Gas Chromatography: An Analytical Overview
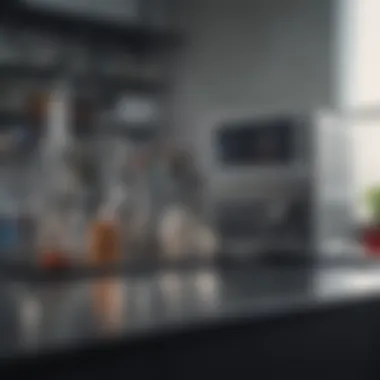
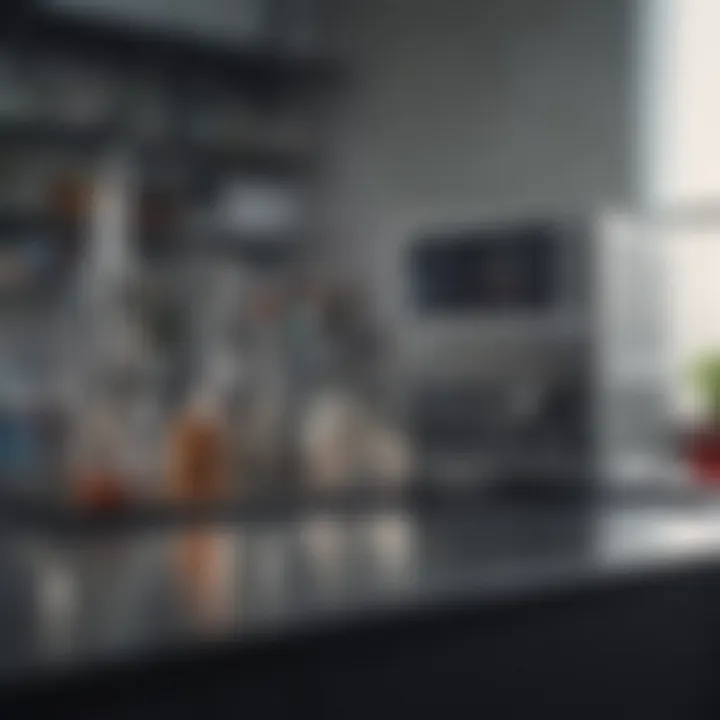
Intro
Gas chromatography (GC) is a powerful analytical technique used to separate and analyze compounds that can vaporize without decomposition. It plays an essential role across multiple scientific disciplines including chemistry, environmental science, forensics, and pharmaceuticals. Understanding gas chromatography is vital for students and professionals who seek to apply this method for qualitative and quantitative analysis of complex mixtures.
The technique involves passing a sample through a column filled with a stationary phase while a carrier gas flows continuously. As the sample components partition between the stationary phase and the moving gas, they separate based on their volatility and interaction with the stationary phase. This separation leads to a unique output read in the format of a chromatogram, providing valuable insights into the sample's composition.
In this article, we will explore gas chromatography in depth. We will discuss its fundamental principles, key components, various applications, and advancements in methodologies. Furthermore, we will examine the implications of gas chromatography in scientific research. Such a comprehensive discussion will benefit educators, students, and industry professionals alike.
Prelude to Gas Chromatography
Gas chromatography (GC) plays a crucial role in analytical chemistry and other scientific disciplines. This section presents an overview of its significance, providing context and relevance in various fields of research and industrial applications.
Definition and Significance
Gas chromatography is an analytical technique used to separate and analyze compounds in a mixture. The method relies on the interaction of vaporized samples with a stationary phase to facilitate separation. The significance of this technique lies in its ability to provide high-resolution data on volatile and semi-volatile substances with a high degree of sensitivity.
The applications of gas chromatography span numerous industries, such as pharmaceuticals, environmental monitoring, and food safety. The technique is instrumental for quality control, where precise measurements and detection of impurities are necessary. Furthermore, the sensitivity of GC enables the detection of consistently low concentrations of substances, making it an invaluable tool in laboratory analyses.
