Photonic Integration: Transforming Optical Technologies
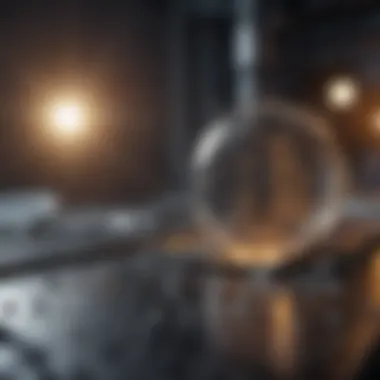
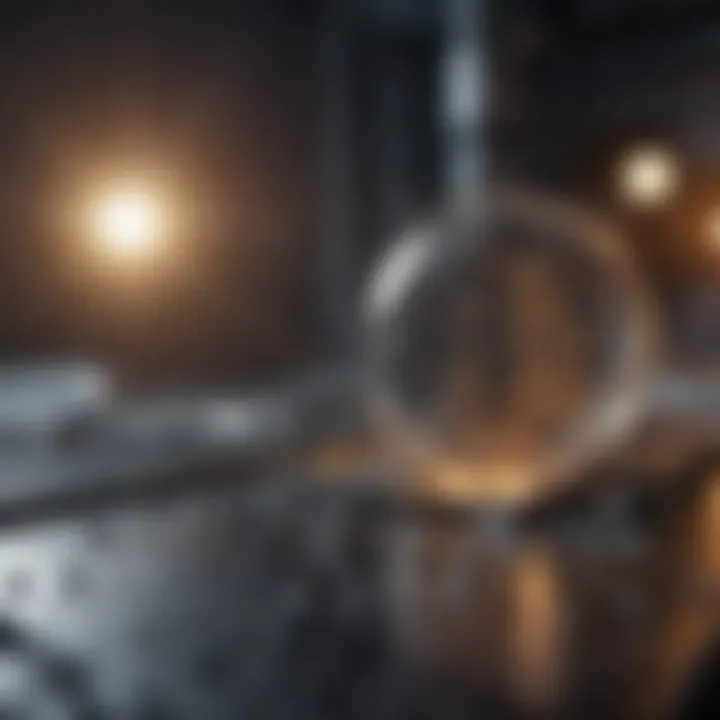
Intro
Photonic integration has emerged as a pivotal area in advancing optical technologies. This integration combines different photonic components onto a single chip, streamlining processes and enhancing the overall performance of optical systems. The importance of photonic integration is underlined by its diverse applications in telecommunications, sensing technologies, and computer systems. Furthermore, its potential to revolutionize industries cannot be overstated, as it enables faster data transmission rates and improved device efficiency.
The movement towards integrated photonics stems from the growing demand for faster, more reliable communication systems. Traditional methods often rely on bulky and complex setups that are challenging to scale. In contrast, integrating these components leads to reduced size and cost, making modern optical technologies more accessible.
This exploration will delve into the foundational principles of photonic integration, addressing its fabrication techniques, applications, and the challenges faced in this field. An understanding of these elements will provide insights into the transformative capabilities of integrating photonic components, paving the way for future innovations.
Research Highlights
Key Findings
In recent years, significant advances have been made in photonic integration.
- Efficiency Gains: The integration of waveguides, lasers, and detectors onto a single platform has resulted in improved energy efficiency.
- Scalability: Techniques such as photonic circuit design allow scaling to accommodate advances in technology, catering to increasing data needs.
- Cross-Disciplinary Synergies: Photonic integration benefits from advances in materials science and nanotechnology, enhancing fabrication techniques.
These findings underscore the importance of ongoing research and development in the field of integrated photonics.
Implications and Applications
Photonic integration's implications extend across various sectors. It is essential in:
- Telecommunications: Enhancements in data transmission rates.
- Sensing: Development of precise sensors for environmental monitoring and healthcare.
- Computing: Utilization in quantum computing for faster processing speeds.
These applications illustrate the versatility and significance of photonic integration in shaping the future of technology.
Methodology Overview
Research Design
The research on photonic integration primarily follows a multi-disciplinary approach. It combines theoretical analysis with practical fabrication techniques. Case studies of existing technologies provide valuable data to guide future innovations.
Experimental Procedures
Various fabrication methods are explored to achieve photonic integration. These include:
- Epitaxial Growth: A technique used to create semiconductor materials suitable for photonic devices.
- Lithography: Essential for creating precise waveguides and circuits.
- Etching Techniques: Important for defining the structures integrated into photonic chips.
