The Evolution and Applications of Photon Technology
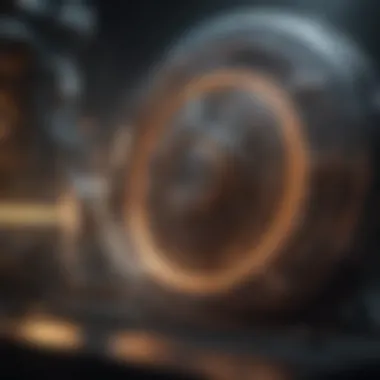
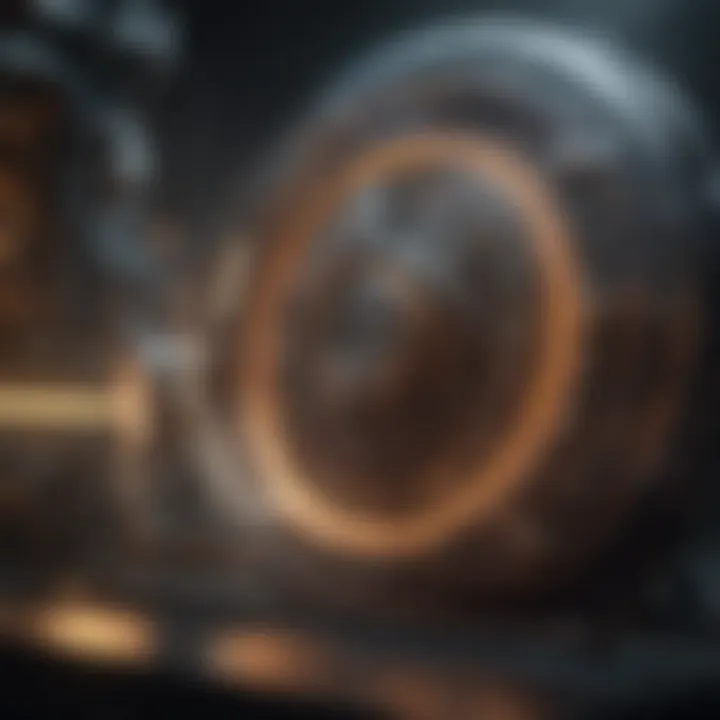
Intro
Photon technology is rapidly becoming a cornerstone in various scientific fields, intertwining basic principles with groundbreaking applications. As we delve into the essence of this evolving domain, we find that understanding the evolution of photon-related innovations opens up a treasure trove of insights into the modern world.
Fundamentally, photons are elementary particles representing a quantum of light or other electromagnetic radiation. Their behavior is governed by the principles of quantum mechanics, which reveal not just the nature of light, but also how we can manipulate it for our advantage. From telecommunications, where information travels at the speed of light, to sophisticated medical applications such as phototherapy, the myriad of uses for photon technology is staggering. As we traverse this landscape, it helps to grasp the historical context, the evolution of technology, and the implications of using photons in our daily lives.
Research Highlights
Key Findings
- Historical Context: The roots of photon technology can be traced back to early studies in optics and light behavior. Notable figures like Isaac Newton and Max Planck laid the groundwork with their pioneering work on light.
- Technological Advancements: Over the decades, advancements in laser technology and fiber optics have transformed the way we use light. The invention of the laser in the 1960s, for example, catalyzed a surge in applications, particularly in telecommunications and medical fields.
- Current Applications: Today, photon technology permeates various domains, including:
- Future Directions: As research deepens, we see potential in areas such as quantum computing and sustainable energy solutions.
- Telecommunications: Fiber optics rely on light to transmit data over great distances, revolutionizing how we communicate.
- Medicine: Advanced techniques using lasers are now commonplace in surgeries and treatments for conditions such as eye disorders.
- Research: High-energy photon beams are used in particle physics experiments, shedding light on the fundamental particles that constitute our universe.
Implications and Applications
The applications of photon technology extend beyond mere convenience; they reshape our understanding of efficiency and capability in various fields. The implications are profound:
- Enhanced communication speeds facilitate global connectivity.
- Medical technologies improve treatment efficacy and patient outcomes.
- Environmental sustainability may see breakthroughs through innovative photon applications in photovoltaic cells.
"Photon technology not only pushes the boundaries of what is possible but also redefines how we engage with the universe."
