Understanding Gamma Ray Shielding: Key Concepts and Uses
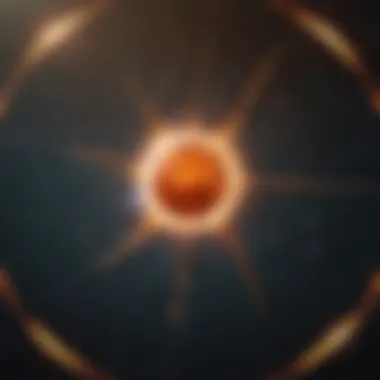
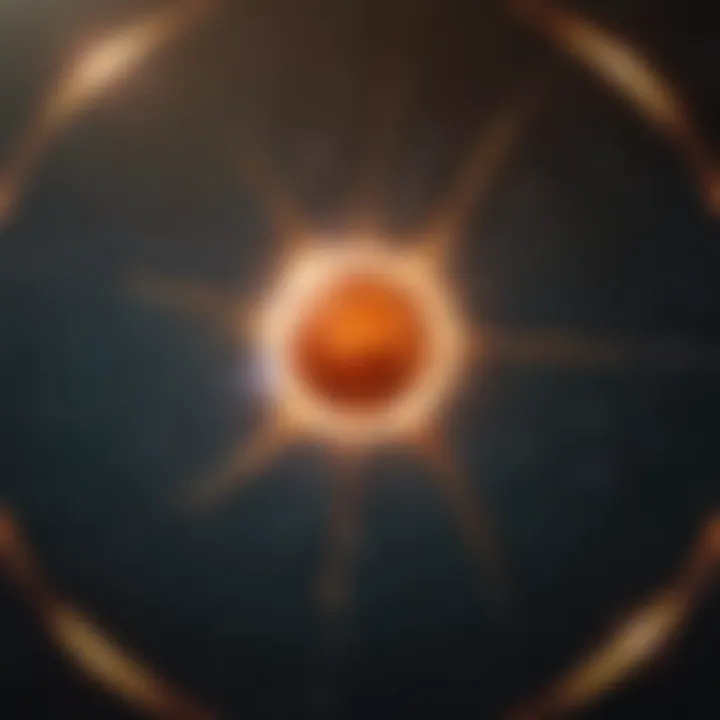
Intro
Gamma rays, a form of high-energy electromagnetic radiation, pose significant concerns in various fields, particularly in radiation safety, nuclear science, and healthcare. Understanding how gamma rays interact with matter is crucial for developing effective shielding techniques. From construction materials to advanced technology, the way we can mitigate exposure to gamma radiation is essential not only for safety but also for efficient operation in environments where gamma rays are prevalent. This comprehensive guide aims to illuminate the multifaceted nature of gamma ray shielding, exploring its core principles, the materials involved, and the wide-ranging applications that underscore its relevance.
Research Highlights
Key Findings
The investigation into gamma ray shielding presents several critical insights:
- Material Selection: The effectiveness of different materials in attenuating gamma radiation varies widely. Heavy metals such as lead and tungsten are often chosen for their density and atomic number, which greatly influence their shielding capabilities.
- Thickness Matters: The thickness of the shielding material correlates directly with the level of protection. Calculations based on half-value layers help define how much material is necessary to significantly reduce gamma radiation exposure.
- Innovative Approaches: Recent advancements in shielding technologies indicate a shift toward lighter, composite materials that can provide similar levels of protection while reducing structural burden.
Implications and Applications
The implications of gamma ray shielding extend across industries:
- Healthcare: In medical settings, where diagnostic imaging and cancer treatment using radiation are commonplace, effective shielding protects both patients and medical staff from unnecessary exposure.
- Nuclear Industry: In power plants and waste management facilities, proper shielding is crucial for safeguarding personnel and the surrounding environment.
- Research Facilities: Laboratories conducting experiments with radioactive materials must implement shielding structures to maintain operational safety.
Methodology Overview
Research Design
The research design revolves around empirical studies, combining theoretical calculations with practical experiments. Field data gather insights into real-world applications of shielding materials, allowing for a broader understanding of how gamma rays interact under various conditions.
Experimental Procedures
Experimental procedures involve assessing the effectiveness of various materials through controlled tests. These tests typically include:
- Gamma Radiation Source Utilization: Use of calibrated gamma sources, ensuring consistent exposure levels.
- Measurement Techniques: Utilizing dosimeters to measure radiation levels before and after passing through shielding materials.
- Data Analysis: Developing models based on the collected data to predict performance under different scenarios.
"The future of gamma ray shielding lies in balancing efficiency, effectiveness, and material sustainability."
By carefully investigating these dimensions, research continues to refine our approach to managing gamma radiation hazards.
Prelude to Gamma Rays
Gamma rays are a form of electromagnetic radiation with high energy, penetrating power, and a significant role in both natural and engineered environments. Understanding gamma rays is essential for professionals and researchers in diverse fields like nuclear science, radiation safety, and healthcare. It provides a foundation for grasping how gamma rays interact with matter and the rational behind protective measures.