Historical Context
The roots of gas chromatography trace back to the 1940s, with its development attributed to pioneers like Archer J. P. Martin and Anthony T. James. They laid the groundwork for what would become a dominant analytical technique. The introduction of the first commercial gas chromatograph in the 1950s marked a significant evolution in analytical chemistry. Over the decades, advancements in technology, such as the introduction of capillary columns and mass spectrometry coupling, have transformed the field, increasing both the efficiency and range of applications for gas chromatography.
The historical development of gas chromatography is essential for appreciating current methodologies and their applications. Understanding where gas chromatography started sets a clear trajectory for interpreting its advancements and future potential.
Principles of Gas Chromatography
Understanding the principles of gas chromatography is essential for grasping its applications in scientific analysis. This section outlines the core concepts governing this technique. The focus on the underlying mechanisms and separation phases provides insight into why gas chromatography is a preferred method in various fields, including environmental, food, and pharmaceutical analysis.
Basic Operational Mechanism
Gas chromatography operates on the principle of partitioning compounds between a stationary phase and a mobile phase. The mobile phase in this context is an inert gas, commonly nitrogen or helium, which serves to transport the sample vapor through the chromatographic system. The process involves injecting a small volume of the liquid or gaseous sample into the injector column. Here, the sample is vaporized and pushed into a column where it interacts with the stationary phase coated inside the column.
Each component of the sample has a unique affinity to the stationary phase, whereby it is retained for a distinct amount of time, known as the retention time. This difference in retention is the basis of separation.
- Injection: The sample is introduced into the system using either a split or splitless injector.
- Vaporization: Upon injection, the sample is instantly vaporized before entering the column.
- Separation: As the compounds travel through the column, they separate based on their interactions with the stationary phase.
This basic operational mechanism underscores gas chromatography's efficiency in analyzing complex mixtures, allowing for the identification and quantification of various components within samples.
Phases of Separation
The separation in gas chromatography occurs through several distinct phases. Understanding these phases is vital to assess the efficiency and capability of the technique.
- Mobile Phase Movement: The inert gas carries the vaporized sample through the column, facilitating movement and separation.
- Stationary Phase Interaction: As the sample passes through, different compounds will interact differently with the stationary phase, leading to varied retention times.
- Detection Phase: After separation, the individual components exit the column separately and are detected, typically using a detector like a flame ionization detector or a mass spectrometer.
The phases of separation emphasize the role of affinity differences in the efficiency of gas chromatography. Each stage requires meticulous control to ensure optimal results, thereby influencing the overall sensitivity and selectivity of the analysis. In this way, the principles of gas chromatography not only facilitate separation but also enhance analytical accuracy and repeatability, making it invaluable in scientific research.
Key Components of Gas Chromatography
Gas chromatography is a sophisticated method that relies on a few critical components to function effectively. Each element plays a significant role in ensuring the precision and reliability of the analysis. Understanding these components is fundamental for anyone engaged in analytical chemistry, as they directly affect the outcomes of experiments and the quality of data collected.
The Injector System
The injector system is a vital part of gas chromatography. Its main function is to introduce a small volume of liquid or gaseous sample into the system. This component is carefully designed to minimize sample loss and ensure that the introduction of the sample is as efficient as possible. One common type of injector is the split/splitless injector, which allows users to control how much of the sample goes into the column.
Here are some benefits of a well-functioning injector system:
- Accuracy: Precise injection leads to better reproducibility of results.
- Flexibility: The system can handle a variety of sample types and volumes.
- Sensitivity: Minimizing sample loss enhances detection limits.