Methodology Overview
Research Design
The exploration of photon technology typically involves a combination of experimental physics, engineering practices, and theoretical frameworks. Researchers often utilize interdisciplinary approaches to synthesize findings from various fields.
Experimental Procedures
Experiments often include using lasers in controlled environments to study photon behavior. For example:
- Setups that measure the efficiency of energy transfer in laser applications.
- Investigations on photon entanglement phenomena to develop quantum technologies.
Preface to Photon Technology
Photon technology represents a cornerstone of modern science and engineering, fundamentally transforming how we perceive and interact with light. It serves as a bridge connecting various fields, from telecommunications to medicine. Understanding photons is not just an academic exercise—it has real-world implications that affect technology, environmental science, and communication practices. This section aims to illuminate the nuances surrounding photon technology, presenting it as a pivotal component in the tapestry of contemporary innovation.
Defining Photons
Photons are the elementary particles of light. They are massless and carry energy, moving at the speed of light in a vacuum. Their behavior can be somewhat counterintuitive; for instance, they exhibit properties of both particles and waves. This duality is a core concept in quantum mechanics, underscoring the complexities of photon interactions. Essentially, one can think of photons as tiny packets of energy that can influence a vast range of physical phenomena.
To get a bit more technical, the energy of a photon is directly related to its frequency, which can be described with the formula:
[ E = h u ]
Where represents the energy, is Planck's constant, and is the frequency of the light wave. This relationship is crucial for understanding how photons interact with matter. It also sets the stage for various applications in fields such as optical communications, where the modulation of light can transmit vast data streams.
Historical Context
The journey to understand and harness photons stretches back to ancient civilizations that harnessed sunlight. However, in the scientific realm, significant strides began with Sir Isaac Newton and later, Albert Einstein. Newton proposed a corpuscular theory of light, while Einstein's work on the photoelectric effect in 1905 eventually earned him the Nobel Prize in Physics. His assertion that light can be quantized into photons laid the groundwork for modern quantum physics.
As the 20th century unfolded, various technologies focusing on photon utilization began to emerge. The advent of the laser in 1960 by Theodore Maiman marked a watershed moment. Suddenly, applications that used to be confined to theoretical possibilities were now practical realities, from telecommunications to advanced medical devices.
Importance in Modern Science
Photon technology has become a linchpin for numerous fields of study and practical applications. In telecommunications, fiber optic communication relies heavily on the principles of light. It allows for rapid data transfer over vast distances, surpassing traditional methods like copper wiring both in speed and capacity. This advancement has Columbia Technology in the communication sector, enabling everything from international calls to high-speed internet.
In the medical realm, photon technology has revolutionized diagnostics and treatment methods. Techniques like MRI and PET scans utilize photons to create detailed images of the body, which in turn enhance diagnosis and therapeutic strategies. This kind of imaging has been a cornerstone of modern healthcare, enabling lighter, more accurate, and less invasive procedures.
Ultimately, the evolution of photon technology underscores not just a technical advancement but a broader societal shift toward an increasingly interconnected world. Both theoretical explorations and practical applications continue to advance, raising important questions about the ethical implications and potential future developments in photon research. Understanding these layers is essential for students, researchers, educators, and professionals alike, as they navigate the complexities of this dynamic field.
"The most exciting breakthroughs of the 21st century will happen at the intersection of biology and technology." — Steve Jobs
This exploration sets the stage for the subsequent discussions in the article, which dive deeper into the fundamentals of photon physics and their applications across various domains.
Fundamentals of Photon Physics
Photon physics forms the bedrock of our understanding of how light behaves and interacts with matter. Its significance in the broader narrative of photon technology cannot be overstated. By grasping the fundamentals, one can truly appreciate the mechanics and implications of advanced applications ranging from telecommunications to medical imaging.