Nature of Gamma Radiation
Gamma radiation is characterized by its high energy photons, generated during the decay of radioactive materials. Unlike alpha and beta particles, gamma rays do not have mass or charge, allowing them to travel immense distances without being significantly impacted by matter. This feature contributes to their utility and hazards alike. In space, astronomical sources like neutron stars and supernovae emit gamma rays, showcasing their cosmic significance, while on Earth, they primarily originate from radioactive isotopes like uranium and radon. Essentially, gamma rays embody the dual nature of science, serving both beneficial and harmful purposes depending on the context and exposure levels.
Sources of Gamma Rays
Gamma rays can spring from various sources, both natural and artificial. Understanding these sources is critical for effective shielding and safety protocols. Here are some notable examples:
- Natural Sources: Cosmic events such as supernova explosions and gamma-ray bursts in space are potent sources. On a terrestrial level, isotopes like uranium, thorium, and potassium-40 are found in rocks and soil, contributing to background radiation.
- Artificial Sources: In medicine, gamma rays are used in diagnostic imaging and cancer treatment via external beam radiation therapy. Additionally, nuclear reactors and radioactive waste from various industrial processes buck up artificial exposure to gamma radation.
Here’s the gist: From astrophysics to medical applications, the sources of gamma rays vary widely, each necessitating particular approaches to manage their interaction with biological and environmental systems.
"Gamma rays are not just a concern for nuclear scientists; they are a fundamental part of medical technology and industrial processes, illustrating the need for thorough understanding and appropriate protective measures."
As this exploration unfolds, readers will appreciate how crucial it is to delve into the principles governing gamma rays, as well as the materials employed for shielding against their potentially harmful effects.
The Science of Shielding
Gamma ray shielding is not just a technical requirement; it represents a critical line of defense against high-energy radiation. Shielding is all about keeping radiation at bay to protect people, equipment, and the environment. When radiation interacts with matter, complex processes unfold, which are essential to grasp if we want to design effective shielding systems. Understanding this science helps us select the right materials and methods tailored to specific situations, whether in medical, industrial, or research settings.
Interaction of Gamma Rays with Matter
Gamma rays interact with matter in three primary ways, and each plays a significant role in how we can shield against them. These interactions are pivotal to determining the effectiveness of any shielding material.
Photoelectric Effect
The photoelectric effect occurs when a gamma photon strikes an atom, transferring its energy to an electron, which gets ejected from the atom. This process is most significant at lower photon energies.
- Key Characteristics: One of the standout features of the photoelectric effect is its dependency on the atomic number of the material. Higher atomic numbers result in a higher probability of absorption.
- Benefits: This is particularly favorable for medical applications where high atomic number materials, like lead, can provide substantial attenuation of low-energy gamma rays.
- Disadvantages: However, as photon energy increases, the likelihood of this effect decreases, making it less effective against high-energy gamma radiation.
Compton Scattering
Compton scattering denotes a scenario in which a gamma photon collides with a loosely bound electron. This interaction leads to a partial transfer of energy and a change in direction of the photon.
- Key Characteristics: Unlike the photoelectric effect, Compton scattering is more relevant at mid energies, around a few hundred keV.
- Benefits: It allows for significant scattering of gamma rays, which can help spread radiation more widely. Thus, materials that rely on this effect can be deemed versatile.
- Disadvantages: However, the scattering can lead to a secondary radiation problem as the scattered rays still possess sufficient energy to be harmful.
Pair Production
Pair production is an interaction that occurs when gamma rays have energy exceeding 1.022 MeV, converting a photon into an electron-positron pair.
- Key Characteristics: This is distinct because it requires a high energy threshold, meaning it only comes into play with very high-energy gamma rays.
- Benefits: It effectively neutralizes high-energy photons, making it indispensable in nuclear facilities dealing with such energy levels.
- Disadvantages: The threshold energy requirement means that for many applications—especially those with lower energy emissions—this process may not contribute significantly to shielding effectiveness.
