Advanced Insights into Cryo-EM Equipment and Applications
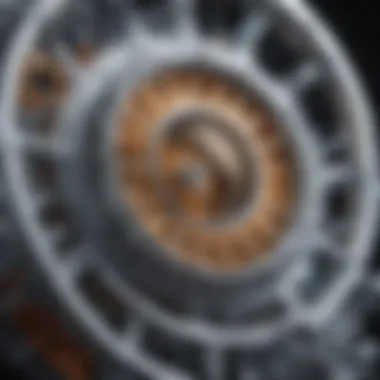
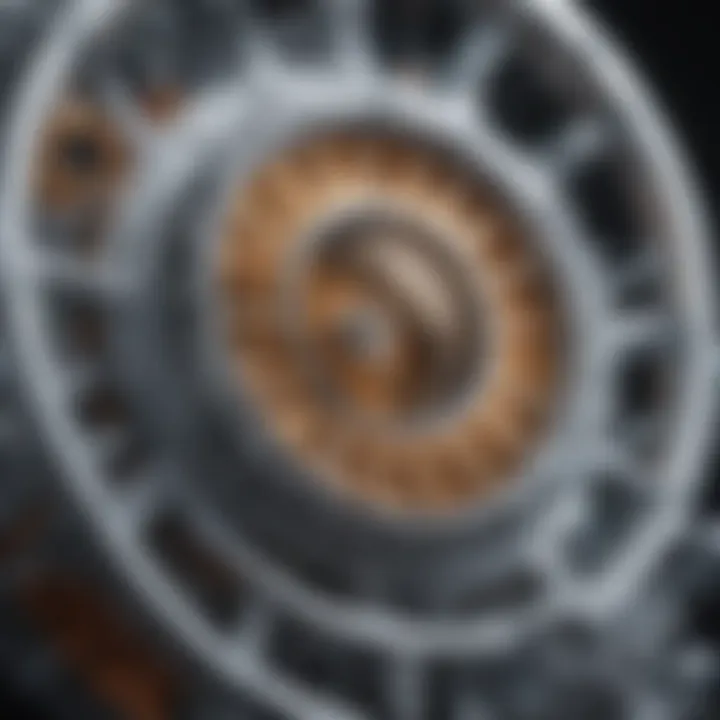
Intro
Cryo-electron microscopy (Cryo-EM) has revolutionized the field of structural biology, allowing scientists to visualize biomolecules at near-atomic resolutions. This technique has a unique capability to capture the native state of samples in a frozen-hydrated form, providing insights that traditional imaging methods often miss. The significance of Cryo-EM equipment goes beyond just the imaging; it encompasses a comprehensive system of components that work together to deliver precise and detailed results.
In this article, we will explore the various aspects of Cryo-EM equipment, including its crucial parts, the mechanisms behind its operation, and the recent advancements that continue to influence its applications in both biological and material sciences. Understanding this intricate apparatus is essential for researchers, educators, and students who aim to harness its potential or enhance their comprehension of contemporary scientific advancements.
Research Highlights
Key Findings
One of the most compelling findings in Cryo-EM technology is its ability to observe protein complexes without the need for crystallization. This allows for a more authentic representation of the biological macromolecules. Recent advances have also shown that even smaller particles can be captured effectively, broadening the scope of what can be studied.
Furthermore, developments in image processing software have drastically improved the fidelity and speed of obtaining structural data, making Cryo-EM a critical tool in drug discovery and disease research.
Implications and Applications
The implications of these findings are vast. As researchers continue to unlock the structures of significant biological entities, the applications extend into various fields:
- Drug Development: Enhancing the understanding of drug targets and proteins involved in disease.
- Vaccine Research: Providing a detailed look at viral structures, aiding in vaccine design.
- Material Science: Offering insights into the structures of new materials at the atomic level, guiding future innovations.
"Cryo-EM stands at the forefront of bridging structural biology and material science, transforming our understanding of complex systems."
Methodology Overview
Research Design
The research design surrounding Cryo-EM typically involves a rigorous approach to sample preparation, imaging, and analysis. The objective is to maintain the integrity of biological samples through each stage of the experimental process. Key aspects include:
- Sample Preparation: Rapid freezing of samples followed by their placement in the electron microscope.