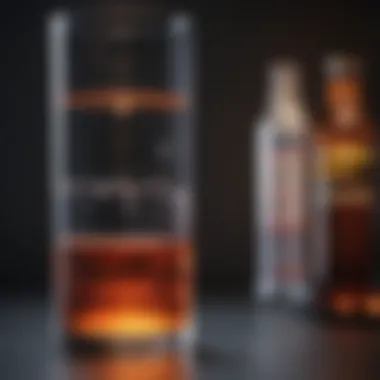
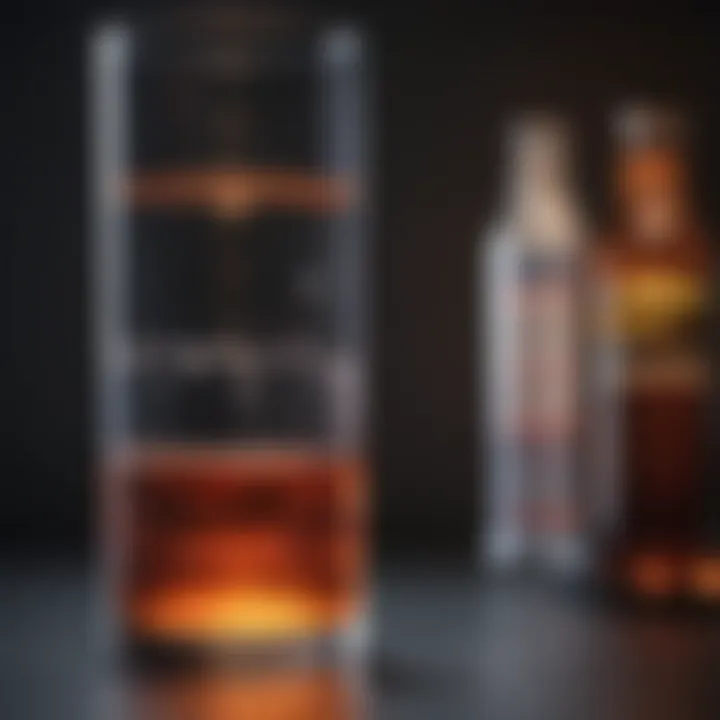
The Column
The column is perhaps the most critical component in gas chromatography. It is where separation takes place. Typically, it is a long, narrow tube filled with a stationary phase that interacts with the sample components as they travel through. There are different types of columns, such as packed columns and capillary columns. Each type has its specific applications depending on the nature of the sample being analyzed.
Capillary columns are particularly favored for their efficiency and high resolution. Their thin structure provides a larger surface area for interaction with the stationary phase.
The choice of column directly influences the resolution of the chromatogram, affecting the overall analytical outcomes.
The Detector
The detector serves to identify and quantify the separated compounds exiting the column. Various types of detectors are available, and the selection often depends on the specific requirements of the analysis. Popular detector types include the flame ionization detector (FID) and the thermal conductivity detector (TCD).
Key aspects of the detector include:
- Sensitivity: Effective detectors can identify compounds at very low concentrations.
- Selectivity: The ability to distinguish different compounds within a mixture is crucial for accurate analysis.
- Response time: Quick response allows for better real-time data collection.
Understanding these key components provides a clearer view of how gas chromatography functions. Each element is intricately connected to others, contributing to the effectiveness of this analytical technique.
Gas Chromatography Techniques
Gas chromatography techniques play a crucial role in the field of analytical chemistry. These methodologies ensure that the separation and identification of chemical compounds are done with accuracy and efficiency. Understanding the specific techniques in gas chromatography allows scientists and researchers to customize their analysis depending on the nature of the sample and the compounds of interest.
Type of Columns Used
The choice of column in gas chromatography is vital for effective separation. There are various types of columns, but they mainly fall into two categories: packed columns and capillary columns.
- Packed Columns: These contain a solid stationary phase and are generally larger in diameter than capillary columns. They can handle larger sample volumes, making them suitable for particular applications where high throughput is necessary. However, packed columns tend to show less efficiency in terms of separation power compared to capillary columns.
- Capillary Columns: These are narrow and long, providing a larger surface area for interaction between the sample and the stationary phase. The thin design enhances separation efficiency, making capillary columns ideal for complex mixtures. Furthermore, they can operate with lower pressure, which reduces the risk of sample degradation. Selecting the right column type is often determined by the desired resolution and the physical characteristics of the sample being analyzed.
