Harnessing Solar Energy for Efficient Electricity Production
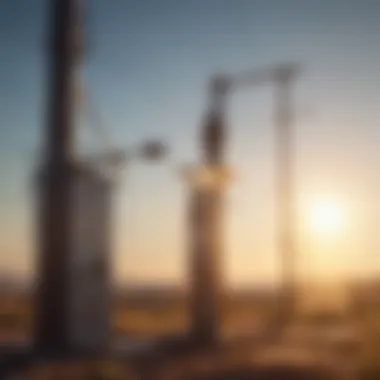
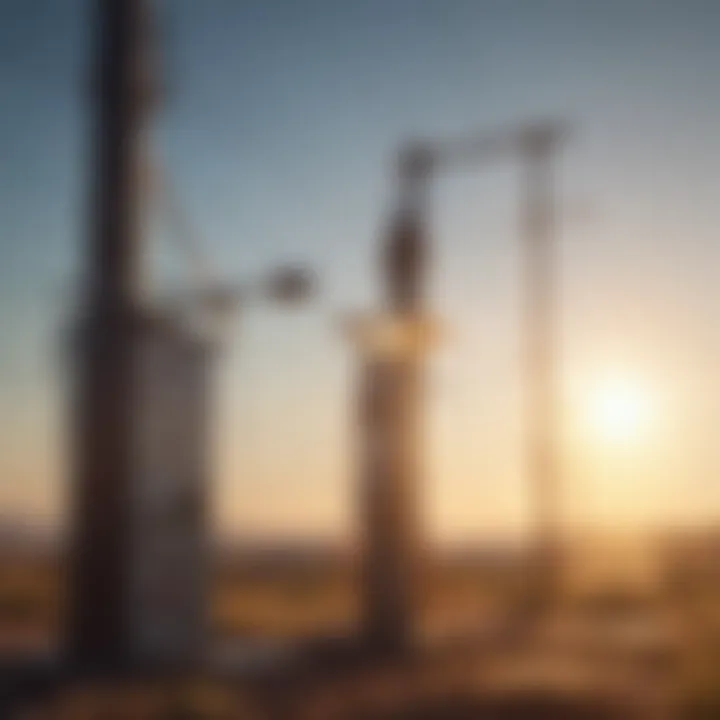
Intro
The transition to renewable energy sources has been a pressing subject in recent decades. Among these sources, solar energy stands out due to its accessibility and potential for widespread adoption. The process of converting solar energy into electricity is crucial for meeting energy demands while mitigating environmental risks.
Solar power harnessing involves two primary technologies: photovoltaic cells and solar thermal systems. Each offers unique advantages and applications that cater to different energy needs. Further examination of these mechanisms reveals their economic viability, performance optimizations, and implications for energy systems worldwide.
This article aims to provide a thorough understanding of how solar energy contributes to electricity production. Also, it discusses advancements in technology, economic impacts, and the environmental benefits tied to solar energy's wider adoption. By exploring these facets, the discussion provides a holistic view of solar energy's role in modern society and energy systems.
Research Highlights
Key Findings
- Photovoltaic cells convert sunlight directly into electricity using semiconductor materials.
- Solar thermal systems capture heat from sunlight, which can be used to produce steam and generate electricity.
- Technological advancements have increased efficiency rates in both photovoltaic and solar thermal systems significantly over the past few years.
- The economic implications of solar energy adoption include reduced reliance on fossil fuels and job creation in the renewable energy sector.
- Environmental benefits include reduced greenhouse gas emissions and lower air pollution levels compared to traditional energy sources.
Implications and Applications
The implications of harnessing solar energy extend beyond mere energy production. As societies increasingly shift towards sustainability, solar power becomes integral to energy policy and grid planning. Moreover, its implementation can enable energy independence for various communities, lessening the impact of volatile fossil fuel markets.
Solar energy can be integrated into existing infrastructure, such as residential buildings, commercial spaces, and even agricultural practices. Applications range from home solar panels to large-scale solar farms, which contribute significantly to national energy grids.
Preamble to Solar Energy
Solar energy serves as a cornerstone in the pursuit of sustainable electricity production. As global energy demand continues to rise, integrating renewable sources into the mix becomes not just beneficial but essential. This section explores solar energy, establishing its significance as a reliable alternative to traditional energy sources. The ongoing shift toward renewables is influenced by various factors including environmental concerns, energy security, and economic viability.