- Data Collection: Use of electrons to generate images, requiring careful calibration and optimization of parameters.
Experimental Procedures
Experimental procedures in Cryo-EM consist of several phases:
- Cryo-Preparation: Samples are flash-frozen using liquid ethane or propane, preserving their natural state.
- Imaging: Advanced microscopes, such as the Titan Krios, capture high-resolution images of the frozen samples.
- Reconstruction: Through software algorithms, the gathered images are processed to recreate 3D structures, providing invaluable insights into molecular architecture.
This methodological rigor ensures that the resulting data is reliable and accurate, crucial for further research and application.
Preface to Cryo-Electron Microscopy
The significance of cryo-electron microscopy (Cryo-EM) in modern scientific research cannot be overstated. This advanced imaging technique allows researchers to visualize biological specimens at near-atomic resolution. It bridges the gap between traditional electron microscopy and the requirement for analyzing delicate biological samples without altering their natural state.
Cryo-EM has emerged as a powerful tool, particularly in the field of structural biology. It has significantly advanced the understanding of complex molecular structures, proteins, and other macromolecules. Through this method, researchers can observe proteins in their functional form and understand how they interact with other molecules. Thus, Cryo-EM plays a crucial role in drug discovery, vaccine development, and protein engineering, further highlighting its relevance in contemporary science.
Historical Context
Cryo-EM has a rich history that dates back to the early developments in electron microscopy. The technique gained momentum in the mid-20th century. Initially, electron microscopy was not capable of capturing images of wet specimens without causing damage. The introduction of cryogenic methods revolutionized the field, allowing for samples to be cooled to liquid nitrogen temperatures. This cooling process preserved the biological structures in their native state, minimizing artifacts caused by traditional preparation methods.
In the 1970s, advancements in electron optics and digital imaging led to the first significant applications of Cryo-EM. Researchers began to produce high-resolution images of viruses and smaller protein complexes. By the 1990s, the method began to gain recognition, culminating in multiple landmark studies that demonstrated its power. The work of pioneering researchers, such as Joachim Frank and Richard Henderson, laid the groundwork for what would eventually lead to the awarding of the Nobel Prize in Chemistry in 2017 for developments in Cryo-EM. Today, this technique continues to evolve, finding applications across various fields beyond biology.
Basic Principles of Cryo-EM
The basic principle behind Cryo-EM involves rapidly freezing biological specimens to preserve their structure. This process usually occurs through a technique called plunge freezing. In plunge freezing, a thin layer of the sample is placed on a grid and then immersed in a cryogen, such as liquid ethane. This rapid cooling prevents the formation of ice crystals, which can disrupt the sample’s integrity.
Once frozen, samples are stored in liquid nitrogen until they are ready for imaging. Cryo-EM uses an electron beam to penetrate the frozen samples, capturing high-resolution images. The resulting images are 2D projections of the 3D structures. Advanced algorithms and automated software enable researchers to reconstruct these images into three-dimensional models.
Overall, Cryo-EM offers vital advantages over other imaging methods such as X-ray crystallography and nuclear magnetic resonance (NMR). Its ability to analyze specimens at high resolutions with minimal sample preparation makes it an invaluable tool in modern research. The technique continues to draw interest for its potential applications in various domains, including drug design and synthetic biology.