Types of Detectors
Detectors in gas chromatography are essential for identifying and quantifying the separated compounds. Several types of detectors are utilized, with varying sensitivities and specificities. Here are some key types:
- Flame Ionization Detector (FID): FID is one of the most commonly used detectors. It measures ions produced from the combustion of organic compounds in a hydrogen flame. It is highly sensitive to hydrocarbons but does not respond to inorganic compounds like water or carbon dioxide.
- Thermal Conductivity Detector (TCD): This detector measures changes in the thermal conductivity of the gas as it flows through the detector. TCD can detect a wide range of compounds, including inorganic gases, making it versatile. However, it is generally less sensitive than FID.
- Mass Spectrometer (MS): When coupled with gas chromatography, mass spectrometry becomes a powerful tool for identifying compounds based on their mass-to-charge ratio. This method offers highly specific identification, making it a preferred choice in complex analyses.
Choosing an appropriate detector involves considering sensitivity needs, sample composition, and desired outcome of the analysis.
Temperature Programming
Temperature programming is a critical technique in gas chromatography that enhances the efficiency of the separation process. It involves the gradual increase of the column's temperature during the analysis. The significance of temperature programming lies in its ability to manage retention times for various compounds effectively.
- Initial Temperature: A low starting temperature may help retain less volatile components for a longer duration.
- Ramp Rate: The rate at which the temperature increases can be adjusted, optimizing the separation of compounds with significantly different boiling points.
- Final Temperature: Depending on the compounds being analyzed, a higher final temperature can provide a more comprehensive elution profile, ensuring that all components are analyzed within a reasonable timeframe.
Integrating temperature programming allows for improved resolution and sensitivity. Ultimately, it enables scientists to capture comprehensive data on complex mixtures more effectively.
Understanding the techniques of gas chromatography is crucial for achieving precise analytical results. The selection of columns, detectors, and temperature programming can significantly influence the outcomes of experiments.
Applications of Gas Chromatography
Gas chromatography (GC) stands as a cornerstone in analytical chemistry, with diverse applications spanning multiple disciplines. Understanding these applications is essential for grasping the true significance of this analytical technique. This section explores several critical areas where gas chromatography plays a vital role. The emphasis lies in the specific benefits and considerations of using GC in distinct fields, demonstrating its adaptability and reliability in analyzing complex mixtures.
Environmental Analysis
Environmental analysis represents a significant application of gas chromatography. GC is extensively utilized to assess air, water, and soil quality, offering crucial insights into pollution levels and environmental health. For instance, volatile organic compounds (VOCs) emanating from industrial sites can be accurately quantified using gas chromatography. The method's high sensitivity allows for detection of trace levels of contaminants, aiding regulatory agencies in enforcing environmental standards. Moreover, the ability to separate mixtures effectively ensures that the data collected is both precise and reliable.
"Gas chromatography has become an essential tool for environmental monitoring due to its unmatched sensitivity and speed of analysis."
Additionally, GC can be combined with mass spectrometry (MS) for enhanced analytical capability. This conjunction provides qualitative and quantitative information about complex environmental samples, allowing for a more thorough understanding of pollutant behavior and degradation pathways. The results contribute significantly to developing pollution control strategies and public health policies.
