellular DNA found in the nucleus, and enables them to replicate independently.
The principal role of mitochondria is to generate ATP through a process known as oxidative phosphorylation. This process involves the electron transport chain and the proton gradient created across the inner mitochondrial membrane. The energy captured from these reactions is used to convert adenosine diphosphate (ADP) and inorganic phosphate into ATP, the main energy currency of the cell.
Historical Perspectives
The history of mitochondrial research is quite rich and spans many decades. The term 'mitochondria' was first introduced in the 1890s. Early scientists postulated about the role and importance of these organelles, but it wasn't until the 1940s and 1950s that significant advances were made. Research unveiled that mitochondria are not only involved in energy metabolism but also play roles in various biochemical pathways.
Since then, the advancements in biochemistry and molecular biology allowed for deeper insights into mitochondrial functions. The discovery of mitochondrial DNA, for example, has led to a greater understanding of their autonomous activities. Today, studies continue to uncover their complex relationship with human health and disease, marking mitochondria as an area of great importance in both research and clinical contexts.
Mitochondria's historical journey illustrates not just a deeper understanding of cellular processes, but also highlights their relevance in health and disease over the years.
Structure of Mitochondria
The structure of mitochondria is critical to their function as energy producers. Understanding the distinct components of a mitochondrion allows researchers to comprehend how these organelles contribute to various metabolic processes. Each part of a mitochondrion serves a specific role that supports its overall efficacy in energy generation and metabolic regulation.
Outer Membrane Composition
The outer membrane of mitochondria acts as the first line of defense and interface with the cytoplasm. This membrane is composed of a lipid bilayer that contains porins, which are proteins that allow the passage of ions and small molecules. This permeability is vital for nutrient and metabolite exchange between the cytoplasm and the mitochondrion.
Commonly, the outer membrane includes phospholipids and cholesterol, which provide stability and fluidity. The presence of various proteins embedded within, such as receptors and transport proteins, enables the outer membrane to play an active role in signaling and transport processes. The outer membrane's composition directly influences mitochondrial functionality and can affect how cells respond to changes in their environment.
Inner Membrane Functionality
The inner membrane is crucial for ATP production and is highly folded into structures called cristae. These folds increase the surface area, providing more space for the electron transport chain and ATP synthase complexes. The inner membrane is selectively permeable, controlling the entry of molecules and ions, thus maintaining the specialized environment required for energy metabolism.
A significantly active transport system exists in the inner membrane, facilitating the uptake of pyruvate and fatty acids, which are essential substrates for energy production. This membrane also houses various enzymes involved in the Krebs cycle, further emphasizing its importance in cellular respiration and energy generation.
Intermembrane Space
The intermembrane space is the region between the outer and inner membranes. It plays a lesser-known but significant role in mitochondrial function. This space is crucial for establishing a proton gradient during oxidative phosphorylation, as protons are pumped from the matrix into the intermembrane space. This gradient generates potential energy that drives ATP synthesis.
Additionally, the intermembrane space is involved in redox reactions and can serve as a site for the release of cytochrome c, an important factor in apoptosis. Understanding the dynamics of the intermembrane space provides insight into how mitochondria regulate cell death and energy production.
Matrix and Its Role
The matrix is a gel-like substance found within the inner membrane. It contains mitochondrial DNA, ribosomes, and various enzymes essential for metabolic pathways. The matrix is where the Krebs cycle occurs, producing electron carriers such as NADH and FAD, which are vital for ATP production.
Furthermore, the matrix serves as a site for the synthesis of certain proteins encoded by mitochondrial DNA. This contrasts with the majority of mitochondrial proteins, which are encoded by nuclear DNA and imported post-translationally. The diverse functions of the matrix emphasize its significance in cellular respiration and metabolism, underlining the complex interplay that occurs within mitochondria.
Mitochondria not only generate energy but also regulate metabolic pathways and play a role in apoptosis, making their structural integrity vital for cell survival.
Ultimately, the structure of mitochondria is intricately designed for their multifaceted roles within the cell. Analyzing this structure is essential for understanding how mitochondria function and their impact on cellular health.