Quantum Theory of Light
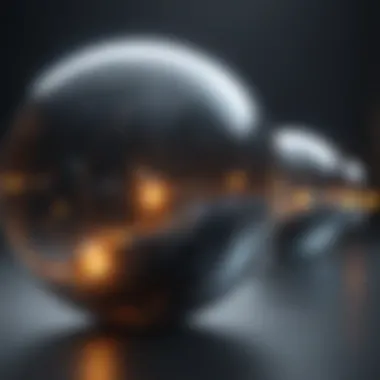
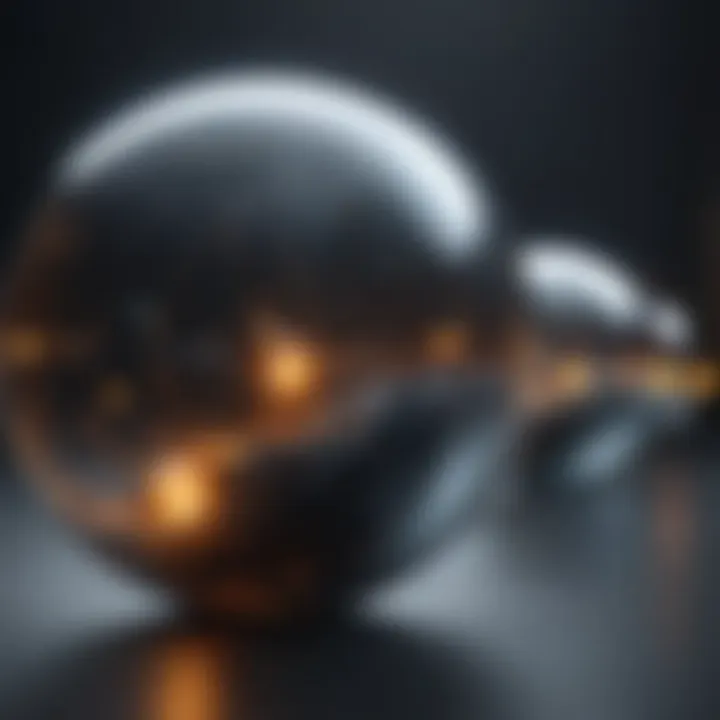
At its core, the quantum theory of light describes how photons behave not just as particles, but also as waves. This dual nature is key to many technologies we rely upon today. For instance, when considering lasers, understanding their quantum principles is essential for creating coherent light beams, which pack a punch in both power and precision. Such insights are not only foundational but also pave the way for innovations in various fields, emphasizing the need for a thorough comprehension of these principles in any serious discourse on photon technology.
Properties of Photons
Energy and Frequency
Exploring the energy and frequency of photons gives us insights into their behavior and applications. This aspect stands out for its direct influence on how we utilize light energy. Each photon carries energy directly proportional to its frequency, which is tantamount to saying that higher frequency photons, like ultraviolet light, pack more energy than their lower frequency counterparts, such as infrared light. In practical terms, this means that specific applications can be designed based on the desired energy absorption capabilities of materials, making understanding this relationship a benefit for materials science and photonics alike.
Wavelength and Color
The wavelength of a photon is intricately linked to the color of light we see. It's not just a trivial detail; the attributes of light shift dramatically with variations in wavelength. For example, different wavelengths give rise to different colors in the visible spectrum. This facet is particularly beneficial for applications in optics and colorimetry, as precise control of wavelengths can lead to more effective sensors and imaging techniques. However, one must also keep in mind that while short wavelengths can offer higher resolution, they also come with the drawback of being absorbed more readily by many materials, sometimes limiting their practical application.
Behaviors in Different Media
Light's behavior can change drastically depending on the medium it's traversing. For instance, light bends when it moves from air into glass—a phenomenon known as refraction. This behavior is crucial for designing lenses used in a multitude of devices, from cameras to corrective eyewear. Moreover, understanding these interactions can lead to the improvement of optical coatings and filters, enhancing overall performance across various applications. However, there's a nuanced challenge as well; differing media can lead to varying degrees of dispersion, which can complicate optical systems if not carefully managed.
Photon Interaction with Matter
Absorption and Emission
Absorption and emission of photons are fundamental processes that govern interactions between light and matter. When light hits an atom, it can be absorbed, causing an electron to jump to a higher energy level. This principle underpins diverse applications, particularly in spectroscopy, where knowledge of absorption spectra allows scientists to infer atomic and molecular characteristics. However, there's a balance to strike; excessive absorption can lead to loss of energy and limitations in signal strength in various applications.
Refraction and Reflection
Refraction and reflection represent two sides of the same coin. When light hits a boundary between two different media, some of it bounces back (reflection), while some continue through with a change in speed and direction (refraction). Understanding this duality helps in various fields, from designing optical instruments to creating effective safety signage using reflective materials. One downside is that these phenomena can introduce distortions, especially at extreme angles or with more complex surfaces, which researchers often need to mitigate.