Food and Beverage Testing
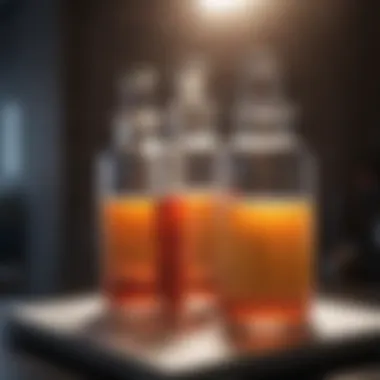
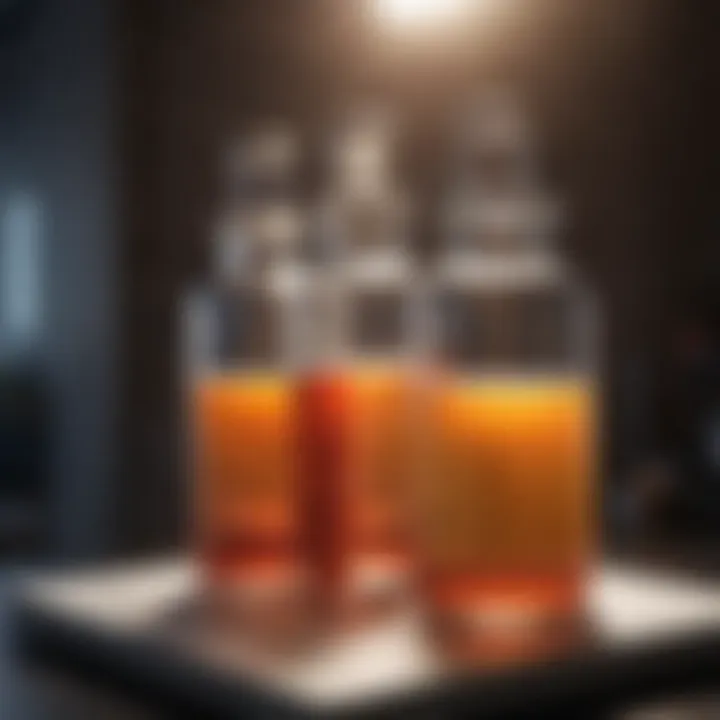
Food quality and safety remain paramount in the food industry. Gas chromatography plays a critical role in food and beverage testing. It ensures that products meet safety standards and are free of harmful contaminants. Common applications include analyzing flavor compounds, assessing food adulterants, and studying the stability of aromas in beverages. For example, testing for pesticide residues in fruits and vegetables can be efficiently carried out using gas chromatography, providing essential data for consumer safety and regulatory compliance.
GC is also instrumental in evaluating essential oils and fragrances in the food sector. By separating the various volatile compounds, manufacturers can ensure product consistency and quality, thereby maintaining consumer trust and brand integrity. Moreover, the fast analysis time ensures that companies can respond quickly to any safety concerns.
Pharmaceutical Industry
In the pharmaceutical industry, gas chromatography aids in the development and quality control of medications. Its ability to separate and quantify chemical compounds is vital for ensuring the purity and potency of drugs. GC is commonly used to analyze active pharmaceutical ingredients (APIs) and excipients, verifying that formulations meet stringent regulatory guidelines.
The technique also facilitates the detection of impurities and contaminants, which is crucial in maintaining the safety and efficacy of pharmaceutical products. With the recent shift towards biologics and complex molecules, the integration of gas chromatography with advanced detection methods, such as mass spectrometric detection, enhances the analysis of drug formulations, leading to improved patient outcomes.
GC’s role in rapid analysis contributes to the efficiency of pharmaceutical development processes, allowing researchers to make data-driven decisions quickly, thereby reducing time-to-market for new drugs. Furthermore, its applications extend to stability testing, ensuring drugs remain effective throughout their shelf life.
In summary, gas chromatography’s applications across environmental analysis, food testing, and the pharmaceutical industry underscore its importance as an analytical tool. The technique’s adaptability, coupled with its precision and reliability, positions it as a preferred method in various sectors, affirming its integral role in modern scientific analysis.
Advantages of Using Gas Chromatography
Gas chromatography offers several important benefits that make it a preferred analytical method in many fields. Understanding these advantages is crucial for students, researchers, and professionals who rely on precise analytical techniques. The following sections will explore specific elements, providing clarity on why gas chromatography is essential.
High Sensitivity and Selectivity
One of the primary advantages of gas chromatography is its high sensitivity. This means it can detect even minute quantities of substances in a sample. This sensitivity is particularly important in fields such as environmental science and pharmaceuticals, where trace levels of contaminants or active ingredients may significantly impact results. The ability to measure down to parts per billion (ppb) enhances the reliability of findings, fostering more accurate conclusions in scientific studies.