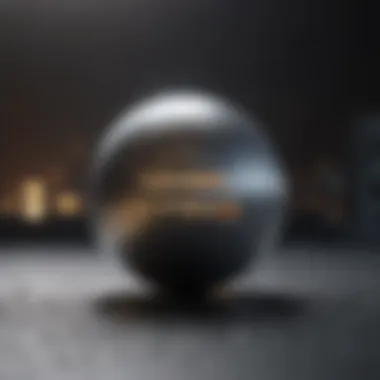
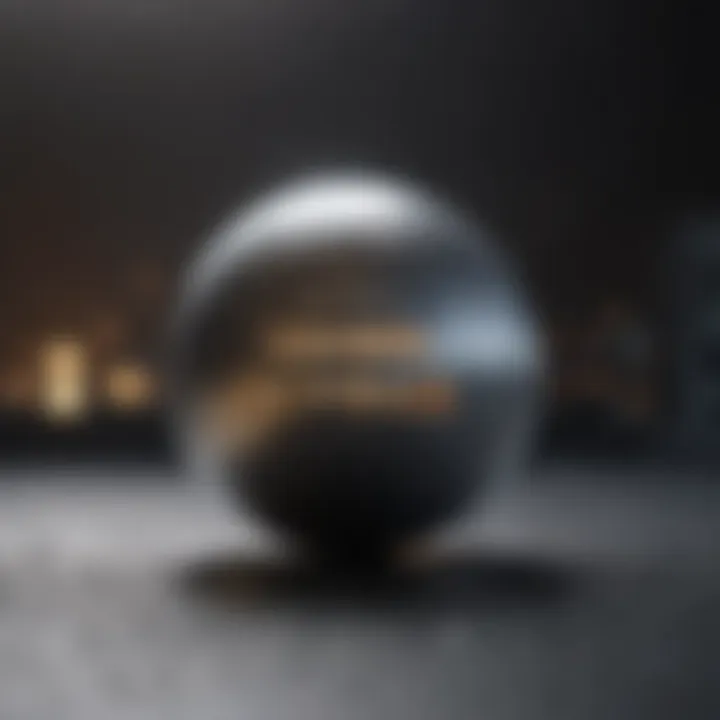
Physical Principles of Shielding
The understanding of physical principles is crucial for effective gamma ray shielding. These principles allow us to quantify how materials attenuate radiation and provide foundational knowledge for engineering solutions.
Attenuation Coefficient
The attenuation coefficient defines how much radiation is absorbed or scattered per unit distance in a material.
- Key Characteristics: It varies with photon energy and the material used. High attenuation coefficients indicate effective shielding capabilities.
- Benefits: Knowing this coefficient is invaluable for engineers as it helps in estimating required material thickness for desired protection levels.
- Disadvantages: Yet, it does not account for the shape or configuration of the shielding material, which may influence overall effectiveness.
Half-Value Layer
Half-value layer (HVL) denotes the thickness of material needed to reduce gamma radiation intensity by half.
- Key Characteristics: This is a practical measure that simplifies calculations for shielding effectiveness.
- Benefits: The HVL approach is user-friendly, providing a straightforward benchmark for designing shielding systems.
- Disadvantages: However, it assumes uniform material and does not address varying environmental conditions that could affect performance.
Mass Energy Absorption Coefficient
The mass energy absorption coefficient measures the energy absorbed per unit mass of the material.
- Key Characteristics: This coefficient considers the energy carried away by the secondary electrons produced when gamma photons are absorbed.
- Benefits: It gives a more comprehensive picture of how much energy is truly absorbed, going beyond mere attenuation. This measure is helpful in nuclear medicine contexts where dose calculations are critical.
- Disadvantages: The complexity of this coefficient can be a barrier for some practitioners unfamiliar with advanced radiation physics.
Understanding these interactions and principles allows for a nuanced approach to gamma ray shielding. While the basics provide a solid foundation, various materials and their properties can be leveraged for enhanced radiation safety across multiple domains.
Shielding Materials
In the realm of gamma ray shielding, the importance of shielding materials cannot be understated. These materials act as barriers that absorb or deflect radiation, thereby reducing exposure and protecting both humans and sensitive equipment. The choice of material significantly influences the safety measures implemented in various applications ranging from medical imaging to nuclear energy. Each material has its strengths, weaknesses, and specific use cases, which makes understanding these factors crucial in ensuring effective shielding solutions.
Types of Shielding Materials
Lead
Lead is perhaps the most famous shielding material used in radiation protection. Its density, combined with its atomic number, allows it to effectively attenuate gamma rays. This high density means that lead can be used in thinner sheets, providing significant shielding without the bulk associated with other materials. Notably, lead is very popular in x-ray rooms and labs where radiation exposure is a concern. However, the disadvantage lies in its toxicity and environmental impact, necessitating careful disposal and handling processes.
- Key characteristic: High density
- Unique feature: Thin sheets provide significant protection
- Advantages: Effective and ease of use
- Disadvantages: Toxicity and disposal issues
Concrete
Concrete is a widely used shielding material, especially in large structures such as nuclear power plants. Its primary advantage comes from its availability and cost-effectiveness. While not as dense as lead, when used in sufficient thickness, concrete can achieve comparable attenuation. The unique aspect of concrete is its ability to be molded into various forms, allowing for versatile applications in construction. On the downside, the bulkiness and weight of concrete can be a drawback in scenarios where space is limited.
- Key characteristic: Easy to source and inexpensive
- Unique feature: Versatility in design
- Advantages: Cost-effective solution
- Disadvantages: Bulkiness and weight limitations
Water
Water serves as an interesting choice for gamma shielding, particularly in nuclear reactors. Its unique characteristic is the high hydrogen content, which plays a vital role in slowing down neutrons and absorbing gamma rays. The advantage of using water lies in its abundance and safety—it poses no toxicity or long-term disposal issues. However, the effectiveness of water as a shielding material diminishes as the gamma ray energy levels increase, requiring careful consideration of the application.