The concept of solar energy revolves around harnessing the sun's rays and converting them into usable forms of energy. This process can take various forms, including direct conversion to electricity via photovoltaic cells or capturing heat for thermal energy applications. The effective use of solar energy has the potential to alleviate reliance on fossil fuels, thus supporting global efforts towards mitigating climate change.
Defining Solar Energy
Defining solar energy involves understanding both its essence and its applications. Solar energy is essentially the radiant light and heat from the Sun, harnessed through a variety of technologies including solar panels and solar thermal systems. These systems convert sunlight into electricity or heat, depending on their design and intended purpose.
Solar photovoltaic (PV) systems play a significant role here, capturing sunlight directly to generate electricity. In contrast, solar thermal systems utilize sunlight to heat water or air. Both methods showcase the versatility of solar energy, allowing it to cater to diverse energy needs across residential, commercial, and industrial sectors. This adaptability underscores its relevance in modern energy discussions.
The Importance of Renewable Energy Sources
Renewable energy sources, such as solar, wind, and hydro, are paramount in addressing the world's energy challenges. Solar energy, in particular, offers several significant advantages:
- Sustainability: Unlike fossil fuels, solar energy is inexhaustible on a human timescale, making it a crucial asset for long-term energy planning.
- Reduced Environmental Impact: Harnessing solar power contributes to lower greenhouse gas emissions, thereby supporting global climate goals.
- Energy Independence: Increased reliance on local solar energy can enhance national energy security, reducing vulnerability to foreign energy supplies.
- Economic Growth: The solar energy industry has the potential to create numerous jobs and stimulate economic activity in manufacturing, installation, and maintenance.
"Investing in solar energy not only combats climate change, but it also creates job opportunities and promotes energy independence."
How Solar Energy Translates to Electricity
The process of converting solar energy into electricity is crucial for understanding the viability of this renewable resource. As global energy demands grow, utilizing solar energy presents sustainable options. This section discusses the mechanisms involved, emphasizing the photovoltaic effect and the overall components of a solar panel system. These elements work together to facilitate the efficient transformation of sunlight into usable electrical power.
The Photovoltaic Effect
The photovoltaic effect forms the heart of solar energy conversion. It occurs when sunlight strikes a solar panel and excites electrons within the material, typically silicon. This phenomenon is what enables solar cells to generate an electric current.
When photons from sunlight hit the silicon atoms, they transfer energy to electrons, allowing them to escape their atomic bonds. This process creates a flow of electrons, generating direct current (DC) electricity. However, most home appliances and the grid use alternating current (AC) electricity. Thus, an inverter is needed to convert DC into AC for practical use.
Understanding the photovoltaic effect is essential for several reasons:
- It forms the foundational principle behind most solar technologies.
- Grasping this concept helps assess the efficiency of solar panels.
- It allows for advancements in materials, design, and technology to improve energy output.
Components of a Solar Panel System
The functionality of a solar panel system relies heavily on its components. Each part plays a significant role in optimizing energy production and ensuring system longevity. Here are the main components involved:
- Solar Panels: The most visible component, these panels contain numerous solar cells that utilize the photovoltaic effect to convert sunlight into electricity.
- Inverter: This device converts DC electricity generated by the panels into AC electricity suitable for home use or feeding into the grid.
- Mounting Systems: These structures secure solar panels to rooftops or the ground, optimizing their angle to maximize exposure to sunlight.
- Battery Storage (optional): While not always included, solar battery systems store excess energy for use during cloudy days or at night. This feature adds resilience and flexibility to the power supply.
- Charge Controller (when batteries are used): This component regulates the charging of batteries to prevent overcharging and enhances battery life.
Together, these elements create a streamlined process for harnessing and converting solar energy into electricity efficiently. The choice of quality components can significantly impact overall performance and energy yield, stressing the importance of proper selection and installation.
Photovoltaic Solar Panels
Photovoltaic solar panels play a crucial role in the conversion of solar energy into electrical energy. These systems primarily harness sunlight and convert it directly into electricity through the photovoltaic effect. Understanding the specifics of photovoltaic solar panels is essential for various stakeholders, including students, researchers, and professionals interested in renewable energy technologies. The benefits of these systems extend beyond mere electricity production, influencing economic growth, environmental sustainability, and energy independence.
Types of Photovoltaic Cells
Within photovoltaic technologies, several types of solar cells exist, each with its own characteristics and benefits. The three main types are monocrystalline cells, polycrystalline cells, and thin-film solar cells. Each type has unique attributes that influence its application in solar energy systems.