Components of Cryo-EM Equipment
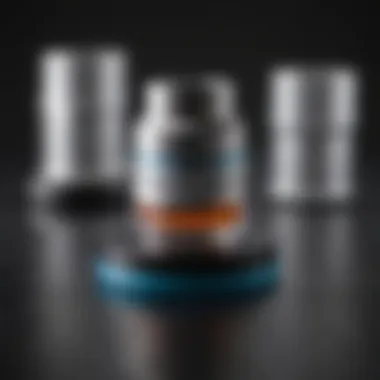
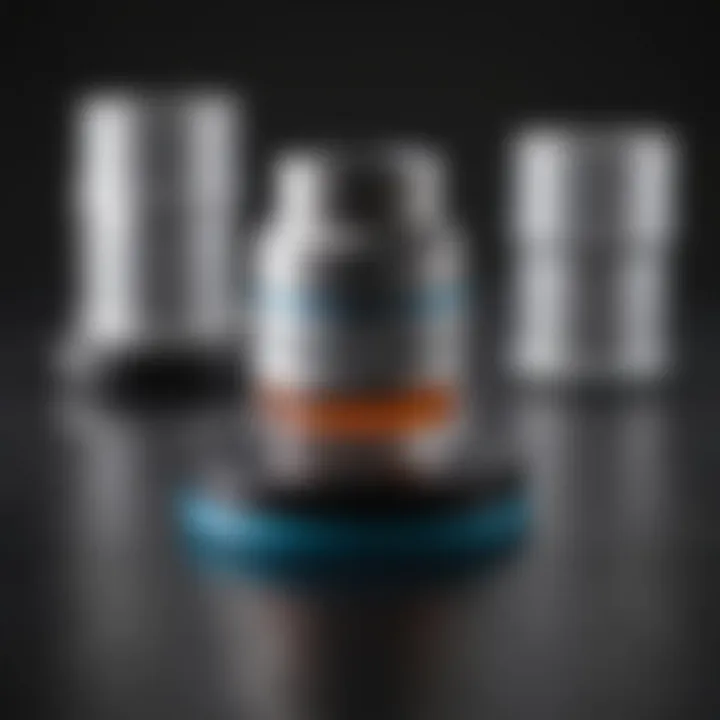
Understanding the components of cryo-electron microscopy (Cryo-EM) equipment is integral to grasping how this advanced technology operates. These elements work in concert to capture high-resolution images of biological specimens in their native states, significantly enhancing research capabilities in various fields such as biology, materials science, and nanotechnology. Each component plays a vital role in the entire process, from sample preparation to data acquisition.
Cryo-holder
The cryo-holder is a crucial component in the Cryo-EM setup. This device is designed to securely hold the frozen samples while maintaining their cryogenic temperature. It ensures that the sample remains in the liquid nitrogen phase, which is essential for preserving the structural integrity of biological materials.
Typically, cryo-holders integrate with the electron microscope. They provide a stable environment to minimize vibration, which could affect imaging quality. The precision of a cryo-holder is determined by its capability to keep the sample below critical temperatures during imaging and to facilitate easy transfer from storage to the microscope. This technology minimizes the risk of thawing and contamination, both critical factors in obtaining reliable results.
Electron Sources
Electron sources generate the electron beams essential to imaging in Cryo-EM. Common sources include thermionic guns and field emission guns. Thermionic guns use heated filaments to produce electrons, while field emission guns create electrons from a sharp metal tip. Both types have unique advantages and limitations, influencing the resolution and contrast of images.
Field emission guns, for instance, offer higher brightness and lower energy spread than their thermionic counterparts. The choice of electron source depends on the imaging needs; higher-quality sources facilitate clearer images, which is vital for structural analysis at the molecular level.
Lenses and Detectors
In Cryo-EM, lenses are engineered specifically to focus electron beams onto the specimen. These lenses are highly sophisticated, often using magnetic fields for precision. The focused electron beam then interacts with the specimen, leading to the emission of secondary electrons and allowing for imaging at an atomic resolution.
Detectors play an equally important role. They capture the emitted electrons and convert them into signals that can be processed into images. Common types of detectors include CCD (charge-coupled devices) and direct electron detectors. Each type has its unique characteristics regarding sensitivity, speed, and resolution, essential factors for effective imaging and analysis.
Vacuum Systems
Vacuum systems are integral for achieving the operational milieu necessary for Cryo-EM. These systems generate a high vacuum to prevent electron scattering caused by air molecules. A properly functioning vacuum is vital for high-resolution imaging.
Most Cryo-EM systems employ turbomolecular pumps and ion pumps to maintain the required vacuum levels. The maintenance of a constant and stable vacuum environment is critical for being able to perform long imaging sessions without compromising data quality.
Sample Preparation Tools
Sample preparation tools are fundamental in the cryo-electron microscopy workflow. These tools include devices for plunge-freezing, freeze-fracturing, and cryo-sectioning. Each method has its own set of procedures and equipment that ensure specimens are prepared correctly for analysis.