Selectivity is another crucial aspect of gas chromatography. It allows for the distinction between closely related compounds in a complex mixture. This feature is vital for situations where multiple analytes may interfere with each other. For instance, in food and beverage testing, the technique can differentiate flavors and odors properly, ensuring quality control. The combination of both high sensitivity and selectivity ensures that the results are not just accurate but also relevant.
"Gas chromatography can identify and quantify components in a mixture with remarkable precision."
The role of the stationary phase in the column further aids in enhancing these qualities. Tailoring the stationary phase to match the specific properties of target compounds allows for optimized separations, which is a fundamental advantage of using gas chromatography over other techniques.
Quick Analysis Time
Another significant advantage is the quick analysis time associated with gas chromatography. The entire process, from sample injection to result output, can often be completed in a matter of minutes. This efficiency is a considerable advantage in various applications, such as routine quality checks in laboratories or rapid field testing. The rapid turnaround not only speeds up research but also enhances productivity in laboratories.
Moreover, the quick analysis time allows for real-time monitoring of chemical processes. For industries that require timely data, such as petrochemicals or chemical manufacturing, the ability to obtain results swiftly means better decision-making and process optimization.
Some laboratories even manage to run multiple samples simultaneously, effectively doubling the throughput without compromising quality. These efficiencies are paramount in today's fast-paced scientific environments, where time-sensitive results can have significant implications.
In summary, the advantages of using gas chromatography lie in its high sensitivity, selectivity, and quick analysis time. Understanding these benefits provides a solid foundation for appreciating the technique's critical role in various scientific disciplines.
Limitations of Gas Chromatography
Gas chromatography is a powerful analytical technique with numerous applications; however, it is not without its limitations. Understanding these limitations is crucial for scientists and professionals who rely on this method for analytical purposes. Recognizing potential drawbacks ensures that proper precautions and considerations are taken when interpreting results. Below, we delve into two primary limitations: sample preparation challenges and the incompatibility with certain compounds.
Sample Preparation Challenges
Effective sample preparation is a fundamental step in analytical chemistry, and gas chromatography is no exception. Preparing samples for gas chromatography requires significant attention to detail. Contamination can skew results and lead to inaccurate quantification.
Some notable challenges in sample preparation include:
- Volatile Components: Samples containing volatile compounds can evaporate during preparation, resulting in loss and inaccurate analysis.
- Matrix Effects: Complex samples may contain interferents that affect the separation efficiency during the chromatographic process.
- Concentration Variability: Low concentrations of target analytes may pose difficulties for detection. Techniques like liquid-liquid extraction or solid-phase microextraction can mitigate this, but they often introduce additional complexity.
Due to these challenges, careful method development and validation are essential. Researchers must optimize parameters to reduce variability and enhance reproducibility.
Incompatibility with Certain Compounds
Gas chromatography is not universally applicable to all compounds. Several types of substances pose compatibility issues, which can limit the technique's effectiveness.
- Complex Polymers: Large, heavy molecules do not vaporize efficiently and often cannot be analyzed using conventional gas chromatography techniques.
- Thermally Labile Compounds: Chemicals that decompose at elevated temperatures cannot withstand the conditions required for gas chromatography and, thus, cannot be analyzed directly.
- Polar and Ionic Compounds: Some polar compounds do not partition well in the stationary phase of a gas chromatograph, leading to poor separation and detection.