Energy Production in Mitochondria
Mitochondria serve as the core units of energy production within cells, making their functioning a pillar of cellular activity. The ability to generate energy in the form of adenosine triphosphate (ATP) allows cells to function and, by extension, sustain life. Understanding this process is essential for anyone delving into cellular biology or medicine, as it bridges the gap between energy metabolism and various physiological processes.
Adenosine Triphosphate (ATP) Generation
Adenosine triphosphate (ATP) is the primary energy carrier in all living organisms. ATP generation occurs primarily through two processes: substrate-level phosphorylation and oxidative phosphorylation. The significance of ATP cannot be overstated; it powers cellular functions ranging from muscle contraction to biochemical synthesis. In mitochondria, ATP production takes place mainly in the inner membrane where ATP synthase is located. The synthesis of ATP is intricately linked to the electron transport chain, which will be discussed further in later sections.

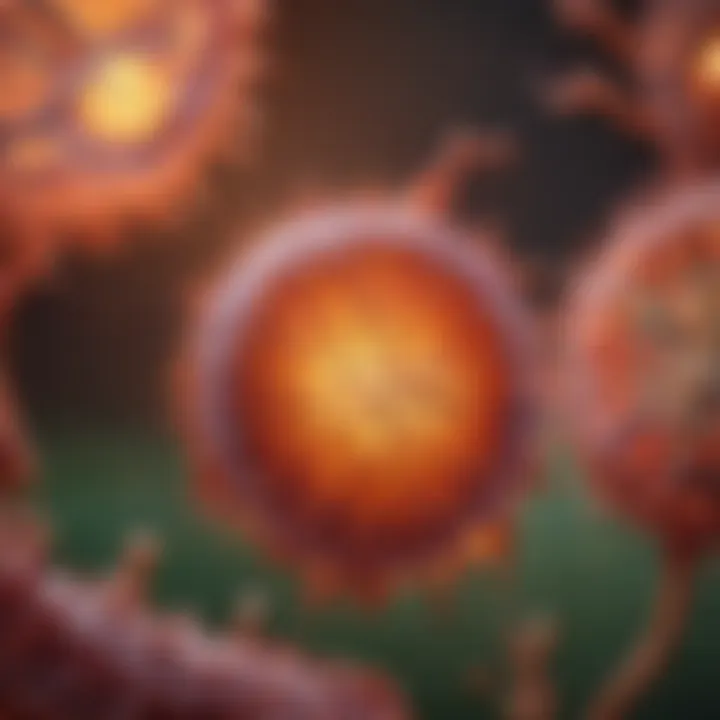
The efficient production of ATP is crucial for health. Cells depend on a steady supply of ATP to meet their needs. When energy demands increase, such as during physical activity or stress, mitochondria ramp up ATP production.
ATP is vital; without it, cellular life is unsustainable.
Oxidative Phosphorylation Mechanism
Oxidative phosphorylation refers to the metabolic pathway in which cells use enzymes to oxidize nutrients, thereby releasing energy, which is used to produce ATP. This process occurs in the inner mitochondrial membrane and involves two significant components: the electron transport chain and ATP synthase. The electron transport chain consists of several protein complexes that transfer electrons derived from nutrients. As electrons move through these complexes, protons are pumped into the intermembrane space, creating a proton gradient.
This gradient becomes a stored form of energy, often referred to as a proton motive force. ATP synthase then utilizes the energy from this gradient to convert adenosine diphosphate (ADP) and inorganic phosphate into ATP.
Ultimately, oxidative phosphorylation is efficient and responsible for approximately 90% of ATP generation in aerobic organisms. This mechanism underscores the mitochondria's role in energy metabolism, revealing how they adapt to various cellular energy demands.
Role of Electron Transport Chain
The electron transport chain (ETC) represents a series of complexes and mobile electron carriers involved in oxidative phosphorylation. It plays a critical role in transferring high-energy electrons derived from NADH and FAD, which are the reduced forms produced during the Krebs cycle.