Monocrystalline Cells
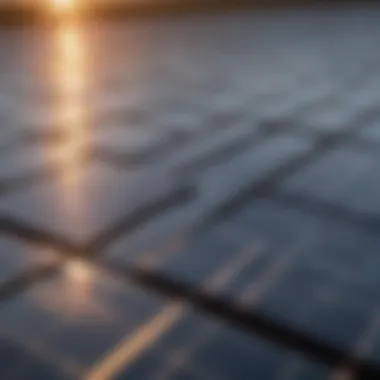
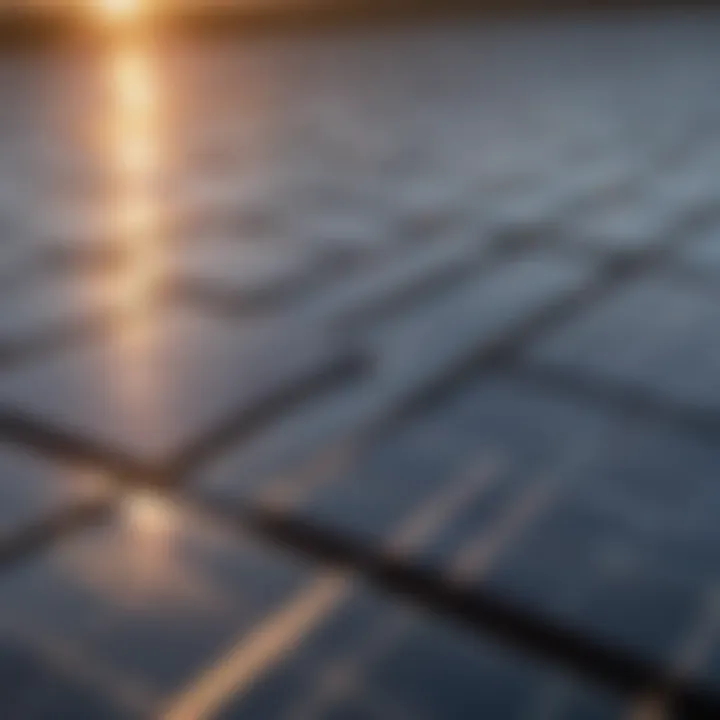
Monocrystalline cells are known for their high efficiency and space-saving design. They are made from a single crystal structure, which allows for better electron movement. One key characteristic is their purity, leading to more power output compared to other types at equivalent areas. This makes them a popular choice for residential solar installations where space might be limited.
The unique feature of monocrystalline cells is their longevity, often with warranties of up to 25 years. However, they come at a higher price point, which can be a disadvantage for some users. The investment is often justified by their efficiency and performance, especially in areas with high energy demand.
Polycrystalline Cells
Polycrystalline cells, on the other hand, are made from multiple crystal structures, which gives them a distinct blue hue. The manufacturing process is simpler and more cost-effective, making these cells a more economically attractive choice. They are favored in large-scale solar farms due to their lower production costs.
A notable characteristic of polycrystalline cells is their slightly lower efficiency compared to monocrystalline cells. They tend to occupy more space than crystalline counterparts for the same power output. This is an important consideration for large installations where space is less of a concern.
Thin-Film Solar Cells
Thin-film solar cells offer a different approach by using layers of photovoltaic material on a substrate. This type of solar cell is lightweight and flexible, allowing for innovative applications such as integration into building materials. One key advantage is their performance in low-light conditions, making them effective in diverse environments.
However, thin-film solar cells have lower efficiency ratings and require more area to produce equivalent power. Their lifespan is also shorter than crystalline cells, which can affect long-term viability. Their lightweight nature and versatility can provide unique advantages in specific applications though.
Efficiency Ratings and Performance
Efficiency ratings for each type of photovoltaic cell are a critical aspect when evaluating their performance. Higher efficiency ratings indicate a greater ability to convert sunlight into usable electricity, which is paramount in optimizing solar energy use in any application. The actual efficiency can depend on a myriad of factors including environmental conditions, installation methods, and site location.
Higher efficiency leads to better performance, reduced space requirements, and increased energy yield over time.
In addition to efficiency, performance also encompasses reliability and longevity, both of which affect overall energy production and return on investment. Understanding these factors aids stakeholders in making informed decisions about solar energy systems.
Solar Thermal Energy Systems
Solar thermal energy systems play a critical role in the landscape of renewable energy. They harness the sun's heat for the generation of electricity, primarily using thermal energy instead of relying solely on photovoltaic cells. This approach presents specific advantages, such as increased efficiency under certain conditions and the ability to provide thermal energy for various applications beyond electricity production.