Plunge-freezing, for instance, is a common technique where samples are rapidly cooled in liquid ethane to preserve their native structure. Improper preparation can lead to artifacts, thus undermining the quality of the final data. Furthermore, having adequate sample preparation tools ensures that researchers can study a wide variety of specimens, facilitating a more comprehensive analysis of materials.
These components collectively influence the performance, reliability, and applications of Cryo-EM, making their understanding crucial for effective experimentation and research.
Operational Mechanisms of Cryo-EM
Understanding the operational mechanisms of cryo-electron microscopy (Cryo-EM) is vital for those engaged in research and applications of this technology. These mechanisms not only delineate how cryo-EM functions, but also emphasize its practical implications in the fields of biology, materials science, and nanotechnology. Without grasping these concepts, it becomes challenging to appreciate the innovation behind Cryo-EM and the data it produces.
Cryogenics in Sample Preparation
Cryogenics is a critical aspect of sample preparation in Cryo-EM. This process involves rapid freezing of samples using very low temperatures, typically using liquid nitrogen or other cryogenic fluids. The foremost goal is to preserve the sample's native structure and composition without the formation of ice crystals, which can damage delicate biological samples and alter their morphology. When scientists prepare samples, they often utilize a method called plunge-freezing. This method allows the samples to be immersed in a cryogen quickly, thus preventing any significant dehydration or changes to their state.
The successful application of cryogenics hinges on maintaining a temperature around -196 degrees Celsius, crucial for obtaining clear and interpretable images.
Additionally, teams working with cryo-EM must pay attention to the environmental conditions, ensuring a stable and contamination-free atmosphere. Keeping air movement at a minimum is essential to prevent any heat exchange that might compromise the sample integrity.
Data Acquisition Techniques
Data acquisition in Cryo-EM involves capturing high-resolution images of frozen samples while minimizing radiation damage. The radiation emitted from electron sources can lead to sample degradation, thus effective acquisition techniques are necessary. One common approach is to use low-dose imaging strategies. This technique entails taking multiple images at varying exposures, thus reducing damage and allowing for post-processing to enhance resolution.
Moreover, automated data collection systems are increasingly employed to optimize efficiency. These systems can acquire thousands of images in a single session, which are crucial for large samples or projects requiring extensive data.
Researchers also make use of advanced imaging sensors that increase sensitivity and resolution, ensuring that data acquisition leads to quality results. The integration of sophisticated software for indexing and sorting data further enhances the efficiency of the image collection process.
Reconstruction Algorithms
Reconstruction algorithms play an integral role in translating the 2D images captured during data acquisition into 3D models of the sample. The complexity of these algorithms directly impacts the accuracy and reliability of the results. One popular method is the Fourier reconstruction technique, which uses the Fourier transform to manipulate the data collected from multiple 2D projections.
The use of machine learning techniques is on the rise in the development of these algorithms, providing more robust frameworks for analyzing cryo-EM data. These innovations can significantly improve the speed and precision of model reconstructions, making it easier to interpret structural formations at the nanoscale.