As electrons traverse the chain, energy released is harnessed to pump protons across the inner mitochondrial membrane. This process not only contributes to creating a proton gradient but is also essential for maintaining mitochondrial membrane potential. Dysfunction in any component of the chain can lead to diminished ATP production and excess reactive oxygen species (ROS), contributing to various pathologies, including metabolic disorders and neurodegenerative diseases.
Collectively, the energy production processes within mitochondria demonstrate their pivotal role in cellular function. They not only provide ATP but also modulate critical metabolic pathways necessary for maintaining cellular health.
Metabolic Functions of Mitochondria
The metabolic functions of mitochondria extend far beyond their well-known role in energy production. These organelles are also pivotal in a variety of metabolic pathways, contributing to the biosynthesis of key molecules and the regulation of cellular metabolism. Understanding these functions is crucial as they connect energy metabolism with broader biochemical processes, highlighting the importance of mitochondria in health and disease.
Acetyl CoA Production
Acetyl CoA is a central metabolite in several biochemical processes. It serves as a key substrate in the citric acid cycle, thus impacting ATP synthesis. Mitochondria are the primary site for the generation of Acetyl CoA from various sources, including glucose, fatty acids, and certain amino acids. The conversion of pyruvate to Acetyl CoA occurs in the mitochondria and is critical for cellular respiration. This process is facilitated by the pyruvate dehydrogenase complex, which helps in the decarboxylation of pyruvate.
- Acetyl CoA is also a precursor for the synthesis of fatty acids and cholesterol. This synthesis is significant for maintaining cellular structure and function.
- Disruptions in Acetyl CoA production can lead to metabolic disorders, emphasizing the essential nature of mitochondrial health in metabolic regulation.
Fatty Acid Oxidation
Fatty acid oxidation is another critical process for energy production within mitochondria. This metabolic function involves the breakdown of fatty acids into Acetyl CoA units, providing a substantial source of energy for the cell. The process occurs via several stages, including:
- Activation of fatty acids in the cytoplasm, converting them to acyl-CoA.
- Transport of acyl-CoA into the mitochondria through the carnitine shuttle.
- Beta-oxidation, where fatty acids are systematically cleaved into Acetyl CoA.
Mitochondrial fatty acid oxidation plays a vital role in maintaining energy balance, especially during prolonged exercise or fasting. However, excessive accumulation of fatty acids can lead to mitochondrial dysfunction and metabolic diseases such as obesity and diabetes. Therefore, understanding the regulation of fatty acid oxidation in mitochondria can provide insights into preventing and managing these diseases.
Amino Acid Metabolism
Amino acids, while typically associated with protein synthesis, also undergo significant metabolic processing within mitochondria. Mitochondria are involved in the catabolism of amino acids, wherein excess amino acids are broken down to generate energy or to produce key metabolic intermediates. There are various pathways for amino acid metabolism in mitochondria, including transamination and deamination processes.
- Transamination allows amino acids to donate their amino groups to other compounds, which can then enter metabolic pathways such as the citric acid cycle.
- Deamination results in the removal of amino groups, converting amino acids into substrates like pyruvate or Acetyl CoA.
Mitochondria also play a role in the synthesis of specific amino acids, making them essential for overall protein metabolism. Disruption in amino acid metabolism within mitochondria can have far-reaching effects on cellular function, potentially leading to various metabolic disorders.
Mitochondria serve as a hub for metabolic activities, bridging energy production and metabolic pathways essential for cellular health.
Understanding the metabolic functions of mitochondria therefore provides a deeper insight into the intricate workings of cellular processes. Attention to these functions can lead to better management of health conditions associated with mitochondrial dysfunction.
Mitochondrial Dynamics
Mitochondrial dynamics refers to the processes of fission, fusion, and other alterations that regulate mitochondria's shape and function within cells. This topic is crucial in understanding how mitochondria not only produce energy but also maintain cellular health. As the cellular energy factories, how they manage their form can directly influence their efficiency and overall function. Thus, examining mitochondrial dynamics provides insights into their role in both normal physiology and disease states.
Fission and Fusion Processes
Fission and fusion are central processes that enable mitochondria to adapt to the energy demands of the cell. These processes are responsible for the continual remodeling of the mitochondrial network.