How Solar Thermal Generation Works
Solar thermal generation utilizes sunlight to heat a fluid, which then produces steam to drive turbines. The process involves concentrating solar power (CSP) technology, which includes several key components designed to effectively capture and convert solar energy into usable electricity.
The fundamental mechanism rests on using mirrors to concentrate sunlight onto a receiver. The receiver absorbs heat and transfers it to a working fluid, which can be either water or a synthetic oil. This heat energy transforms the fluid into steam, which spins a turbine connected to a generator. The system allows for energy production, even when the sun isn't shining, by storing thermal energy.
Components of Solar Thermal Systems
Collecting Units
Collecting units are essential for the efficiency of solar thermal systems. They gather and concentrate sunlight. Typically designed as parabolic troughs or solar towers, these units direct the sun's rays onto a receiver. A key characteristic of collecting units is their ability to track the sun, thereby maximizing solar exposure throughout the day.
One beneficial feature is the reflective surface, which enhances light capture, leading to improved performance.
However, these systems can be more complex and expensive to install compared to flat-plate collectors. Their effectiveness often improves in direct sunlight but may less efficient during overcast conditions.
Heat Exchangers
Heat exchangers play a crucial role in transferring the heat collected by the working fluid to produce steam. They facilitate this transfer without mixing the working fluid and steam, ensuring efficient systems operation. A significant characteristic of heat exchangers is their high thermal conductivity, which aids in effective heat transfer.
This component is popular for its reliability and effectiveness in maintaining system efficiency, thereby improving energy output.
On the downside, they may require maintenance to ensure they remain efficient, especially in harsh operating conditions.
Storage Systems
Storage systems retain thermal energy, making solar thermal plants capable of producing electricity on demand. These systems are vital for improving overall energy reliability and availability, particularly during periods of low sunlight.
The primary characteristic of storage systems consists of their use of phase change materials or molten salt for heat retention. This allows energy to be used even when solar conditions are not ideal.
A unique feature is the ability to store heat for long durations, providing a buffer against fluctuations in energy supply. However, the installation of these systems can increase project costs, requiring careful cost-benefit analysis.
The integration of solar thermal systems provides a pathway for scaling renewable energy production. Their infrastructure can harness sun energy effectively to minimize dependence on fossil fuels.
Overall, solar thermal energy systems present a promising alternative for electricity generation, combining efficiency with sustainability.
Hybrid Systems
Hybrid systems represent a significant advancement in the way solar energy can be harnessed for electricity production. These systems combine solar energy with other renewable sources, such as wind or biomass, to create a more reliable and efficient energy supply. This integration allows for better energy management and reduced dependency on any single source. By leveraging the strengths of different energy types, hybrid systems can provide a solution that maximizes output while minimizing carbon emissions, thus aligning well with sustainability goals.
Combining Solar and Other Renewable Sources
When discussing hybrid systems, the combination of solar and other renewable sources becomes crucial. Solar energy systems, particularly photovoltaic and solar thermal, excel during sunny days, but their efficiency can be compromised during periods of cloud cover or night-time. By integrating wind energy, for example, the strength of that complementarity can be leveraged. Wind energy tends to peak at night or during stormy weather, balancing the limitations of solar generation.
In practice, a hybrid system might involve:
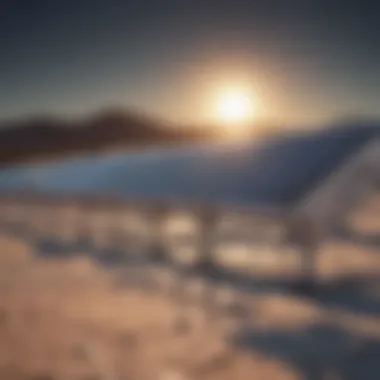
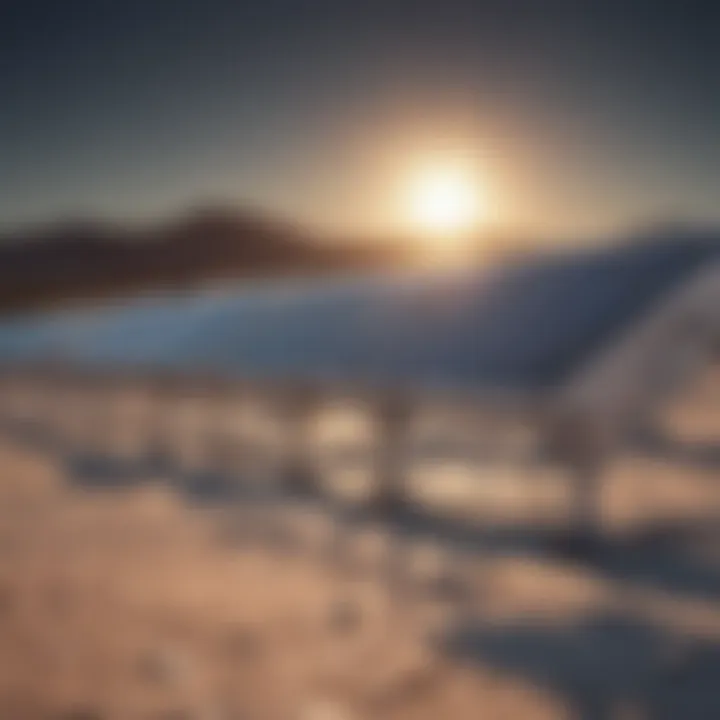
- Photovoltaic solar panels working in conjunction with wind turbines
- Utilizing hydroelectric power for storage and supplementary energy during low solar production
- Incorporating biomass systems to utilize organic materials that can be harvested in conjunction with other energy sources
Such combinations enhance reliability. Users can enjoy a more consistent energy supply without having to rely solely on the availability of sun or wind.