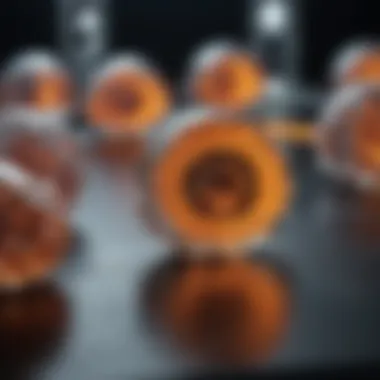
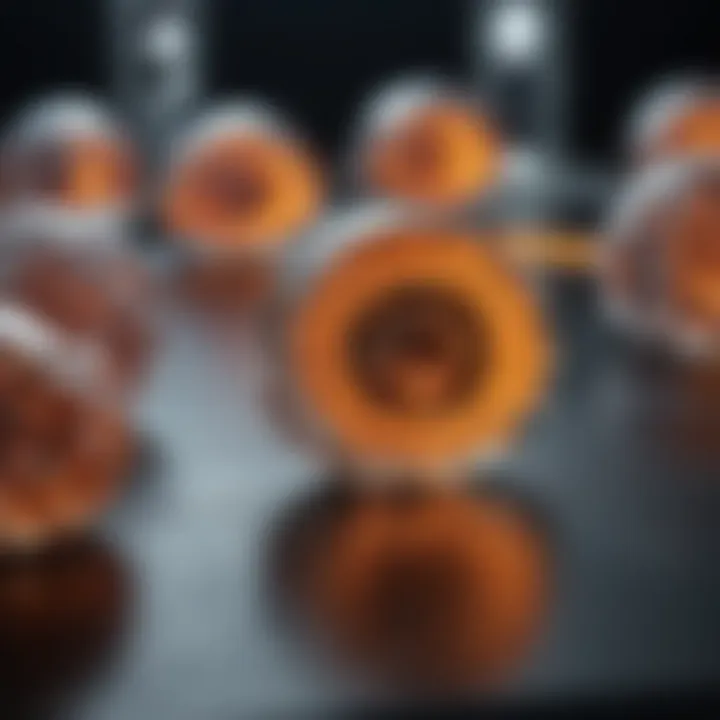
In summary, the operational mechanisms of cryo-EM are the foundation upon which the efficacy and utility of the technique rest. From maintaining cryogenic temperatures during sample preparation to employing effective data acquisition techniques and sophisticated algorithms for reconstruction, each component is critical for achieving the high standards expected in cryo-EM research.
Understanding these aspects allows researchers and practitioners to maximize the insights gained from their investigations and opens avenues for further innovation in the use of cryo-electron microscopy.
Applications of Cryo-EM
Cryo-electron microscopy (Cryo-EM) has emerged as a revolutionary tool, particularly in analyzing complex biological structures and materials at nanoscale resolution. The relevance of this technology is far-reaching, and its applications are crucial in multiple fields. In this section, we will delve into three primary domains where Cryo-EM plays a significant role: structural biology, materials science, and nanotechnology. Each of these areas reveals unique benefits and considerations regarding the use of Cryo-EM, showcasing its versatility and importance in modern science.
Structural Biology
In the realm of structural biology, Cryo-EM has redefined how researchers visualize macromolecular complexes. Traditional techniques such as X-ray crystallography often require crystallization of samples, which can be a limiting factor for certain biomolecules. Cryo-EM circumvents this issue by allowing scientists to observe samples in their native hydrated state, preserving their functional conformation.
One of the notable breakthroughs in structural biology through Cryo-EM includes the determination of the structures of large protein complexes, such as ribosomes and viruses. This capability to visualize large structures at high resolution enhances our understanding of biological processes and interactions. The advent of sophisticated detectors and advanced software has substantially improved image quality and reconstruction algorithms.
"The ability to see proteins and their functions in real-time is a game-changer for molecular biology."
In summary, the application of Cryo-EM in structural biology has not only facilitated significant discoveries but has also opened avenues for drug design and therapeutics. Researchers can now analyze targets for new medicines with greater precision, making Cryo-EM an invaluable component in the toolkit of molecular biology.
Materials Science
Cryo-EM is also making waves in materials science, where it is used to study nanoscale materials and their properties. For instance, the investigation of composite materials and nanostructures has gained momentum thanks to Cryo-EM. This technique allows scientists to visualize the arrangement and organization of nanoparticles within a matrix, providing insights into their behavior and interactions.
The ability to analyze materials at low temperatures preserves their properties and prevents chemical changes that could occur at higher temperatures. For example, researchers can observe phase transitions in a material under different environmental conditions without altering its structure. This perspective is vital for developing new materials with specific characteristics, such as strength or conductivity.
Furthermore, Cryo-EM can assist in understanding defects and dislocations in materials, which are critical for assessing material performance and reliability. As industries demand more efficient and reliable materials, the use of Cryo-EM will continue to grow, aligning with technological needs in areas such as electronics and construction.
Nanotechnology
The role of Cryo-EM in nanotechnology cannot be overstated. As the field focuses on the manipulation and characterization of materials at the atomic or molecular scale, the ability to visualize these structures is paramount. Cryo-EM provides detailed imaging of nanomaterials such as carbon nanotubes, graphene, and nanoparticles, helping researchers explore new properties and functionalities.