Fission is the process where a single mitochondrion divides into two. It is important for distributing mitochondria throughout the cell and for removing damaged components, thus contributing to cellular health. On the other hand, fusion allows two mitochondria to merge into one. This process is vital for mixing the contents of partially damaged mitochondria with healthier ones, which can help in compensating for potential defects and maintaining a functional mitochondrial population.
The balance between fission and fusion impacts mitochondrial integrity. Disruption in these processes can lead to mitochondrial dysfunction, contributing to several diseases, including neurodegenerative disorders and metabolic syndromes.
Mitochondrial Biogenesis
Mitochondrial biogenesis refers to the process by which new mitochondria are formed within the cell. This process is governed by various signaling pathways, particularly the PGC-1α pathway, which regulates genes essential for mitochondrial replication and function. Mitochondrial biogenesis is activated in response to cellular energy needs and environmental factors such as exercise and dietary changes.
Enhancing mitochondrial biogenesis is essential for maintaining a healthy cellular environment. Increased numbers of mitochondria can improve energy production and support overall metabolic health. In conditions like aging or metabolic diseases, impaired mitochondrial biogenesis can lead to decreased mitochondrial function and an energy deficit. Therefore, understanding this process can present potential therapeutic targets for promoting mitochondrial health.
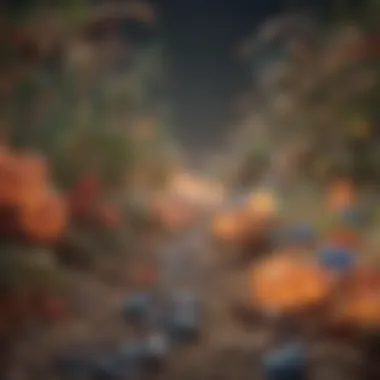
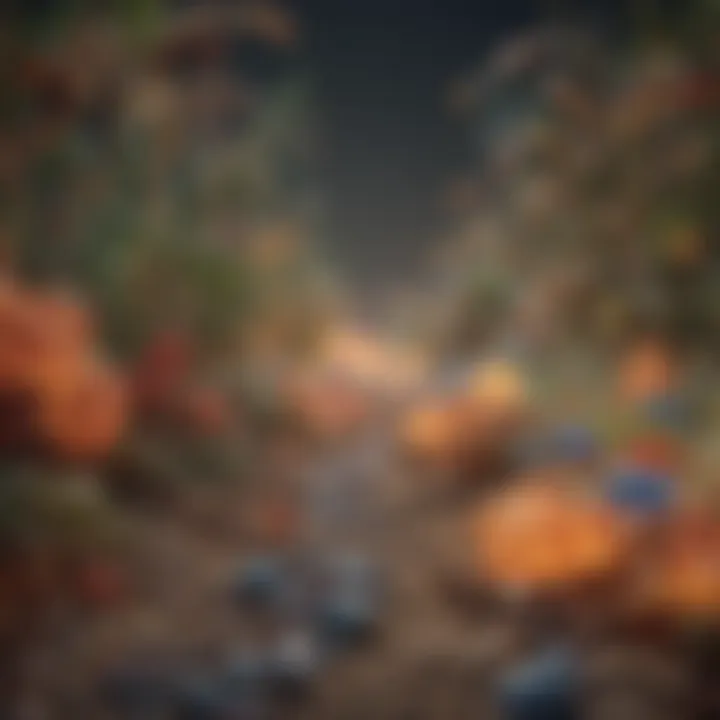
Mitophagy Mechanism
Mitophagy is the selective degradation of mitochondria by autophagy. It is a crucial cellular quality control mechanism that ensures damaged or dysfunctional mitochondria are eliminated. This is vital because accumulated defective mitochondria can lead to increased production of reactive oxygen species, which contribute to cellular stress and damage.
The regulation of mitophagy is particularly important in maintaining mitochondrial function during stress conditions. When energy demand is low or when mitochondria are unhealthy, mitophagy targets them for degradation, facilitating cellular rejuvenation.