Advantages of Hybrid Energy Systems
Hybrid energy systems come with several advantages that can be critical for modern energy needs. First, they offer increased energy reliability. By diversifying the energy supply sources, these systems are better equipped to handle fluctuations in power generation and demand.
Secondly, hybrid systems often lead to economic benefits. Though the initial setup may require higher investment, the long-term savings on utility costs are significant. With effective energy management, these systems can reduce energy bills drastically.
Moreover, hybrid systems promote sustainability. Combining different renewable sources reduces overall dependency on fossil fuels, consequently decreasing greenhouse gas emissions. This move aligns with global initiatives aimed at combating climate change.
In addition to these benefits, a hybrid energy framework can stimulate technological growth. It encourages innovation as engineers and developers strive to improve hybrid technologies, making them more efficient and user-friendly.
Hybrid systems exemplify how innovation can lead to sustainable practices in energy production, strengthening the transition to a clean energy future.
Overall, the potential of hybrid systems in harnessing solar energy effectively cannot be overstated. They cater to an evolving landscape of energy needs, positioning themselves as central players in the transition to a sustainable energy future.
Energy Storage Solutions for Solar Energy
Energy storage is a critical aspect when discussing solar energy production. As solar energy is dependent on sunlight, it is intermittent in nature. This means that energy can be generated only when the sun is shining. Therefore, having effective energy storage solutions allows for the capture and retention of this energy for use during periods when solar generation is low or absent. This enhances the reliability and efficiency of solar energy systems.
The benefits provided by energy storage solutions are significant. They enable a more stable power supply, reduce reliance on the grid, and can even lead to cost savings on electricity bills. Additionally, they support the overall growth of renewable energy by promoting its use in various sectors, including residential, commercial, and industrial applications.
In the context of this article, energy storage solutions will be explored in depth, focusing on the different battery technologies available, as well as future advancements that will likely emerge in this vital area.
Battery Technologies
There are several battery technologies that play an important role in energy storage for solar applications. These include Lithium-Ion batteries, Lead-Acid batteries, and Flow batteries. Each of these has unique characteristics, implications, and suitability for different contexts.
Lithium-Ion Batteries
Lithium-Ion batteries are among the most effective and popular choices in energy storage for solar systems. They are well-regarded for their high energy density and efficiency in conversion and storage. The key characteristic of these batteries is their ability to charge and discharge at a high rate, making them ideal for handling peaks in energy demand.
One unique feature of Lithium-Ion batteries is their reduced self-discharge rate. This means they can retain their charge for a longer time when not in use, which is beneficial for solar energy systems that generate power intermittently. However, a downside can be their cost, as these batteries generally come with a higher upfront investment compared to other types.
Lead-Acid Batteries
Lead-Acid batteries are a traditional and well-established technology in energy storage. They have been used for many years in various applications, including solar energy systems. The primary characteristic of Lead-Acid batteries is their low initial cost, making them an attractive option for those who may have budget constraints.
A unique feature is their robust performance in deep discharge situations, which can be important for energy storage during extended periods without sunlight. However, their lifespan and efficiency are generally lower than that of Lithium-Ion batteries, making replacements necessary more frequently over time.