- Key characteristic: Abundant and safe
- Unique feature: High hydrogen content
- Advantages: Non-toxic and readily available
- Disadvantages: Less effective at high energy levels
Polyethylene
Polyethylene is gaining traction as a shielding material due to its lightweight nature and flexibility. Its hydrogen atoms contribute to effective gamma ray reduction, and it is often used in combination with other materials for enhanced protection. Being cheap and easily manufactured, polyethylene offers a practical approach to shielding without significant weight penalty. Its primary drawback can be strength; when exposed to intense radiation over a longer period, these materials may degrade faster than their denser counterparts.
- Key characteristic: Lightweight and flexible
- Unique feature: Effective hydrogen content
- Advantages: Low cost and ease of manufacture
- Disadvantages: Potential for faster degradation
Selecting Appropriate Materials
Effective gamma ray shielding is not just about picking any material but involves considering various factors to ensure optimal protection. Factors such as density, thickness, cost, and the specific nature of the application must guide decisions in material selection.
Density Considerations
Density considerations are paramount when selecting shielding materials. Heavier materials, such as lead and concrete, tend to provide superior attenuation. The advantage of high density lies in the capability to shield effectively without requiring excessive thickness. However, it's crucial to balance density with other factors like cost and space, as high-density materials can be more expensive.
- Key characteristic: Greater density leads to better attenuation
- Unique feature: Cost and space needs must also be considered
- Advantages: Allows for effective shielding in compact designs
- Disadvantages: Can be more expensive and difficult to handle
Thickness and Geometry
The thickness of the shielding material critically influences the level of protection achieved. A thicker barrier will shield more effectively from radiation. In addition to thickness, the geometry of placement is key; how a material is arranged can lead to better dispersion of rays, enhancing shielding efficiency. However, as thickness increases, so do the costs and handling issues, which must be strategically managed.
- Key characteristic: Direct relationship between thickness and protection level
- Unique feature: Geometry also affects shielding effectiveness
- Advantages: Potential for reduced radiation exposure
- Disadvantages: Increased costs and logistics
Cost-Effectiveness
Cost-effectiveness is an essential aspect in selecting shielding materials. It involves the balance between the initial investment in shielding materials and their long-term operational safety benefits. Finding a cost-effective solution without compromising safety can be a challenging task. Factors such as installation costs, maintenance, and potential future expenses related to radiation exposure must all be considered in the decision-making process.
- Key characteristic: Balance initial cost with long-term benefits
- Unique feature: Potential ongoing expenses should also be accounted
- Advantages: Ensures financial viability of shielding solutions
- Disadvantages: Overlooking initial costs could lead to future safety issues
Effective shielding is crucial in many fields, especially in the healthcare sector and nuclear energy. Choosing the right materials ensures not only safety but also enhances the productivity of operations where radiation is handled.
Understanding these materials equips practitioners with the knowledge they need to implement comprehensive safety measures.
Mathematical Models for Shielding Evaluation
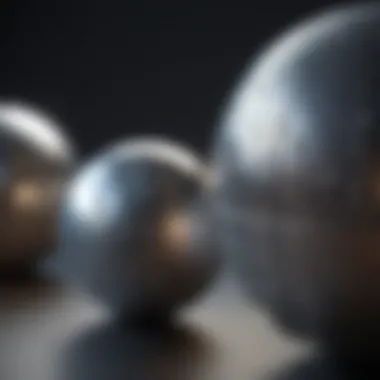
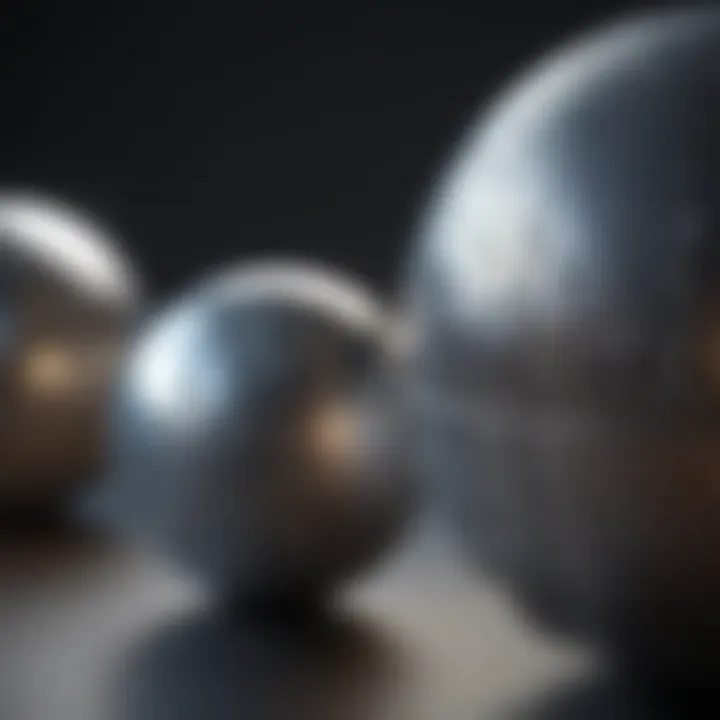
Mathematical models serve as the backbone for evaluating the effectiveness of gamma ray shielding. They provide a framework to predict how various materials will interact with gamma radiation. In essence, these models allow scientists and engineers to make informed decisions regarding shielding configurations, ultimately ensuring safety in medical, nuclear, and industrial settings. By accurately calculating the attenuation of gamma rays, stakeholders can optimize designs and materials, potentially saving resources while maintaining safety standards.