In recent years, research has indicated that enhancing mitophagy could be a therapeutic strategy for diseases involving mitochondrial dysfunction, such as Parkinson's disease and cancer. By understanding how mitophagy works, researchers can develop strategies to promote this process for improving cellular health and preventing disease.
"Mitochondrial dynamics are integral to cellular health, impacting not just energy production but overall cell function."
In summary, mitochondrial dynamics play a pivotal role in the functionality and longevity of mitochondria. They are key factors that facilitate energy adaptability and cellular responses to stress. Understanding these dynamics offers significant opportunities for therapeutic advancements in treating various diseases related to mitochondrial dysfunction.
Mitochondrial Signaling Pathways
Mitochondrial signaling pathways play a crucial role in maintaining cellular homeostasis and regulating various cellular processes. Understanding these pathways is essential for comprehending how mitochondria influence cellular function beyond just energy production. Several key elements define mitochondrial signaling pathways, including calcium homeostasis, regulation of cellular metabolism, and apoptosis induction. Each of these components contributes to the intricate functioning of cells, impacting overall health and disease.
Calcium Homeostasis
Calcium ions function as secondary messengers in many signaling pathways. Mitochondria significantly contribute to calcium homeostasis by acting as storage and release sites for calcium ions. When cells require calcium, mitochondria release it into the cytosol, aiding in various cellular processes such as muscle contraction and neurotransmitter release. Conversely, when calcium levels need to be reduced, mitochondria can uptake excess calcium, preventing cellular toxicity.
This calcium regulation is not only vital for immediate cellular responses but also influences longer-term signaling pathways. For instance, mitochondrial calcium uptake triggers various metabolic responses, leading to enhanced ATP production when energy demand is high. Disruptions in this homeostasis can lead to a variety of diseases, including neurodegenerative conditions like Alzheimer’s Disease. These conditions underscore the importance of calcium regulation by mitochondria for maintaining cellular health.
Regulation of Cellular Metabolism
Mitochondria serve a central role in cellular metabolism, coordinating the use of energy substrates in response to cellular needs. They do this through complex signaling mechanisms that integrate external and internal signals to modulate how cells utilize nutrients. Mitochondria respond to changes in energy status by adjusting metabolic pathways, such as switching between oxidative phosphorylation and glycolysis based on nutrient availability.
The signaling pathways involved in this metabolic regulation are tightly interconnected with various cellular conditions. For example, during times of low energy supply, certain mitochondrial signaling molecules can activate pathways that promote fat metabolism over carbohydrate metabolism. This adaptability allows cells to optimize their energy use. However, when these regulatory mechanisms fail or become impaired, it can lead to metabolic disorders such as obesity and diabetes. Thus, proper mitochondrial metabolic regulation is essential for maintaining energy balance in cells.
Apoptosis Induction
Apoptosis, or programmed cell death, is a critical process for maintaining cellular integrity and homeostasis. Mitochondria play a central role in initiating apoptosis through various pathways. When cellular stress occurs, mitochondria release cytochrome c, which activates caspases, the enzymes responsible for carrying out apoptosis. This release is often influenced by mitochondrial membrane potential and the overall health of the organelle.
The signaling pathways leading to apoptosis are essential for preventing the growth of unhealthy cells. For example, in cancer, the failure to initiate apoptosis allows for unchecked cell division. Additionally, mitochondrial dysfunctions can lead to unintended cell death, contributing to various diseases. Understanding how mitochondria signal for apoptosis can open pathways for therapeutic interventions aimed at regulating cell death in conditions like cancer and neurodegenerative diseases.
In summary, mitochondrial signaling pathways are essential for regulating calcium levels, cellular metabolism, and apoptosis. Their ability to modulate these processes has far-reaching implications for health and disease.
Understanding these pathways allows researchers to explore new therapeutic strategies to address mitochondrial dysfunctions that contribute to various illnesses, illuminating the critical role of mitochondria in cellular biology.
Mitochondrial Genetics
Mitochondrial genetics is an essential aspect of understanding how our cells function and maintain energy balance. Unlike the majority of our genetic material, which is housed within the nucleus, mitochondria possess their own distinct DNA. This unique structure plays a significant role in various cellular processes. Studying mitochondrial genetics offers insights into hereditary diseases, metabolic disorders, and potential therapeutic strategies to address mitochondria-related dysfunctions.