Flow Batteries
Flow batteries are considered a newer technology for energy storage. They operate by pumping electrolyte solutions through a cell stack, allowing for scalable energy storage capacity. The main characteristic of flow batteries is their ability to discharge energy over an extended period, which is advantageous for large-scale applications needing sustained energy output.
One major advantage of flow batteries is their long lifespan and durability. They can be cycled thousands of times with minimal degradation. On the downside, the complexity of their system and relatively higher costs can be a barrier for smaller applications.
Future of Energy Storage
Looking ahead, the future of energy storage is promising. Ongoing research and advancements in battery technology aim to improve efficiency, reduce costs, and increase operational lifespan. Innovations such as solid-state batteries and improved energy management systems are set to revolutionize energy storage.
Moreover, as renewable energy integration with smart grid technologies increases, energy storage solutions will play a key role in optimizing energy distribution and consumption.
The future of energy storage solutions is essential for enhancing the potential of solar energy and making it a viable option for a sustainable power supply.
In summary, the landscape of energy storage solutions for solar energy is evolving, with various technologies offering distinct benefits and challenges. Understanding these aspects will aid in making informed decisions about energy storage in relation to solar energy applications.
Economic Implications of Solar Energy
The economic implications of solar energy are multifaceted and play a crucial role in shaping the future of energy production and consumption. Given the growing need for sustainable energy sources, the transition to solar energy not only supports environmental goals but also influences economic factors like job creation, energy prices, and energy independence. Understanding the economic landscape surrounding solar energy is essential for stakeholders ranging from policymakers to investors.
In this context, several significant elements deserve attention. Firstly, solar energy production leads to cost savings for both consumers and utilities. With the declining cost of solar technology, installing solar panels becomes increasingly affordable. This reduction in expenses is beneficial for households as they may see a decrease in their monthly electricity bills. Moreover, as solar energy systems become more prevalent, overall energy prices can stabilize, reducing dependence on fossil fuels.
Secondly, investing in solar energy projects correlates with job creation across various sectors. The solar industry covers fields such as technology development, manufacturing, installation, and maintenance. Each of these sectors requires a skilled workforce, meaning that the growth of solar energy can lead to numerous employment opportunities.
Investing in solar energy not only mitigates environmental impact but also creates a robust economic framework that supports job creation and sustainable growth.
Lastly, the economic implications extend to energy independence. By harnessing solar energy, countries can reduce their reliance on imported fossil fuels, leading to improved national security and stability in energy supply. This aspect is particularly important for countries with limited natural resources who face fluctuating energy prices on the global market.
Cost Analysis of Solar Power Production
To understand the economics of solar energy, a comprehensive cost analysis of solar power production is essential. The analysis covers several aspects, including the initial costs of installation, operational expenses, and long-term savings. The initial investment in solar panels can be significant, but various factors can influence the total cost. The choice between monocrystalline, polycrystalline, or thin-film solar panels also affects the overall expenditure.
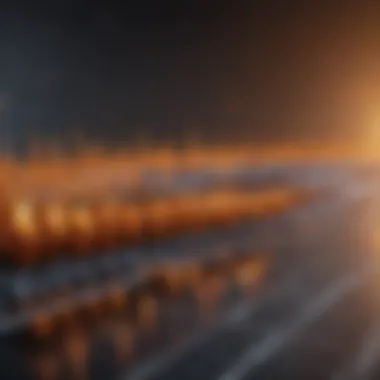
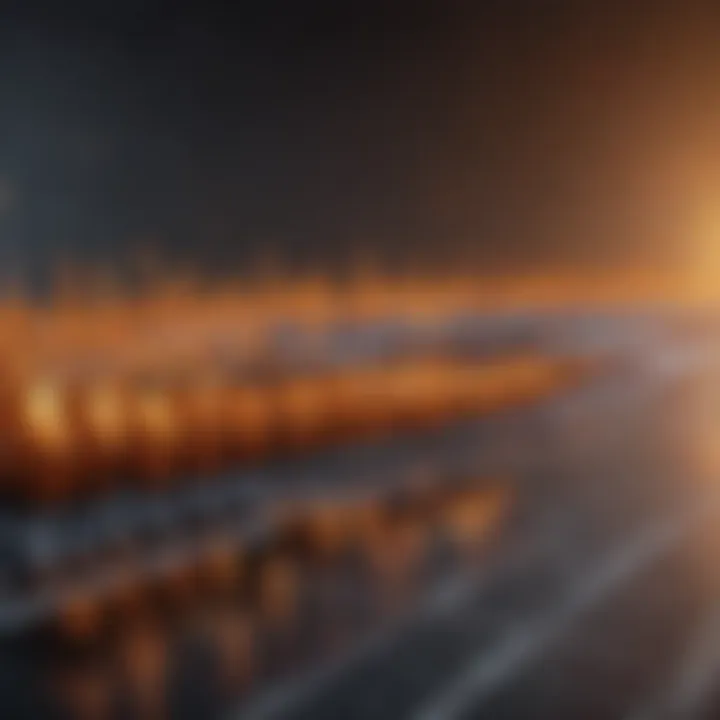
In addition, advances in technology and manufacturing processes have contributed to a steady decline in prices over recent years. According to research, the cost of solar photovoltaic (PV) systems dropped by about 82% between 2010 and 2020, making solar power more accessible to a broader segment of the population. This decreasing trend in costs further underscores the potential for solar energy to contribute to a positive economic model.