Moreover, with the increasing interest in nanomedicine, Cryo-EM's applications are expanding. Understanding how nanocarriers interact with biological systems can significantly influence drug delivery systems. Observing how nanoparticles aggregate or separate in biological fluids aids in designing targeted therapies.
Cryo-EM also enables the assessment of the stability and interactions of nanoscale materials under physiological conditions, providing insights into their potential applications in diagnostics or treatment.
Recent Advancements in Cryo-EM
The field of cryo-electron microscopy (Cryo-EM) is evolving rapidly, driven by technological innovations and interdisciplinary collaborations. Recent advancements have expanded the capabilities of Cryo-EM, making it an indispensable tool in structural biology and materials science. This section will delve into the integration of artificial intelligence in Cryo-EM and the development of novel image processing techniques, highlighting how these advancements enhance overall performance and data quality.
Cryo-EM and Artificial Intelligence
Artificial intelligence has emerged as a transformative force in various scientific fields, and Cryo-EM is no exception. Machine learning algorithms improve data acquisition and image reconstruction. These technologies streamline the analysis of vast datasets produced by Cryo-EM, which can be overwhelming due to their size and complexity.
Key benefits of integrating AI into Cryo-EM include:
- Automated Particle Picking: AI can identify and select particles from noisy images more efficiently than traditional methods. This reduces the time researchers spend on manual selection, allowing for faster processing.
- Enhanced Resolution: Advanced algorithms enable improved image reconstructions, leading to higher resolution structures. This is critical for understanding biological macromolecules and their functions in greater detail.
- Noise Reduction: AI techniques, such as convolutional neural networks, can effectively remove background noise from images. The result is clearer, more interpretable data, which leads to better scientific insight.
Using AI in Cryo-EM not only enhances workflow efficiency but also opens new avenues for research. As the technology develops, one can expect even greater improvements in resolution and accuracy—key attributes for scientists aiming to unravel the mysteries of molecular structures.
Novel Techniques in Image Processing
The image processing techniques in Cryo-EM are also undergoing significant innovation. These advancements are essential for converting raw data into meaningful three-dimensional models. New algorithms and software tools are facilitating this transformation, emphasizing both speed and accuracy in processing.
Some notable advancements include:
- Deep Learning Approaches: These involve training models to recognize patterns in Cryo-EM data. They provide improved object recognition, allowing for more precise alignments and reconstruction.
- Real-Time Processing: Recent developments enable near real-time processing of data during collection. This capability allows researchers to quickly evaluate the quality of the data, making real-time adjustments as needed.
- Focus on User Accessibility: Many of the latest software platforms are designed to be user-friendly. Intuitive interfaces simplify complex workflows, making advanced image processing accessible to a broader audience, including those who may not have extensive computational training.
These techniques vastly improve the efficiency and effectiveness of the data analysis pipeline in Cryo-EM, enabling researchers to leverage their findings significantly.
Recent advancements in Cryo-EM reflect the continuous quest for excellence in imaging technologies, overcoming limitations of previous methods and redefining possibilities in scientific exploration.