Mitochondrial DNA Structure
Mitochondrial DNA (mtDNA) is circular and consists of approximately 16,500 base pairs. This small genome encodes critical genes necessary for mitochondrial functions, especially those related to energy production. The genes found in mtDNA are primarily involved in the electron transport chain and ATP synthesis, which are vital for cellular energy metabolism.
One notable feature of mtDNA is its proximity to the respiratory chain complexes, as they work together to generate ATP efficiently. Unlike nuclear DNA, mtDNA is inherited only from the mother, which makes it invaluable for studies related to maternal lineage and evolutionary biology.
Hereditary Patterns
The inheritance of mitochondria occurs through maternal transmission. Every offspring receives their mitochondria from the egg cell, while sperm typically contribute little to no mitochondria to the embryo. This unique inheritance pattern results in certain diseases being passed directly from mothers to their children.
Mitochondrial disorders can arise from mutations in mtDNA, affecting energy production. For example, conditions such as Leber’s hereditary optic neuropathy and mitochondrial myopathy highlight the implications of mtDNA mutations. Understanding these patterns is crucial for genetic counseling and risk assessment in families with a history of mitochondrial diseases.
Mutations and Their Impacts
Mutations in mitochondrial DNA can occur due to various factors, including environmental influences and normal cellular processes. These mutations can have varying effects, potentially leading to diseases that affect multiple systems in the body. Some mutations may be benign, while others can lead to significant dysfunction in energy metabolism.
Mitochondrial mutations often result in conditions characterized by fatigue, weakness, and impaired cellular function. The severity of symptoms can depend on the proportion of mutated mtDNA present in cells.
In addition, as we age, the accumulation of mutations can contribute to mitochondrial dysfunction, playing a role in age-related diseases. Research into these mutations can elucidate pathways for developing therapies aimed at restoring normal mitochondrial function, thus highlighting the importance of mitochondrial genetics in health and disease.
Mitochondria in Health and Disease
Mitochondria are more than just cellular power plants; they are crucial players in a wide range of health conditions and diseases. Understanding their role in health and disease is essential for unraveling complex biochemical networks that govern our bodies. The implications span from metabolic disorders to neurodegenerative diseases, and even the aging process itself. This section highlights the multifaceted roles of mitochondria in these areas, emphasizing their significance for both cellular health and clinical interventions.
Role in Metabolic Disorders
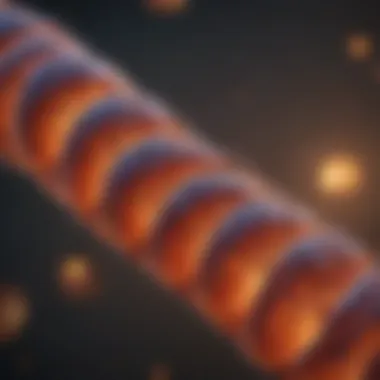
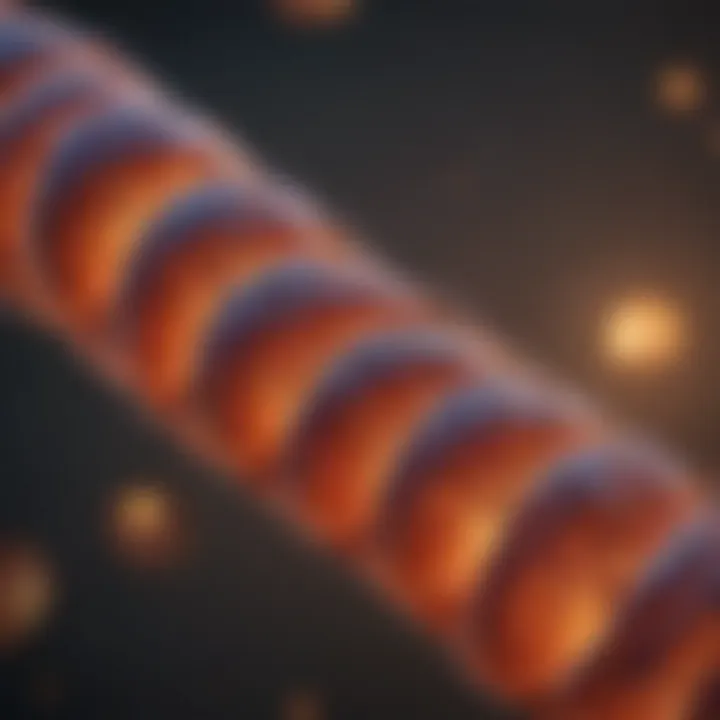
Mitochondria are central to cellular metabolism. They manage energy production and contribute to numerous metabolic pathways. In metabolic disorders such as obesity and diabetes, mitochondrial function is often compromised. Defective mitochondria can lead to reduced energy production and increased fat accumulation. This dysfunction can trigger insulin resistance, which is a key feature of Type 2 diabetes.