The evaluation process is informed by specific equations and computational methods that can accurately portray complex interactions between gamma radiation and shielding materials. These models not only help in understanding the fundamental physics behind shielding but also assist in practical applications, making them indispensable in the discussion of gamma ray protection.
Basic Equations and Formulas
Exponential Attenuation Law
The Exponential Attenuation Law is a core concept in the evaluation of gamma ray shielding. This principle states that as gamma rays pass through a material, their intensity decreases exponentially relative to the thickness of the material. Mathematically expressed as:
[ I = I_0 e^- au x ]
Where (I) is the transmitted intensity, (I_0) is the initial intensity, (\tau) is the attenuation coefficient, and (x) is the thickness of the material. This relationship is pivotal because it allows for straightforward calculations of how much gamma radiation can penetrate a given shield.
The main strength of this law lies in its simplicity and predictability. This makes it a popular choice in various applications ranging from radiation safety assessments to design specifications. One unique feature is its ability to provide quick estimates, which is hugely beneficial for preliminary studies or evaluations.
However, there are disadvantages as well. The Exponential Attenuation Law assumes homogeneous materials and does not account for fluctuations in density or composition, which may affect the effective shielding capability. Therefore, while it offers a good starting point, real-world applications may demand adjustments.
Transmission and Dose Calculations
Transmission and Dose Calculations are essential for determining the radiation exposure and the effectiveness of shielding materials. These calculations build on the Exponential Attenuation Law by integrating factors like source strength and exposure time. The core equation can be simplified:
[ Dose = \fracAd^2 \times T \times \Delta t ]
Where (A) is the activity of the radioactive source, (d) is the distance from the source, (T) is the transmission factor through the shielding material, and (\Delta t) is the time of exposure. This formula is crucial for assessing the actual dose received by individuals working in or around radiation sources.
The key characteristic of these calculations is their specificity. They cater to different scenarios, whether it's estimating exposure in a medical radiology room or evaluating safety in a nuclear facility. While they provide a clear picture of radiation risks, they can also be complex and require accurate data inputs, making them less accessible for quick assessments.
Advanced Computational Methods
Monte Carlo Simulations
Monte Carlo Simulations are sophisticated mathematical techniques that use random sampling to model complex systems, including gamma ray interactions with materials. They are particularly useful in gamma ray shielding evaluations where multiple scattering events and material inhomogeneities come into play.
One of the standout aspects of Monte Carlo Simulations is their incredible flexibility. They can incorporate various parameters, such as energy dependence, geometry of the shield, and material composition. This comprehensive simulation offers insight into how gamma rays scatter and absorb, providing more detailed results than traditional analytical methods can. Despite their power, they often require substantial computational resources and a deep understanding to interpret the results effectively.
Finite Element Analysis
Finite Element Analysis (FEA) is another advanced method used to assess the effectiveness of gamma ray shielding. FEA divides complex structures into smaller, manageable elements, allowing for computing performance under various conditions. This method is especially relevant in scenarios involving geometrically intricate shields or those made of composite materials.
The primary advantage of FEA is its precision in handling multi-dimensional and non-linear problems. It accounts for the physical properties of materials and their behaviors under different conditions, making it a robust choice for structural assessments. However, FEA can be time-consuming and may require specialized software and expertise to set up and run the simulations properly.
Practical Applications of Gamma Ray Shielding
Gamma ray shielding plays a critical role in ensuring safety and efficacy across various fields like medicine, nuclear technology, and industry. The ability to effectively shield against harmful gamma radiation is not just a matter of compliance but also one of safeguarding health and enhancing operational efficiency. With many potential sources of gamma radiation, understanding where and how to apply effective shielding is paramount. Whether in medical imaging, nuclear reactors, or manufacturing environments, the knowledge of practical applications can help mitigate risks associated with radiation exposure.
In Medical Settings
Radiology
Radiology stands out as a significant application of gamma ray shielding in the medical field. It utilizes imaging techniques that often rely on ionizing radiation, making effective shielding crucial. The primary characteristic of radiology is its emphasis on precise imaging for diagnostics. High-quality images from gamma sources, such as gamma cameras, help identify conditions deep within the body with minimum patient discomfort. Choosing the right shielding material, like lead, ensures that unnecessary exposure to radiation is minimized for both the patient and healthcare providers.