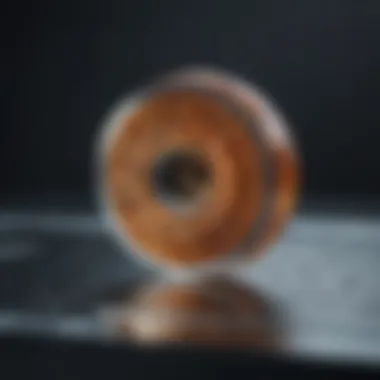
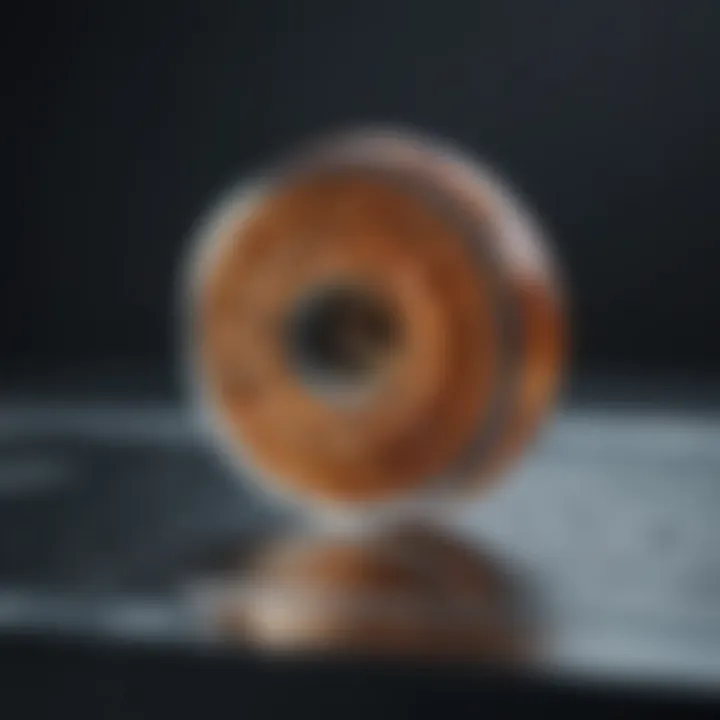
Together, these advancements in artificial intelligence and image processing are not only enhancing the quality of Cryo-EM data but also paving the way for new applications in various scientific domains. The research community remains keenly focused on exploiting these developments, promising a vibrant future for Cryo-EM.
Challenges and Limitations
Understanding the challenges and limitations of cryo-electron microscopy (Cryo-EM) is crucial for researchers who wish to harness its potential. While Cryo-EM has revolutionized structural biology and material sciences, it does not come without its hurdles. The successful application of Cryo-EM depends on overcoming these barriers, enhancing data interpretation, and ensuring the reliable generation of high-quality images. This section dives into specific technical challenges associated with Cryo-EM equipment, as well as the complexities in data interpretation, shedding light on factors that can limit its effectiveness.
Technical Challenges
Cryo-EM, although powerful, presents several technical challenges that affect imaging capability. These challenges might stem from the equipment itself or the processes involved in sample preparation. Some common technical issues include:
- Sample Preparation: The process of preserving biological samples in a frozen state can introduce artifacts. Maintaining structural fidelity during rapid freezing is vital. If procedures are not executed correctly, samples may suffer from distortion.
- Vacuum Systems: The electron beam used in Cryo-EM must travel through a high-quality vacuum. Any imperfections in the vacuum system can lead to scattering of particles and ultimately compromise image clarity.
- Defocusing and Aberrations: Maintaining a precise focus is essential in Cryo-EM. Defocus can blur images, leading to difficulties in assembling accurate 3D reconstructions. Aberrations introduced by lenses further impact image quality.
- Beam Damage: Samples in their native environment can be sensitive to electron irradiation. Extended exposure to the electron beam can lead to degradation, altering the images captured.
Addressing these challenges often requires optimization of equipment and meticulous tuning of the operational parameters. Continuous innovation in cryo-EM techniques helps mitigate many of these issues, but they still pose significant considerations for users.
Interpretation of Data
The interpretation of data generated from Cryo-EM is another area fraught with complexity. As the field evolves and scientists increasingly rely on intricate models, the nuances of data analysis become paramount.
- Noise Reduction: Images captured can be noisy, with a low signal-to-noise ratio, making it necessary to develop advanced techniques for noise reduction. The presence of noise complicates data refinement and analysis.
- Software Limitations: The tools used for reconstructing 3D models from 2D images encounter limitations. Existing software can sometimes fail to accurately reconstruct the true structure, influencing the resulting models.
- Biological Variability: Biological samples can vary significantly, even among closely related structures. This variability makes it difficult to draw definitive conclusions about structural details.
Ultimately, the successful interpretation of Cryo-EM data requires a combination of robust analytical skills and deep understanding of the biological context. Scientists must remain aware of the inherent limitations when evaluating results and making inferences.
"The true utility of cryo-EM lies not only in capturing stunning images but also in the careful interpretation of these structures, understanding their context within biochemical pathways."
In summary, recognizing both the technical challenges and the intricacies involved in data interpretation is vital for advancing the application of Cryo-EM in scientific research. These considerations will guide researchers in optimizing their approaches, leading to more effective utilization of Cryo-EM technology.