Photon Entanglement
Photon entanglement is a baffling yet intriguing phenomenon where pairs or groups of photons become interconnected in ways that defy classical physics. This characteristic holds incredible potential, particularly in quantum computing and secure communications. The peculiar benefit lies in the instant correlation between entangled photons, regardless of distance, paving pathways for innovations such as quantum networks. That said, producing and maintaining entangled states comes with substantial technical challenges, requiring highly controlled environments to ensure stability.
"The exploration of photon physics not only deepens our understanding of light but also illuminates a myriad of possibilities in advancing technology."
By comprehending these fundamental principles, we lay a solid foundation for discussing the advancements and applications that follow. Each element plays a pivotal role, shaping how we harness the power of photons to push boundaries in science and technology.
Technological Advancements in Photon Technology
Photon technology has come a long way, and the advancements in this field are truly transformative. It plays a crucial role in various cutting-edge applications, impacting everything from communication systems to medical treatments. Understanding these advancements not only sheds light on how we harness light but also emphasizes the importance of ongoing research and development in this area.
Lasers and Their Applications
Medical Uses
The medical arena has seen significant benefits from laser technology. Laser treatments are commonly used in surgeries, dermatology, and optometry. One of the standout characteristics of medical lasers is their precision. They allow for targeted treatments with minimal damage to surrounding tissues. This means shorter recovery times and less pain for patients, making laser procedures a popular choice.
A unique feature of these medical applications is the ability to customize laser wavelengths for specific treatments. For instance, the use of different types of lasers like the CO2 or Nd:YAG laser offers varied depths of penetration and tissue interaction. However, one should also consider the cost implications and the need for specialized training for practitioners, which can be barriers in some contexts.
Industrial Applications
In the industrial sector, lasers serve multiple purposes, from cutting and welding to engraving materials. The high energy density of laser beams allows for precise cuts that reduce waste and enhance productivity. This precision is invaluable, especially in industries where tolerances are tight, like aerospace and automotive manufacturing.
One prominent aspect of industrial lasers is their adaptability to various materials. Whether it's metals, plastics, or even ceramics, lasers can efficiently work with diverse substrates. However, the initial cost of laser machinery can be significant, which might discourage smaller businesses from investing.
Scientific Research
Lasers are indispensable in scientific research, particularly in fields like physics and chemistry. They enable researchers to conduct experiments with accuracy that wouldn't be possible with traditional methods. The ability to manipulate laser light at ultra-fast speeds has opened up new avenues for exploring phenomena at the atomic scale.
A key benefit of using lasers in research is the non-invasive nature of their applications. Researchers can study living cells without altering their natural state. However, there's a downside—access to high-quality laser equipment can be limited by funding and resource availability, potentially slowing down research progress.
Photonics in Communication
Optical Fiber Technology
Optical fiber technology represents a pinnacle of advancement in telecommunications. This technology utilizes light to transmit data, enabling speeds far surpassing traditional copper wiring. The very properties of light, such as its speed, contribute to quicker and more efficient data transfer.
What makes optical fiber particularly beneficial is its capacity for vast amounts of bandwidth, supporting the demands of modern high-speed Internet. Nevertheless, it comes with challenges, such as installation costs and the complexity of the technology, which can deter widespread adoption in certain areas.
Data Transmission
The method of data transmission through photonic systems has revolutionized how information is shared globally. By encoding data as pulses of light, systems can achieve incredible speeds with lower power consumption compared to conventional electrical transmission.
A noteworthy characteristic is the reduced signal degradation over long distances when using light. This characteristic allows for extended ranges without the need for boosting signals repeatedly. However, the complexity of maintaining these systems can pose challenges, particularly in areas without proper infrastructure.