The unique feature of radiology is its dual need for accuracy and safety. While techniques like computed tomography can offer detailed insights, they also expose patients to varying levels of gamma radiation. Thus, effective shielding is beneficial in maintaining the delicate balance between obtaining critical diagnostic information and protecting against radiation harm.
Radiation Therapy
Radiation therapy represents another vital application that utilizes gamma ray shielding extensively. This method employs high doses of radiation to target cancer cells while minimizing damage to surrounding healthy tissues. The key characteristic that defines radiation therapy is its therapeutic intention, which fundamentally sets it apart from diagnostic radiology. In this context, the shielding does not just serve to protect; it becomes an integral part of the treatment process.
The special aspect of radiation therapy is the careful planning involved to effectively deliver radiation precisely where it’s needed while shielding the rest of the body. Although highly effective in treating various cancers, it poses challenges such as potential collateral damage or exposure to medical staff. Therefore, the choice of shielding material and design is carefully crafted to optimize outcomes and minimize risks.
In Nuclear Facilities
Nuclear Power Plants
In nuclear power plants, effective gamma ray shielding is indispensable for both safety and operational efficiency. Here, the use of thick concrete and lead barriers serves as a critical line of defense against radiation emitted during nuclear fission. The defining characteristic of nuclear power plants is the high radiation levels they generate; thus, robust shielding is not just beneficial, but a regulatory requirement that protects plant workers and the surrounding community.
The unique feature of nuclear power plants lies in their continuous operation under high radiation. Therefore, they incorporate extensive shielding designs that are regularly evaluated and upgraded. While the benefits are significant in keeping exposure low, the initial installation and periodic examination can be quite costly, presenting a challenge in keeping facilities economically viable.
Research Reactors
Research reactors also significantly employ gamma ray shielding, primarily for educational and experimental work. These reactors are designed for research purposes, involving neutron and gamma radiation generation. One of their key characteristics is their flexibility, as they can be configured for different types of research, making effective shielding paramount to protect researchers and students.
A unique aspect of research reactors is the emphasis on safety protocols, which includes extensive shielding measures. However, these setups often pose logistical challenges—researchers must balance the need for access versus safety. The advantage of utilizing advanced shielding materials continues to grow, enabling more effective experiments with a reduced radiation risk.
In Industrial Applications
Radiation Measurement
In industrial settings, radiation measurement plays a vital role, particularly in sectors like oil & gas or manufacturing. Instruments that monitor radiation levels need effective shielding to achieve accurate readings without interference from gamma rays. A key characteristic is that treated shielding enhances precision in detection. By accurately measuring radiation, companies can ensure that operations remain within safety parameters and comply with regulatory standards.
The specific feature of radiation measurement technologies is the blend of reliability and sensitivity. However, safeguarding personnel while gathering accurate data can be a conundrum. The advantage lies in utilizing materials that provide both safety and responsiveness, although the costs of sophisticated devices may deter some companies from upgrading their systems.
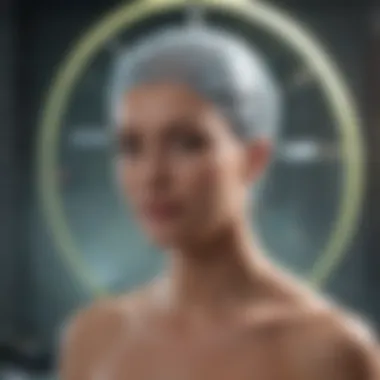
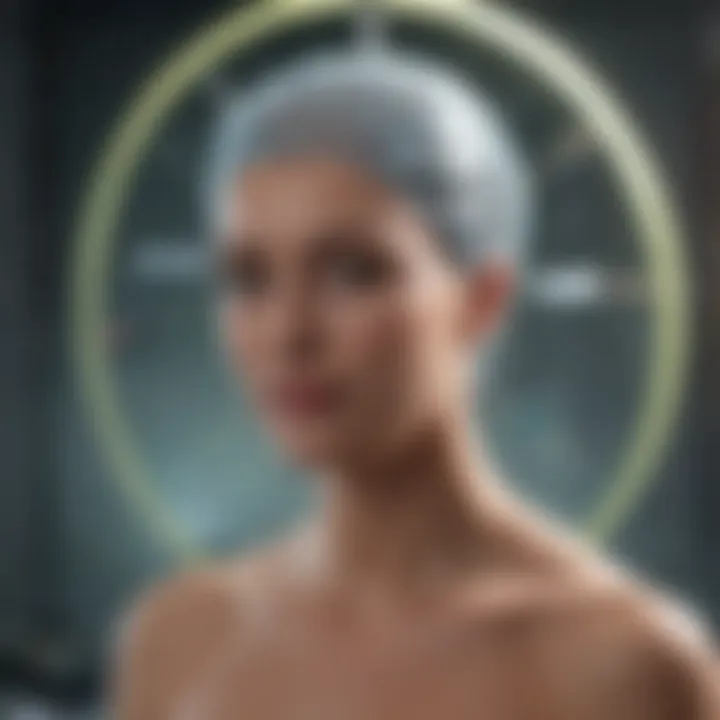
Quality Control
Quality control, particularly in manufacturing processes, is another vital area where gamma ray shielding is applied. This technique involves using radiation to inspect the quality of products, ensuring they meet safety and performance standards. The key characteristic of this application is that it facilitates non-destructive testing, enabling manufacturers to maintain quality without damaging the product.