Moreover, operational costs are relatively low compared to traditional energy sources. Solar power systems generally require minimal maintenance and have long lifespans, often exceeding 25 years. The lower operational costs help maximize the return on investment over time, allowing consumers and businesses to benefit financially in the long run.
Government Incentives and Subsidies
Government incentives and subsidies play a vital role in promoting solar energy adoption. Various forms of financial assistance, such as tax credits and grants, reduce the burden of upfront costs, making solar energy installations more appealing to potential users.
In many countries, initiatives like the Investment Tax Credit in the United States allow homeowners and businesses to deduct a significant percentage of the installation cost from their federal taxes. Such incentives can dramatically lower the effective cost of solar panel systems, contributing to faster payback periods and enhanced economic viability.
Additionally, state and local programs often complement federal benefits. These programs can take various forms, including rebates for solar installations, performance-based incentives, or renewable energy certificates. These incentives encourage a diverse range of participants to invest in solar technology, further driving down costs and increasing market penetration.
Environmental Impact of Solar Energy
The environmental implications of harnessing solar energy are critical to understanding its role in future energy systems. As societies strive to reduce their reliance on fossil fuels, the integration of solar energy into the energy mix offers significant benefits to the environment. These benefits primarily revolve around lowering greenhouse gas emissions and enhancing sustainability. However, it's equally essential to consider the potential challenges that come with the large-scale adoption of solar technologies.
Reduction of Carbon Footprint
One of the most significant environmental advantages of solar energy is its capacity to reduce carbon emissions. When solar panels convert sunlight into electricity, they do so without releasing harmful byproducts. This starkly contrasts with traditional energy sources like coal or natural gas, which emit a considerable amount of carbon dioxide and other pollutants during combustion.
According to data, solar power can eliminate millions of tons of carbon emissions per year. This reduction is crucial in combating climate change and its effects. In addition, solar energy contributes to a decrease in air pollutants, which can lead to improved public health outcomes. Clean air reduces respiratory diseases and other health issues associated with smog and pollution.
Sustainability and Resource Management
Beyond carbon footprint reduction, solar energy plays a vital role in promoting sustainability. The harnessing of solar power encourages the use of abundant and renewable resources. Unlike fossil fuels, which are finite and deplete over time, solar energy is nearly limitless. Moreover, advancements in solar technologies enable efficient energy capture and usage, minimizing waste.
Efficient resource management is essential for the sustainable deployment of solar energy systems. It involves thoughtful consideration of land use, where large solar farms may compete with agricultural land or natural habitats. Thus, policies promoting dual-use agricultural systems or rooftop solar installations can be encouraged.
In addition, the materials used in solar panel production can have environmental implications. It's essential to focus on recyclable materials and proper disposal methods to minimize the ecological footprint.
"Transitioning to solar energy has not only the potential to mitigate climate change but also encourages a sustainable future for resource management and energy independence."
In summary, the environmental impact of solar energy is profound. With the ongoing advancements in technology, the solar energy sector continues to evolve, paving the way for sustainable energy solutions that align with global climate goals. A comprehensive approach, which includes both reduction of emissions and sustainable resource management, is essential for realizing the full potential of solar energy.
Technological Advancements in Solar Energy
Technological advancements play a crucial role in the evolution of solar energy. They enable more efficient methods of harnessing solar power, thus contributing to increased electricity production and sustainability. Innovations in this domain facilitate the integration of solar technology in various settings, ranging from residential rooftops to large-scale solar farms. Furthermore, advancements in efficiency, reliability, and affordability of materials stimulate wider adoption by consumers and industries alike. Continuous research and development ensure that solar energy remains a viable and competitive option in the energy market.