Future Directions in Cryo-EM Research
Future directions in Cryo-Electron Microscopy (Cryo-EM) research hold significant promise for advancing a multitude of scientific fields. Given the increasing necessity for high-resolution structural data in structural biology, materials science, and nanotechnology, researchers are exploring innovative methodologies and applications that can enhance the efficiency and outcomes of Cryo-EM studies. This section examines emerging technologies and the potential applications that may shape the future of Cryo-EM.
Emerging Technologies
Emerging technologies within Cryo-EM are paving the way for more refined and effective imaging capabilities. Some noteworthy advances include:
- Automation: Modern Cryo-EM setups are increasingly integrating automation for sample handling and data collection, reducing the time requirements and human error.
- Laser Systems: Enhanced lasers are being utilized to improve the imaging contrast and resolution, enabling clearer visualization of biological specimens in their near-native states.
- High-Throughput Screening: New technologies are allowing for high-throughput imaging, which can dramatically speed up the data acquisition process, thus enabling larger sample sets to be analyzed in shorter timeframes.
These developments are crucial in reducing the turnaround times for structural determination and increasing the throughput of the research process.
Potential Applications
The applications of advanced Cryo-EM technologies are expansive and varied, influencing several domains of research and industry. Some potential applications include:
- Drug Development: Cryo-EM can facilitate the visualization of drug targets at an atomic level, streamlining the drug discovery process by offering insights into protein structures and interactions.
- Vaccine Research: High-resolution imaging of viral particles can enhance vaccine formulations, contributing to rapid advancements in infectious disease preparedness and response.
- Materials Characterization: In materials science, Cryo-EM can aid in understanding the micro- and nanostructures of novel materials, bridging the gap between theoretical research and practical applications.
These applications may significantly impact real-world challenges, making Cryo-EM a pivotal player in both academic and industrial research arenas.
"The evolution of Cryo-EM is not just about better imaging; it’s about unlocking the mysteries of matter at the atomic scale, ultimately driving innovation across diverse fields."
As these technologies and applications develop, researchers and professionals in the field must stay abreast of these changes to harness the full potential of Cryo-EM in future discoveries.
Finale
In this comprehensive guide, we explored the critical landscape of cryo-electron microscopy (Cryo-EM) equipment. The significance of Cryo-EM in a variety of scientific fields has become increasingly apparent. The technology's ability to visualize biological samples at near-atomic resolution allows for unprecedented insights into molecular structures and mechanisms. This capability enhances our understanding in structural biology, materials science, and nanotechnology, spurring innovations and driving forward multiple disciplines.
Summary of Key Points
Key aspects highlighted throughout the text include:
- Components of Cryo-EM: The importance of individual parts, such as cryo-holders, vacuum systems, and electron sources, in ensuring optimal performance and results.
- Operational Mechanisms: Techniques involved in sample preparation and data acquisition, which are essential for high-quality imaging.
- Applications and Advancements: Overview of how Cryo-EM is employed across various fields and the recent developments that are revolutionizing its scope.
- Challenges: Understanding the limitations faced in this technology, including technical challenges and the interpretation of complex data sets.
- Future Directions: Potential emerging technologies that could enhance Cryo-EM capabilities and broaden its application range.
The Importance of Cryo-EM in Modern Science
Cryo-electron microscopy is not just a tool; it is a cornerstone of modern scientific inquiry. The implications of advancements in Cryo-EM resonate beyond structural understanding. For instance, in the fight against diseases, Cryo-EM plays a role in drug design, helping to elucidate the structure of viral proteins, which can lead to the development of novel therapeutics.
Moreover, the precision offered by this technology significantly contributes to materials science. Understanding materials at the nanoscale can lead to breakthroughs in electronic devices, catalysis, and nanocomposites. The interplay between Cryo-EM and artificial intelligence presents further opportunities for data analysis that push the boundaries of what can be achieved.
As science continues to evolve, Cryo-EM remains at the forefront, providing vital insights that can shape the future of research and development. Its multifaceted relevance underscores not only its technical capabilities but also its profound influence on scientific progress.