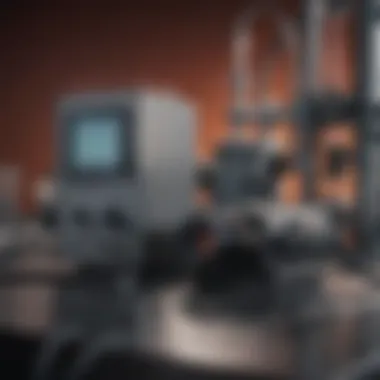
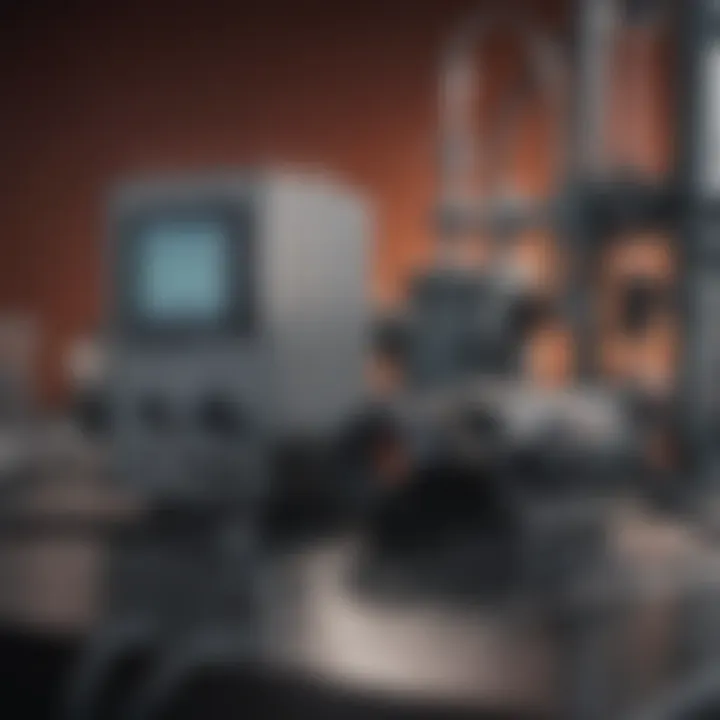
Scientists must choose alternative analytical methods, such as liquid chromatography or mass spectrometry, for these incompatible analytes. This necessitates a thorough understanding of the analyte characteristics to select the most suitable analytical approach.
Understanding the limitations of gas chromatography is essential for effective analysis. Researchers must be aware of potential challenges in sample preparation and the compatibility of certain compounds to ensure reliable results.
Recognizing these limitations not only aids in better planning of experiments but also enhances the interpretation of results, allowing for more informed conclusions in scientific research.
Advancements in Gas Chromatography
The field of gas chromatography has seen significant advancements that enhance its efficiency and versatility. As analytical demands increase, scientists strive for methods that not only improve separation and detection but also adapt to a wide range of applications. The advancements in gas chromatography reflect critical innovations that cater to both academic researchers and industry practitioners. These developments focus on refining existing techniques while integrating new technologies that promise to increase the accuracy and reliability of analyses.
Capillary Gas Chromatography
Capillary gas chromatography stands out as a prominent advancement in the field. Unlike traditional packed columns, capillary columns offer higher resolution and sensitivity due to their smaller inner diameters. The reduced diameter allows for more efficient separation of compounds by enhancing the interaction between the stationary phase and the analyte. This greater efficiency results in faster analysis times and lower sample requirements.
Capillary gas chromatography also allows for the use of various coatings, optimizing it for specific applications. For example, different stationary phases can provide tailored selectivity, improving the analysis of complex mixtures. Additionally, the technology has emerged to be essential in the fields of biomedical research and environmental monitoring, where detecting trace levels of substances is crucial.
Mass Spectrometric Detection
Mass spectrometric detection is another pivotal advancement in gas chromatography. This technique combines the separation capabilities of gas chromatography with the mass analysis of mass spectrometry. The integration of these two methods enhances the sensitivity and specificity of analyses significantly.
Mass spectrometers identify compounds based on their mass-to-charge ratios, providing high-resolution data on molecular composition. By combining mass spectrometry with gas chromatography, analysts gain more profound insights into complex samples. This synergy allows for the detection of low-abundance compounds, which is especially valuable in toxicology and forensic analysis.
Moreover, the implementation of mass spectrometric detection enhances quantification accuracy. It leads to reliable results, which are essential in regulated industries such as pharmaceuticals and food safety. The ability to characterize and quantify compounds with such precision helps in addressing regulatory requirements and advancing product safety.