Innovations in Solar Panel Design
Recent innovations in solar panel design have led to notable improvements in their efficiency and aesthetics. New concepts in solar panel architectures, such as bifacial panels, allow energy capture from both sides of the panel, maximizing sunlight utilization. This design is particularly beneficial in snowy or reflective environments where sunlight can bounce off the ground, providing additional energy without the need for extra panels.
Vertical solar panels, which can be integrated into building designs, serve a dual purpose: they provide building shade and generate electricity. Such innovations avoid the issue of land use while catering to urban environments where space is limited.
Additionally, the development of transparent solar panels is emerging as a flexible solution for integrating solar technology into windows. These panels maintain visibility while capturing sunlight, allowing building owners to produce energy without sacrificing natural light.
Emerging Technologies in Solar Energy Capture
Emerging technologies are essential to improve solar energy capture efficiency. One significant innovation is perovskite solar cells, which have shown great potential in terms of efficiency and manufacturing costs. Perovskite materials can be produced using simpler processes compared to traditional silicon cells, yielding cheaper and more efficient panels.
Moreover, concentrated solar power (CSP) technology enhances energy capture by using mirrors or lenses to focus sunlight onto a small area, producing heat that can generate electricity. This method can be especially effective in regions with high solar insolation, maximizing energy production.
Smart solar trackers also represent a modern trend. These devices follow the sun's movement, ensuring that solar panels remain aligned with direct sunlight throughout the day. This capability can increase energy output significantly, making solar systems more economically viable.
Investing in advanced solar technologies is critical for future energy needs, as they bring together efficiency and cost-effectiveness, leading to broader adoption of solar energy.
In summary, advancements in solar technology not only aim to increase efficiency and power output but also address practical concerns such as space usage and aesthetic integration. As these technologies mature, they solidify solar energy's role as a cornerstone of sustainable electricity production.
Future Trends in Solar Energy Production
As the global energy landscape evolves, the focus on sustainable energy sources has intensified. Solar energy holds a pivotal position in this transition, promising to reshape electricity production in years to come. The trends identified in the solar energy sector reflect advancements in technology and shifting dynamics in energy consumption patterns. Understanding these trends is vital for students, researchers, educators, and professionals alike. This section explores innovations, research avenues, and the integration of solar power with emerging technologies.
Predicted Innovations and Research Directions
Innovations in solar technology are expected to drive significant improvements in efficiency and accessibility. Key areas of research include:
- Bifacial Solar Panels: These panels capture sunlight on both sides, enhancing energy production. The efficiency gains from bifacial technology can be substantial, especially in areas with reflective surfaces.
- Perovskite Solar Cells: Research is ongoing into perovskite materials, which have demonstrated the ability to achieve high efficiency at a lower production cost than traditional silicon panels. This could revolutionize the market.
- Building-Integrated Photovoltaics (BIPV): This concept integrates solar cells directly into building materials. It optimizes space usage while generating energy, making it a practical option for urban environments.
- Advanced Inverters: New inverter technologies are improving grid integration and energy management. Smart inverters facilitate increased solar energy input into the grid, enhancing overall power quality.
Future research directions will likely focus on improving the longevity and performance of existing technologies, as well as optimizing energy storage solutions. Additionally, the compatibility of solar technology with smart devices and grid systems will be an active area of exploration.
Integration with Smart Grid Technologies
Smart grid technologies represent a significant opportunity for enhancing the effectiveness of solar energy systems. Here are considerations regarding this integration:
- Maximized Energy Efficiency: Smart grids facilitate real-time data collection and communication between the energy provider and consumers. This capacity allows for optimized energy distribution, reducing waste and improving efficiency.
- Demand Response Capabilities: With the integration of solar energy, smart grids enable demand response strategies. Consumers can adjust energy usage based on real-time pricing, promoting a more stable energy market.
- Grid Resilience: The decentralized nature of solar energy combined with smart grid technology provides improved resilience against outages and disturbances. This is crucial for maintaining service during extreme weather events.
- Enhanced Storage Solutions: Smart grids support advanced storage solutions like batteries and other technologies that can store excess energy generated during peak solar production. This stored energy can be utilized during periods of high demand when solar generation may be lower.
The synthesis of solar energy with smart grid technologies not only enhances the sustainability of energy resources but also fosters a more responsive and efficient electricity grid. The future holds promise for the continued growth of solar energy production tailored to intelligent energy distribution systems, ensuring a path toward a more secure energy future.
"Innovations in solar energy capture and smart integration will dictate the pace at which we transition to sustainable energy practices."