Future Trends in Telecommunication
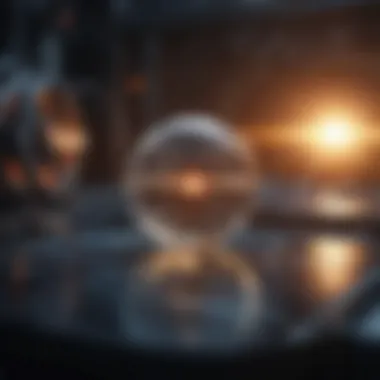
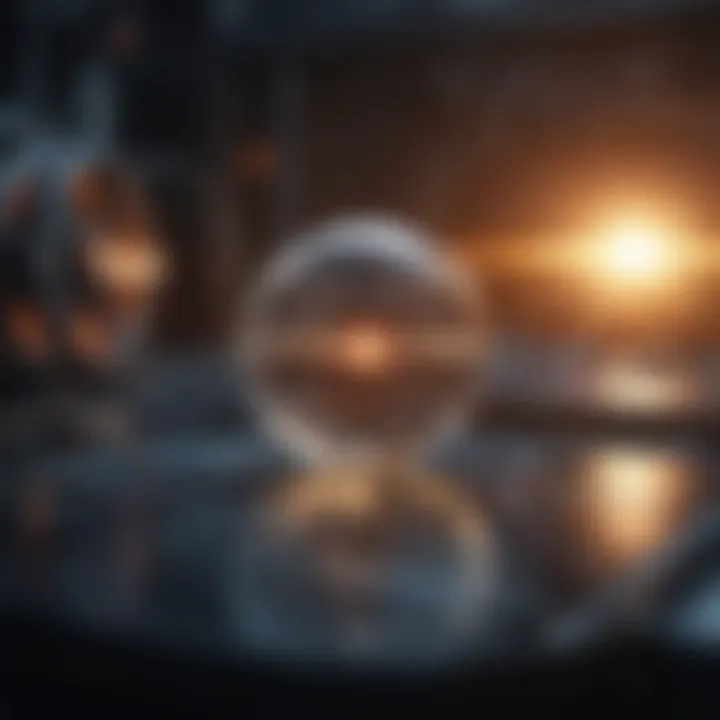
As we look to the future of telecommunication, emerging trends in photon technology are poised to change the landscape drastically. Innovations such as quantum communication suggest potential for even faster and more secure data transfer methods.
The emphasis on sustainability also drives trends, with a push for energy-efficient systems that can handle the increasing data demands safely. Still, the challenges are many—including regulatory hurdles and the need for significant upgrades to existing infrastructure to enable these advancements.
Photon Technology in Imaging
Medical Imaging Techniques
Photon technology has transformed medical imaging, making it more accurate and less invasive than ever before. Techniques like MRI, CT scans, and PET scans rely heavily on photons for capturing detailed internal images of the body. The fundamental characteristic of these imaging modalities is their ability to provide real-time data.
The unique feature of photon-based imaging is the non-invasive nature of the procedures, allowing practitioners to assess conditions without surgical intervention. Yet, high costs and exposure to ionizing radiation in some techniques pose potential risks to patients and further emphasize the need for ongoing innovation and safety improvements.
Environmental Monitoring
In environmental monitoring, photon technology plays a key role in assessing air and water quality. Tools like spectrometers use light to analyze substances, providing accurate detection of pollutants. This aspect is essential for understanding environmental health.
The capacity of photonic systems to monitor large areas quickly makes them indispensable. However, field deployment can be challenging, requiring calibration and maintenance, which adds to operational costs.
Satellite Imaging
Satellite imaging has been transformed through advances in photon technology, offering detailed and timely insights into Earth’s landscapes. Satellites equipped with photon-based sensors can capture high-resolution images over vast areas, assisting with tasks from urban planning to disaster recovery.
The compelling aspect of satellite imaging is its ability to provide continuous data without physical presence in the observed location. However, reliance on weather conditions can pose limitations. Cloud cover, for instance, can hinder image quality, necessitating backup systems or alternatives for critical analysis.
Each of these advancements highlights the multifaceted nature of photon technology today. Its contributions span diverse fields, punctuating the importance of ongoing investment in research and development to overcome challenges and unlock future potential.
Applications Beyond Traditional Fields
The realm of photon technology has seeped into areas beyond its traditional applications, enhancing our understanding and capabilities in various modern sectors. Such applications not only demonstrate the versatility of photons but also show how they can address current challenges and future needs.
Photon technology is not just a tool; it is a linchpin connecting industries and innovation. As we delve into this section, we will explore significant developments in energy generation, quantum computing, and material science. Each of these areas harnesses the properties of photons in unique ways, ultimately leading to advancements that offer both functionality and sustainability.
Photon Technology in Energy Generation
Solar Energy
Solar energy is the darling of renewable resources. It's harnessed using photovoltaic panels that convert sunlight directly into electricity. The key characteristic of solar energy is its sustainability and availability, making it an attractive choice for a greener planet. In essence, this technology promises not just reduced carbon footprints, but a step towards energy independence. A unique feature of solar energy is its modular nature, allowing easy scalability from small residential setups to extensive solar farms. Nevertheless, it does have disadvantages, like dependence on weather and the current reliability challenges in energy storage.