Research shows that mitochondria can influence metabolic flexibility. When these organelles are functioning optimally, the body can switch between using carbohydrates and fats for energy efficiently. Conversely, impaired mitochondrial function results in metabolic inflexibility, creating a cycle that exacerbates disease processes.
Additionally, studies suggest therapies targeting mitochondrial dysfunction may improve metabolic health. Interventions such as exercise facilitate mitochondrial biogenesis, which may restore normal metabolic functions. As a result, maintaining mitochondrial health is crucial in managing metabolic disorders.
Implications for Neurodegenerative Diseases
Mitochondria are implicated in several neurodegenerative disorders, including Alzheimer's disease and Parkinson's disease. These diseases often feature mitochondrial dysfunction, which leads to energy deficits in neurons. Neurons require a significant amount of energy to maintain their function. When mitochondria fail, it contributes to neuronal death and cognitive decline.
Mitochondrial dysfunction may precede and exacerbate the pathophysiological features of neurodegenerative diseases.
In Alzheimer's disease, for example, decreased ATP production correlates with tau protein accumulation and amyloid-beta plaques. Mounting evidence pinpoints the need for therapeutic strategies aimed at enhancing mitochondrial function to slow down neurodegeneration. Targeting mitochondrial pathways may help mitigate symptoms or even prevent the onset of these debilitating diseases.
Mitochondrial Dysfunction and Aging
Aging is closely linked to the decline in mitochondrial function. Over time, mitochondrial DNA can accumulate mutations, impacting energy production. This leads to insufficient ATP levels, contributing to cellular aging and senescence. Furthermore, dysfunctional mitochondria are a source of reactive oxygen species, which can cause oxidative stress and cellular damage.
The connection between aging and mitochondrial health has spurred interest in interventions that promote mitochondrial resilience. Caloric restriction and regular physical activity are two lifestyle changes that have been shown to improve mitochondrial function and reduce markers of aging.
In summary, studies emphasize the centrality of mitochondria in health and disease. From their involvement in metabolic control to their crucial role in neurodegenerative conditions and the aging process, mitochondria remain a focal point of research and therapeutic intervention. Understanding these complexities is vital for both basic science and potential clinical applications.
Therapeutic Strategies Targeting Mitochondria
The exploration of therapeutic strategies aimed at mitochondria is crucial in understanding and potentially treating various diseases linked to mitochondrial dysfunction. These strategies delve into the manipulation and enhancement of mitochondrial activities, aligning closely with advancements in biomedical sciences. By identifying methods to restore or improve mitochondrial function, researchers hope to address the root causes of several debilitating conditions.
Various elements are at play when discussing therapeutic strategies targeting mitochondria. These encompass Mitochondrial Replacement Therapy, the use of Antioxidants, and innovations in Mitochondrial Medicine. Each of these approaches presents unique benefits and considerations surrounding mitochondrial health and disease treatment.
Mitochondrial Replacement Therapy
Mitochondrial Replacement Therapy is an innovative approach that aims to replace defective mitochondria with healthy ones. This technique primarily targets conditions arising from mitochondrial DNA mutations, which often lead to severe metabolic disorders. By employing methods such as pronuclear transfer or maternal spindle transfer, it has been shown that it is possible to prevent the transmission of these detrimental mutations from mother to child.