"The combination of gas chromatography and mass spectrometric detection revolutionizes how analysts approach complex sample matrices."
Future Perspectives in Gas Chromatography
The future of gas chromatography (GC) is promising, with various developments on the horizon. As the demand for precision in analytical chemistry continues to rise, innovations in gas chromatography techniques are becoming increasingly important. These advancements offer benefits that could address current limitations and expand the usability of GC across diverse disciplines.
Integration with Other Analytical Techniques
The integration of gas chromatography with other analytical methods is essential for providing multifaceted insights into complex samples. Combining GC with techniques like mass spectrometry (GC-MS) or infrared spectroscopy adds a layer of depth to the analysis.
The integration brings several advantages:
- Enhanced Sensitivity: By pairing GC with mass spectrometry, analysts can detect compounds at lower concentrations, which is invaluable in fields such as environmental monitoring.
- Broader Application Scope: This combined approach allows for analysis of larger sets of data, making it possible to study various aspects of a sample simultaneously.
- Improved Resolution: By using these techniques together, scientists can separate compounds more effectively, which leads to better identification and quantification.
As labs strive for efficiency, this trend of integration will likely continue. It helps to rain in costs and improve analysis quality, addressing the need for precise results in various settings.
Emerging Trends in Automation
The trend toward automation in gas chromatography is a significant advancement. It aims to reduce human error and increase the throughput of analytical procedures. Automated systems can perform multiple analyses with minimal operator intervention, which is essential in labs with high demands.
Key aspects of this trend include:
- Increased Efficiency: Automated GC systems can process samples rapidly. This enhanced speed is particularly relevant in industries like pharmaceuticals, where time constraints are critical.
- Reduced Labor Costs: Automation helps to decrease the need for manual labor, thus allowing skilled technicians to focus on more complex analytical challenges.
- Consistent Quality Control: Automated processes ensure uniformity in sample handling and analysis, reducing variability in results.
Overall, as automation in gas chromatography continues to evolve, it will lead to streamlined workflows and improved data quality. The convergence of these advancements will enable researchers and industries to push the boundaries of what is currently possible in analytical techniques.
The future of gas chromatography is one where integration with other techniques and automation combine to create a more powerful analytical tool.
In summary, the future perspectives in gas chromatography underscore a significant shift towards improved methodologies and efficiencies. The integration with other techniques and advances in automation are not merely trends; they are the cornerstones of the evolution in analytical chemistry. With ongoing research and development, the capabilities and applications of gas chromatography are sure to expand, ensuring its vital role in scientific exploration and industry applications.
Culmination
The concluding section of this article emphasizes the central importance of gas chromatography within analytical chemistry. This technique is a pivotal tool that not only facilitates the precise separation of compounds but also enhances the identification and quantification of various substances across numerous fields such as environmental science, food safety, and pharmaceuticals.
Summary of Key Points
- Analytical Purpose: Gas chromatography serves as a highly efficient method for analyzing volatile and semi-volatile compounds.
- Applications: Its impact spans various domains including environmental monitoring, food and beverage quality control, and the pharmaceutical sector.
- Scientific Advancement: The technique has evolved through advancements like capillary columns and integrated mass spectrometry, improving its resolution and the ability to analyze complex mixtures.
- Advantages and Limitations: While it offers high sensitivity and quick turnaround times, challenges in sample preparation and compatibility with certain compounds remain significant.
- Future Developments: Emerging trends in automation and the integration of gas chromatography with other analytical techniques promise to enhance operational efficiency and expand application horizons.
Final Thoughts on the Impact of Gas Chromatography
Gas chromatography has reshaped analytical practices by introducing unmatched precision in compound analysis. Its adaptability in various fields signifies its importance. With ongoing developments, the future of this technique appears promising. It may continue to influence new research directions and lead to breakthroughs in various scientific disciplines. Its role in ensuring safety and quality cannot be overstated, making it an indispensable tool for students, researchers, and professionals alike. The continued evolution and integration of gas chromatography with other technologies stand to address current limitations, paving the way for more robust analytical methodologies.