Each method contributes uniquely to the overall efficacy of integrated photonic systems, revealing potential pathways for improvement and innovation.
"The integration of photonic components on a single platform is not just a technological advancement; it is a revolution in how optical systems are designed and utilized."
As the landscape of optical technologies continues to evolve, understanding the principles and practicalities of photonic integration is crucial for anyone engaged in the field.
Prolusion to Photonic Integration
The realm of photonic integration has become increasingly vital in modern technology. This area encompasses the contributions made by photonic components that manage light on a single platform. As optical technologies continue to mature, understanding the principles and methods of photonic integration is essential for leveraging its full potential. These technologies have transformed various sectors including telecommunications and sensing. Moreover, this integration promises compact systems and improved performance, providing significant benefits over traditional technologies.
Definition and Significance
Photonic integration refers to the combination of multiple photonic devices on a single substrate. This process allows for enhanced functionality, miniaturization, and cost-effectiveness. By integrating lasers, modulators, and detectors into one chip, we can achieve functionality previously only possible through discrete components. This evolution not only enables faster signal processing but also reduces power consumption.
The significance of photonic integration lies in its potential to marry the benefits of optics with the computational advantages of silicon-based technologies. This integration supports the development of more efficient communication networks, faster data processing speeds, and superior sensing capabilities. As industries move towards digitization and the internet of things (IoT), the relevance of photonic integration grows stronger.
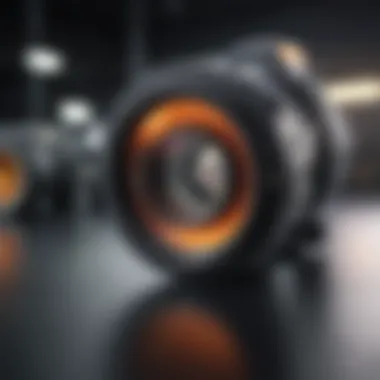
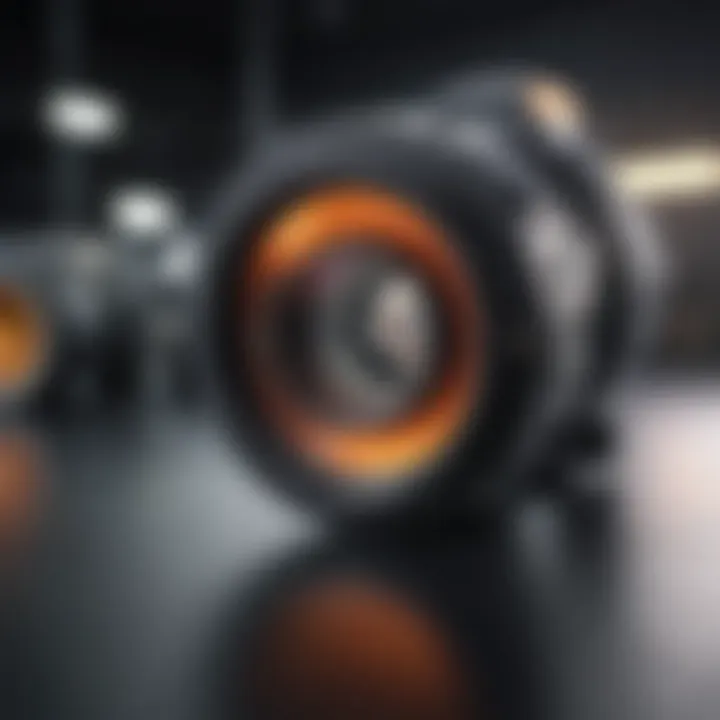
Historical Context
The concept of integrating photonic devices can be traced back to the early explorations of optics. Initially, devices were large and cumbersome, limiting their use in practical applications. The advent of waveguide technology in the 1980s marked a turning point. Scientists realized the potential for creating compact, efficient systems through integration. This included the development of silicon photonics as a promising platform due to its established manufacturing processes.
As the demand for high-speed data transfer escalated, research into photonic integration saw significant growth in the late 1990s and early 2000s. Today, advancements in microfabrication techniques and material sciences enable researchers to develop more sophisticated photonic circuits. This progression highlights a historic shift towards optical solutions that can meet modern technological needs.
"The integration of photonic devices is not merely a trend; it is a necessity for future technological advancements in communications and computing."
As we explore the fundamental principles and applications in subsequent sections, we will uncover how photonic integration continues to reshape the landscape of optical technologies.