The benefits are considerable. Healthy mitochondria can significantly improve cellular function in affected individuals. However, ethical considerations and regulatory challenges remain paramount in the discourse of this type of therapy. The potential for unintended consequences or the modification of human germline raises complex discussions in bioethics. As we move forward, establishing stringent guidelines will be essential to navigate these issues wisely.
Antioxidants and Mitochondrial Health
Antioxidants are critical in preserving mitochondrial health. Reactive oxygen species (ROS), which are naturally produced during ATP generation, can cause oxidative stress if not regulated effectively. This imbalance can lead to cellular damage and contribute to various disorders, including neurodegenerative diseases.
Utilizing antioxidants such as Coenzyme Q10, Alpha-lipoic acid, and vitamins C and E can enhance mitochondrial function. These compounds scavenge free radicals, reducing oxidative stress and thereby protecting mitochondrial integrity. It is worth noting that while antioxidants can be beneficial, the dosage and timing must be monitored to avoid any adverse effects, making personalized approaches vital in this area.
Future Directions in Mitochondrial Medicine
The field of mitochondrial medicine is advancing rapidly, and future directions show promise for enhancing treatment outcomes. Ongoing research is focused on a few key areas. First, developing drugs that specifically target mitochondrial function is becoming a major focus. This includes compounds that can enhance ATP production or improve the efficiency of the electron transport chain.
Second, there is a growing interest in gene therapies aimed at correcting mitochondrial genetic defects. Techniques such as CRISPR/Cas9 could potentially edit mitochondrial DNA, offering hope for conditions previously deemed untreatable.
Finally, understanding the interplay between mitochondria and overall cellular health will further develop therapeutic strategies. As researchers delve deeper into metabolic pathways and signaling mechanisms, new opportunities for targeted therapies emerge.
"Mitochondrial medicine is set to revolutionize the way we approach diseases and treatments. It combines cutting-edge science with practical applications in health care."
Culmination
The role of mitochondria in cellular function is multifaceted and essential for the maintenance of life. Understanding their various functions offers insight into how our bodies operate at a cellular level. Mitochondria are far more than just energy producers; they are vital for metabolism, signaling pathways, and programmed cell death.
Summary of Mitochondrial Functions
Mitochondria primarily generate adenosine triphosphate (ATP) through the process of oxidative phosphorylation. This function is critical as ATP serves as the primary energy currency in cells. Beyond energy production, mitochondria are involved in:
- Metabolic processes: Contributing to fat oxidation and amino acid breakdown.
- Calcium homeostasis: Regulating calcium levels within cells is crucial for signaling.
- Cellular signaling: Participating in pathways that govern cell growth and death.
- Apoptosis: Mitochondria are key players in apoptosis, impacting how cells respond to stress and damage.
These functions interconnect to uphold cellular health, influencing overall organismal health.
Implications for Future Research
As we move forward, the exploration of mitochondria offers promising avenues for research. The implications are significant in several areas:
- Diseases: Mitochondrial dysfunction is linked to a host of diseases, including diabetes, neurodegenerative disorders, and some cancers. Targeting mitochondria may provide therapeutic pathways.
- Aging: Understanding the role of mitochondria in aging could lead to interventions that promote longevity or improve quality of life in older adults.
- Biotechnology: Advancements in biotechnology could harness mitochondrial functions for bioengineering applications.
As researchers continue to unveil the complexities of mitochondrial functions, the need for effective therapies for mitochondrial-related diseases grows increasingly critical. Further studies could lead to innovative treatments that leverage mitochondrial biology to enhance human health.
"Understanding mitochondria is no longer just an academic pursuit; it holds the key to unlocking new therapies that address some of humanity’s most pressing health issues."
In essence, the investigation and ongoing research into mitochondrial biology not only enrich our understanding of cellular processes but may also catalyze significant breakthroughs in medicine, enhancing patient outcomes and addressing chronic health concerns.