Photovoltaic Cells
Photovoltaic cells represent the driving force behind solar technology. These cells facilitate the conversion of photons into usable electrical energy. They are incredibly popular in today's market due to their efficiency and decreasing production costs. The distinctive feature of photovoltaic cells is their ability to operate silently and without emissions, which aligns perfectly with modern eco-friendly aspirations. However, a downside remains in terms of the raw materials used, which can have environmental repercussions in their extraction and processing.
Potential for Future Energy Solutions
The potential for future energy solutions through photon technology is dazzling. Ongoing research continually strives for improved efficiency and energy storage mechanisms. A highlighted characteristic is its focus on innovative methods of energy capture, aiming to encompass not just sunlight but other light sources. This adaptability makes it a beneficial choice for diversifying energy portfolios. Unique features exist with proposals for integrating solar technologies into building materials, which could significantly change energy consumption in urban settings. But caution is necessary; the challenges of infrastructure overhauls and installation costs can deter widespread adoption.
Role in Quantum Computing
Basic Principles of Quantum Computing
Quantum computing rests upon the principles of superposition and entanglement. It shifts our understanding of computation by creating a new era of processing capabilities. These principles enable quantum bits or qubits to represent multiple states at once, offering immense potential for tasks beyond the reach of traditional computers. This paradigm is a game-changer, especially in fields like cryptography and complex system modeling.
Photonic Quantum Bits
Photonic quantum bits stand at the intersection of quantum computing and photonics, uniquely utilizing light to perform computations. They are unparalleled for their speed and efficiency, significantly reducing the heat generation common in classical computing systems. A strong feature of photonic qubits is their ability to transmit data over long distances with minimal loss. Yet they face challenges, such as the ability to maintain coherence during operations, which is crucial for successful computation.
Advantages Over Conventional Systems
Opting for quantum computing through photonics brings forth several advantages. Photon-based computation operates at room temperature, whereas traditional systems often rely on complex cooling methods. Additionally, the high-speed data transfer facilitated by light can revolutionize industries reliant on fast data processing. Nonetheless, transitioning from conventional systems to quantum ones poses its challenges, particularly in terms of cost and expertise required in this nascent field.
Innovations in Material Science
Photonic Crystals
Photonic crystals represent a remarkable leap in material science, manipulating light at the micro and nanoscale. Their ability to control the flow of photons opens doors for advancements in display technology and telecommunications. A key characteristic is their capacity to create band gaps, allowing particular wavelengths of light to be reflected or transmitted selectively. While they are a popular choice for developing innovative optical components, the complexity of manufacturing them remains a hurdle.
Metamaterials
Metamaterials stretch beyond conventional limits of optics by allowing unique properties not typically found in nature, such as negative refractive index. They can bend light around objects, leading to potential applications in invisibility cloaks and superlenses. Their distinctive feature lies in their engineered structure rather than their composition. However, crafting metamaterials remains costly and time-intensive, posing challenges for quick commercial adaptation.
Applications in Nanotechnology
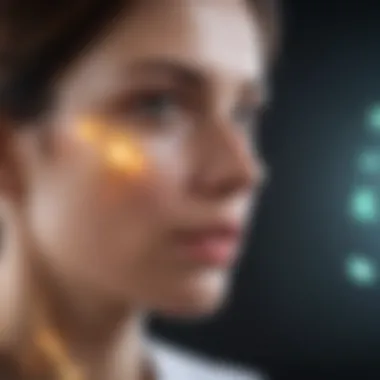
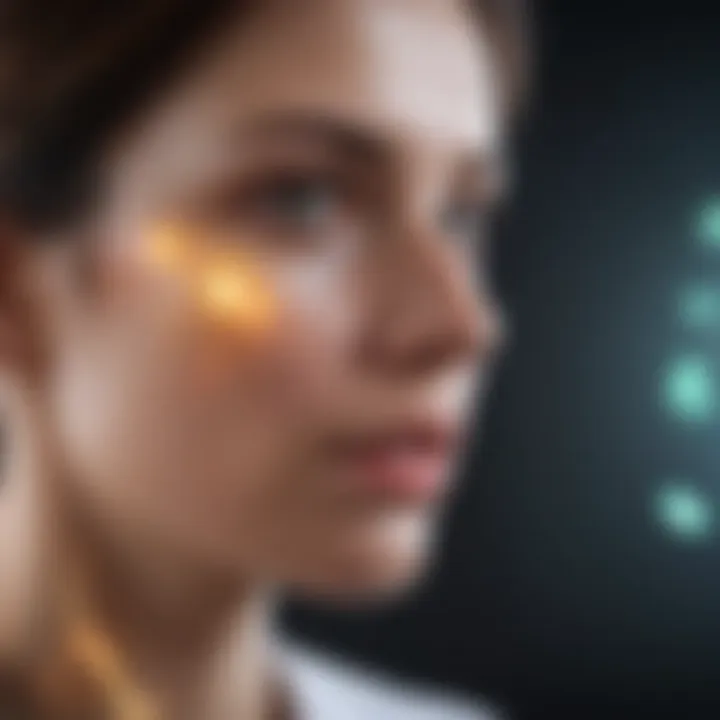
The intersection of photon technology and nanotechnology creates significant advancements in imaging and sensing. Nano-scaled photonic devices can enhance sensors' sensitivity to detect minuscule quantities of substances, which is invaluable in medical diagnostics and environmental monitoring. A unique aspect of these applications is their integration with biological systems, leading to potential breakthroughs in medical therapies. However, ethical implications surrounding the manipulation at the nanoscale, particularly human applications, necessitate stringent regulations and thoughtful advances.