Fundamental Principles of Photonic Integration
The fundamental principles of photonic integration serve as the backbone for understanding how light-based technologies evolve and advance. They underpin the interactions and behaviors of light within various materials and structures. This section highlights the crucial elements of these principles and their implications for the field. Integrating light components on platforms enhances capabilities such as data transmission, sensing accuracy, and computational efficiency. By grasping these principles, one appreciates the nuances of designing and optimizing integrated photonic systems, which leads to innovative applications.
Light-Matter Interaction
Light-matter interaction is a core principle in photonic integration. This phenomenon informs how photons interact with atoms and molecules, a process essential for the development of technologies such as lasers and detectors. When light passes through materials, it may be absorbed, emitted, or transmitted depending on the material's properties.
The key factors affecting light-matter interaction include:
- Absorption: Light energy is absorbed, causing excitation of electrons within the material, which can lead to phenomenon such as fluorescence.
- Emission: After absorption, energy is re-emitted, contributing to the efficiency of lasers.
- Scattering: This affects how light propagates within a medium, impacting signal integrity in communication systems.
By optimizing these interactions, engineers can design photonic devices that operate with higher efficiency. For example, certain materials can be chosen to enhance absorption or minimize loss, depending on the application's needs. Understanding light-matter interactions is vital for advancing integrated photonics, especially in sectors like telecommunications and biosensing.
Waveguiding Principles
Waveguiding principles govern how light is directed and manipulated within integrated photonic systems. A waveguide confines light to specific paths, preventing scattering away from the intended route. This confinement is critical for maintaining signal integrity, minimizing loss, and enabling dense integration of optical components on small chips.
Key aspects of waveguiding include:
- Total Internal Reflection: This is the primary mechanism that keeps light confined within a waveguide. When light enters at an angle exceeding a critical angle relative to the boundary between two materials, it reflects entirely back into the denser medium.
- Mode Propagation: Each waveguide supports a range of modes, which are specific field distributions of the light. Higher-order modes may lead to increased complexity in waveguide design.
- Material Selection: Different materials impact the refractive index and, consequently, the performance of waveguides. Silicon, for example, has gained attention for its compatibility with existing semiconductor technology.
Designing effective waveguides requires a careful balance of these principles, aimed at achieving low-loss transmission, high-speed data transfer, and broad operational bandwidth. Their importance cannot be overstated in fields ranging from optical communication to sensor applications.
"The principles of light-matter interaction and waveguiding define the efficiency and capability of photonic systems, finding applications across multiple disciplines."
By integrating these fundamental principles into the framework of photonic integration, we are paving the way for future advancements. This understanding is instrumental in driving innovations and addressing challenges in evolving optical technologies.
Fabrication Techniques
In the rapidly evolving field of photonic integration, the methods of fabrication are crucial for achieving the desired performance and reliability of photonic devices. The techniques discussed in this section emphasize their role in optimizing the integration process and ensuring the efficiency of optical systems. With advancements in materials and approaches, fabrication techniques are pivotal in bridging the gap between laboratory-scale prototypes and commercial products.
Microfabrication Methods
Microfabrication is the cornerstone of photonic integration, facilitating the production of microstructures that are integral to photonic circuits. Techniques such as lithography, etching, and deposition enable the creation of intricate patterns on substrates, allowing for precise control over optical properties.
- Lithography: This process uses light to transfer a pattern onto a photoresist layer on a substrate. Through various techniques such as photolithography or electron-beam lithography, circuits with spatial precision on the microscale can be achieved.
- Etching: After lithography, etching removes material to form the desired components. Both wet and dry etching methods play essential roles, enabling the creation of waveguides and resonators. These methods allow for selective material removal, essential for functional device characteristics.
- Deposition: This includes techniques like chemical vapor deposition (CVD) and physical vapor deposition (PVD). These methods layer materials atom by atom or molecule by molecule, creating the thin films necessary for effective optical functionality.
Microfabrication methods are important because they enable the scalability of photonic devices, making them suitable for mass production while maintaining high precision.
Integration on Silicon Platforms
Silicon-based platforms are increasingly utilized for photonic integration due to their compatibility with existing microelectronics. The integration of photonic components on silicon enhances the potential for compact, high-performance devices.