The unique aspect of quality control through radiation testing is its efficiency in producing reliable data quickly. While effective, companies must still consider the safety regulations that come with handling radiation, making the selection of appropriate shielding materials crucial. The challenge lies in balancing the need for rigorous testing with the costs and responsibilities associated with radiation safety.
Gamma ray shielding is not merely about keeping radiation contained; it's a vital aspect of ensuring operational integrity and safeguarding health across various applications.
Regulatory and Safety Considerations
Regulatory and safety considerations represent a fundamental aspect of gamma ray shielding. This subsection acknowledges the necessity to establish clear guidelines and standards to ensure that individuals, equipment, and the environment are sufficiently protected from the harmful effects of gamma radiation. Proper adherence to regulations not only promotes safety in various industries, including medical and nuclear sectors, but also bolsters public confidence in radiation practices.
Establishing a robust framework for regulatory compliance involves several critical elements. First off, oversight organizations such as the International Atomic Energy Agency (IAEA) and national regulatory bodies set up regulations that are meant to protect workers and the general public. Compliance with these guidelines can significantly reduce the risk of radiation exposure. Additionally, it helps in creating a uniform standard across different facilities which is crucial for both local and international operations.
Benefits to adhering to these regulations cannot be overstated. For instance, consistent monitoring and evaluation can lead to improvements in shielding effectiveness and safeguards against radiation leaks. This regulatory framework ties into the broader objective — to ensure that protective measures evolve alongside advances in technology and research.
Such guidelines may dictate:
- Design specifications for shielding materials used in various applications.
- Regular safety audits and assessments ensuring that standards are adhered to.
- Training protocols for personnel who work with or around gamma radiation.
With the growing acknowledgment of radiation risks, it's essential that both organizations and individuals engage with these regulations actively. Properly enforced, these safety protocols contribute to a culture of accountability and proactive risk management in industries that utilize gamma rays.
International Guidelines
Several international guidelines play crucial roles. These include standards set forth by well-known organizations. The IAEA provides frameworks that govern radiation safety and lend support for safe practices in both medical and industrial settings. The guidelines address multiple aspects of gamma ray shielding, from how to select appropriate materials to establishing safe exposure limits for workers and the public.
Moreover, the World Health Organization (WHO) outlines health-based recommendations for safe exposure to radiation in healthcare. Maintaining alignment with such guidelines ensures an ethical approach to radiation handling and promotes public trust.
Best Practices for Shielding
To efficiently protect against gamma radiation, best practices for shielding must be not only adhered to but continuously revised as technology advances. Here are some essential elements:
- Regular Training: Personnel should undergo ongoing training to cement knowledge of proper shielding techniques and compliance requirements. This continuous learning model ensures that the workforce remains informed about the latest safety protocols.
- Material Selection: Choose shielding materials that best suit the specific gamma radiation you encounter. For example, lead is often preferred due to its high density, while high-density polyethylene can be effective in different contexts.
- Design Considerations: Shielding design plays a vital role in efficiency. Opt for configurations that maximize shielding effectiveness while minimizing space and cost.
- Monitoring Procedures: Implement continuous monitoring for radiation levels in and around the facility. This practice aids in recognizing any immediate concerns before they become detrimental.
- Public Communication: Maintain transparency with the public about radiation safety measures and potential risks associated with gamma ray use.
"Effective radiation safety is a collective responsibility. Compliance with regulations ensures both the safety of individuals and the longevity of operational practices in radiation environments."
By integrating these best practices into daily operations and developing a thorough understanding of regulatory expectations, professionals can create a safer environment, fostering trust in radiation-utilizing industries.
Ongoing Research in Gamma Ray Shielding
Research into gamma ray shielding continuously pushes boundaries, seeking better methods to protect individuals and sensitive equipment from radiation. This aspect is vital not only for enhancing safety in medical and nuclear sectors but also for addressing environmental and material sustainability concerns.
Innovative Materials
Nanomaterials
Nanomaterials represent a fascinating area in gamma ray shielding due to their small size, granting unique characteristics. Utilizing substances like carbon nanotubes or metal nanoparticles can lead to significant improvements in absorption efficacy. A key characteristic of nanomaterials is their high surface area-to-volume ratio, which augments their capability to interact with gamma radiation effectively. This quality makes them a beneficial choice in modern shielding applications.