Photonic technology underscores the importance of innovation in shaping a sustainable future, from energy solutions to the frontier of computing, and these advancements won't just change how we think—they will redefine what is possible.
Challenges and Considerations
As photon technology continues to evolve, it brings along its share of challenges and considerations which warrant careful attention. Understanding these issues is vital to harnessing the full potential of this technology while mitigating adverse impacts. The challenges range from ethical implications and technical limitations to future research directions, each presenting unique circumstances that can influence advancements in photon technology. Addressing these challenges effectively can lead to responsible innovations that benefit society without compromising ethics or feasibility.
Ethical Implications
One of the standout challenges surrounding photon technology is its ethical implications, particularly regarding its use in surveillance. The rise of optical surveillance systems has changed the landscape of security and privacy. While these systems can greatly enhance public safety, they also raise significant concerns about personal privacy and civil liberties. The key characteristic of this situation is the balance between security needs and individual rights, creating a unique tension. This duality makes it a compelling discussion point in understanding the broader impacts of photon technology.
Surveillance technologies often employ advanced photon detection methods, which can be incredibly efficient. However, the level of surveillance they enable could lead to potential overreach and misuse. For instance, the idea that almost every public space could be under constant monitoring may create a chilling effect on free expression. Thus, while it’s a beneficial advancement for safety, its widespread use can result in a lack of trust within communities.
Environmental Concerns
Environmental considerations are another critical challenge that needs addressing. Photon technology, particularly in energy generation through solar panels, comes with its own set of environmental impacts. The materials used in photovoltaic cells can raise concerns regarding their production and disposal. One of the key characteristics of this challenge is the sustainability factor associated with these technologies. They are, in theory, a great choice for reducing carbon footprints and harnessing renewable energy.
However, the production processes may not be as eco-friendly as one might assume. Unique features, such as the extraction of rare metals for these technologies, pose significant environmental risks including habitat destruction and pollution. Therefore, while solar energy is a step in the right direction, careful consideration is necessary to ensure that its benefits truly outweigh its drawbacks.
Societal Impact
The societal implications of photon technology's advancements also raise important questions. Innovations such as laser treatments in healthcare exemplify how beneficial photon technology can be. It can improve patient outcomes and offer unprecedented levels of precision. Yet, this leads to pressing concerns regarding access and equality. As healthcare continues to modernize with these sophisticated technologies, there exists a risk of widening the gap between those who can and cannot access such advancements.
In this context, the key characteristic here is equity in technological access. Photonic innovations could provide tremendous benefits to society but may simultaneously create divides based on socioeconomic status. Hence, while photon technology has the potential to elevate standards of living, its uneven distribution could exacerbate existing inequalities.
Technical Limitations
When discussing photon technology, technical limitations cannot be overlooked. Various enhancements in this field face notable barriers, chief among them being the cost of technology. While the advancements are groundbreaking, they often come with steep price tags, making access difficult for some communities and industries. The characteristics of high initial costs pose hurdles for widespread adoption. Technologies that require significant investment can deter smaller businesses from ever considering integration.