One unique feature of nanomaterials is their lightweight nature, making them less cumbersome compared to traditional shielding materials such as lead. This reduction in weight is crucial in settings where portability is necessary, such as in mobile diagnostic systems. However, handling and integrating these materials into existing systems can present challenges, given the need for precise manufacturing methods and potential cost implications.
Composite Shielding Solutions
Composite shielding solutions are another crucial innovation in this realm. They involve blending various materials to design multi-layered systems that enhance overall shielding effectiveness. A fundamental aspect of composite solutions is their tailored ability to meet specific shielding requirements. This makes them a popular solution among researchers and industry professionals alike.
The unique feature of composite materials lies in their ability to combine the strengths of different materials, such as high density and high atomic number substances, to create a more effective barrier against gamma radiation. Their advantages include increased flexibility regarding design and performance. Still, they may come at a higher expense due to the complexity of material combinations and fabrication processes.
Emerging Technologies
Advanced Detection Techniques
Advanced detection techniques are crucial in improving gamma ray shielding, enhancing our ability to monitor radiation levels accurately. Key features of these techniques often include rapid data acquisition and improved sensitivity in detection. This makes them an effective addition to shielding strategies, helping to assess exposure in real-time effectively.
A distinctive feature of advanced detection systems is their integration with modern software and analytics, allowing for immediate response actions. While implementing these systems may require initial investments in technology and training, the long-term benefits often outweigh these considerations. They facilitate better protective measures, ultimately leading to a safer environment.
Real-time Monitoring Systems
Real-time monitoring systems have emerged as a vital component in the landscape of gamma ray shielding. Their capacity to provide ongoing assessment of radiation levels not only ensures compliance with safety regulations but also enhances operational awareness. A defining characteristic of these systems is their ability to alert personnel promptly when levels cross predefined thresholds.
One unique aspect is the integration of IoT (Internet of Things) technologies, allowing for remote monitoring and control. Although the deployment of such systems might entail significant upfront costs, the advantage lies in preventing exposure incidents and promoting a culture of safety.
"Investing in real-time monitoring is not just a matter of compliance; it's about fostering a proactive safety culture."
With the ongoing developments in gamma ray shielding, it’s clear that innovation is driving both safety and efficiency in various applications. As these materials and technologies evolve, they present exciting possibilities for improved radiation protection, ultimately benefitting both professionals in the field and society at large.
The End
The conclusion of this article serves as a pivotal juncture, bringing together the multitude of threads woven throughout our exploration of gamma ray shielding. It’s important to distill the vast information into bite-sized pieces that resonate, making clear why the study and application of gamma ray shielding is not merely a scientific endeavor but a cornerstone of safety and innovation. By mastering the principles behind shielding techniques and materials, professionals in various fields can mitigate risks associated with gamma radiation effectively.
Summary of Key Points
The preceding sections paint a comprehensive picture of gamma ray shielding. Here are the key takeaways:
- Nature of Gamma Rays: These high-energy photons are prevalent in various environments and necessitate careful management.
- Physical Principles: A grasp of interactions like photoelectric effect and Compton scattering shapes our understanding of how gamma rays behave when encountering different materials.
- Materials and Their Properties: Diverse materials, including lead, concrete, and advanced composites, each possess unique shielding properties, offering various advantages depending on the application.
- Mathematical Framework: We’ve explored essential equations that guide shielding evaluations, imperative for informed decision-making in both design and implementation.
- Applications Across Fields: From healthcare to industrial settings, these materials and methods bear significant implications. Each case highlights the necessity for stringent safety regulations and the application of best practices.
- Ongoing Research: As science progresses, the promising developments in nanomaterials and real-time detection systems point to a future rich with potential enhancements in shielding effectiveness.
Future Directions in Shielding Research
Looking ahead, there is a wealth of potential paths for further exploration:
- Innovative Materials: The quest for lighter, more effective shielding materials continues, with a keen interest in nanotechnology and composites that can offer the same level of protection with less weight. The adaptability of these materials can dramatically affect efficiency in various applications.
- Real-Time Monitoring: Advances in sensors and smart technology can facilitate continuous monitoring of radiation levels. This leads to proactive safety measures, ensuring environments remain within permissible exposure limits.
- Enhanced Computational Models: Virtual simulations using techniques like Monte Carlo might evolve, yielding more precise models for shielding design and evaluation. These models will allow for tailored solutions based on specific needs and conditions, thus improving overall effectiveness.
- Interdisciplinary Collaboration: Bridging gaps between fields like physics, engineering, and environmental science can yield insights that drive innovation in shielding materials and methodologies.
In essence, gamma ray shielding is not merely a technical measure; it is an essential aspect of ensuring safety in environments where radiation is present. The ongoing research underscores our commitment to improving strategies to protect public health while embracing technological advances that further enhance our capabilities.