The unique feature of these costs often leads to prolonged development times, as innovators may struggle to secure funding or resources. As a result, while the benefits of photon technology are evident, the financial burden can hinder progress, keeping promising solutions just out of reach.
Scaling Production
Another critical aspect to consider is the scaling of production. As the demand for photonic applications rises, maintaining quality while increasing output is a pressing concern for manufacturers. The key characteristic of this issue is balancing efficiency with reliable production methods. It is crucial to develop scalable solutions that do not compromise the integrity of the technology itself. Unique challenges here include material shortages and infrastructure limitations, which can slow down the rollout of new technologies.
As companies strive to meet increasing demand, they may find themselves stretched thin, leading to potential inconsistencies in product quality. Therefore, while scaling production is necessary for meeting market needs, it can result in significant trade-offs.
Integration with Existing Systems
The last challenge to highlight involves the integration of new photon technologies with existing systems. Traditional systems often require adaptations that can sometimes be cumbersome. This situation poses the key characteristic of compatibility as a significant roadblock. The need for client adjustments can create frustrations for companies and consumers alike, as they may not be ready for the transition.
The unique feature of these integration challenges is that they highlight the importance of infrastructure readiness. Failure to successfully integrate new technologies can result in wasted resources and lost opportunities. Thus, while photon technologies promise advancements in various fields, the integration process must be smooth to harness their full potential.
Future Research Directions
Looking ahead, the future of photon technology hinges on various research avenues that can guide effective and responsible development. One promising path involves interdisciplinary approaches. Collaborating across disciplines can lead to innovative solutions that address multiple facets of photon technology's challenges. It opens doors to diverse perspectives, combining insights from physics, engineering, ethics, and more. The key characteristic here is the synergy that arises from sharing knowledge, which can foster more comprehensive solutions.
Interdisciplinary research has unique advantages. It can yield unforeseen innovations as researchers apply principles from one field to tackle problems in another. However, it also requires methodological flexibility, as it can sometimes challenge traditional academic boundaries.
Advancements in Theoretical Framework
Advancements in the theoretical framework surrounding photon technology is another essential area for future research. This aspect focuses on enhancing our understanding of photon behaviors and interactions, potentially leading to new applications in quantum computing and beyond. The key characteristic of advancing theories is that they can shape technological progress significantly. The unique benefit is that a more profound theoretical understanding can drive practical applications that are currently beyond our reach.
Collaborative Research Initiatives
Finally, fostering collaborative research initiatives stands out as a potent strategy for driving progress in photon technology. These initiatives can unite various stakeholders, including academic institutions, industry leaders, and governmental bodies. Such collaboration can be highly beneficial, presenting a framework for pooling resources toward common goals. The unique characteristic of these initiatives is that they encourage shared investment in research, which leads to increased innovation.
While collaborative efforts can amplify the productivity and impact of research, they may face challenges such as conflicting interests and resource allocation issues. Hence, overcoming these obstacles is imperative to fully capitalize on the potential of these partnerships.
By thoughtfully navigating through the challenges and considerations outlined, photon technology can continue on its path of evolution, ultimately enriching various sectors of society.
Finale
In wrapping up our exploration of photon technology, it’s crucial to recognize the profound implications this field holds for both scientific advancement and societal transformation. The multifaceted nature of photon technology not only reshapes industries but also opens avenues for innovation that were previously deemed unattainable. By harnessing the unique properties of photons, we've made strides in communication, energy generation, and medical imaging, among others.
Summing Up Innovations
Throughout the article, we’ve discussed noteworthy innovations stemming from advances in photon technology. These include:
- Laser Technology: Revolutionized medical procedures and manufacturing processes.
- Optical Fiber Communication: Enabled high-speed data transmission, essential for modern connectivity.
- Photonic Devices in Imaging: Advanced methods for diagnosing health issues and monitoring environmental changes.
These innovations exemplify how the quantum properties of light can lead to creations that serve essential roles in everyday life. They highlight how understanding photons can yield practical solutions to complex problems.
Vision for the Future of Photon Technology
Looking ahead, the potential for photon technology appears promising. Here are some anticipated developments:
- Integration with Quantum Computing: The merge of photon technology with quantum computing promises unprecedented processing capabilities.
- Sustainable Energy Solutions: Enhanced photovoltaic systems could lead to more effective solar energy capture and usage.
- Smart Materials and Metamaterials: These materials could greatly influence fields ranging from telecommunications to defense by manipulating photonic arrays in novel ways.