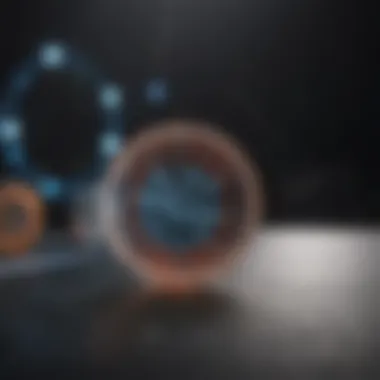
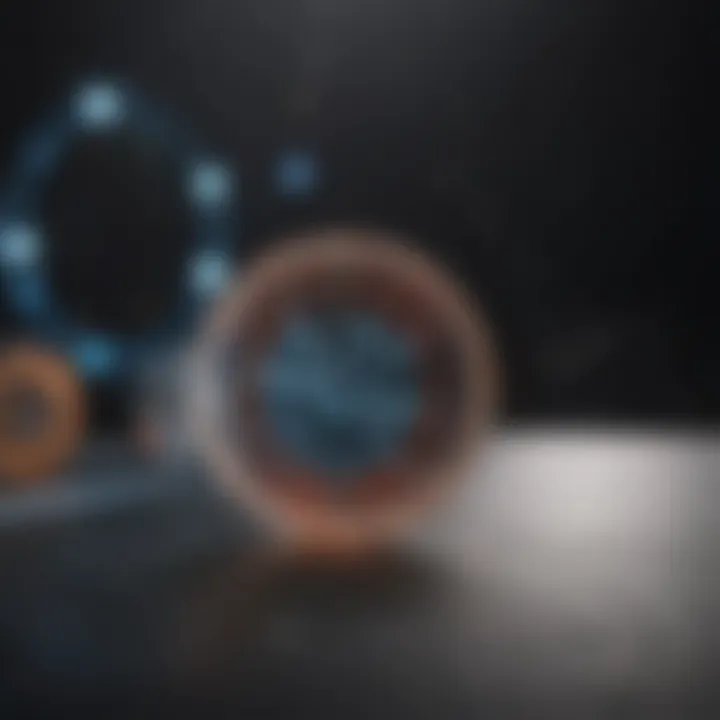
Using silicon provides several benefits:
- Cost-effectiveness: Utilizing mature silicon fabrication techniques developed for microelectronics leads to lower production costs.
- High integration density: Numerous components can be integrated on a single chip, reducing size and improving performance.
- Compatibility with electronics: Combining photonics with electronics allows for seamless integration in applications like data centers and telecommunications.
Materials Used in Photonic Integration
The choice of materials plays a fundamental role in photonic integration. Various materials provide different optical properties, impacting device performance. Some of the key materials include:
- Silicon: Dominant in photonic offerings due to its favorable optical properties and ease of integration with electronics.
- Silicon Nitride: It offers lower optical losses, making it useful for specific applications, including biosensing and telecommunications.
- III-V Semiconductors: Materials like indium phosphide (InP) and gallium arsenide (GaAs) allow for efficient light emission and are often used in laser integration.
The selected materials must align with the desired optical properties and operational bandwidth, making the understanding of material science a critical aspect of photonic integration.
"Effective fabrication techniques provide the backbone for the successful implementation of photonic systems, establishing a road toward innovations in communication, sensing, and computing environments."
In summary, mastering fabrication techniques is essential for the development of advanced photonic technologies. These techniques bridge the theoretical underpinnings of photonic integration and their real-world applications, addressing challenges of cost, performance, and integration density.
Applications of Photonic Integration
The applications of photonic integration span a wide range of industries and fields. These applications signify the importance of integrating optical components on a single platform, affecting both performance and versatility. By exploring the various applications, we can understand not only their immediate benefits but also the broader impacts on technology and society.
Telecommunications
Telecommunications is one of the most prominent fields benefiting from photonic integration. The integration of photonic devices enables faster data transmission, vital for meeting the ever-increasing demand for bandwidth. For instance, integrated photonic circuits, often based on silicon, facilitate the development of compact transceivers that can connect high-speed optical fibers.
Key advantages include:
- Higher data rates without needing extensive physical infrastructure.
- Reduced power consumption, which is essential in sustainable technologies.
- Greater device miniaturization allows for more efficient designs in consumer electronics.
With the consistent growth of internet traffic and the introduction of 5G technology, photonic integration offers a future-proof solution for telecommunications.
Sensing Technologies
Sensing technologies are another significant domain where photonic integration plays a crucial role. The ability to deploy numerous sensors in a compact system allows for real-time monitoring in various environments. Integrated photonics can improve sensitivity and resolution in sensors used for environmental monitoring, biomedical diagnostics, and industrial applications.
Consider the following benefic marks:
- Enhanced rapidity in sensing responses can lead to timely decision-making.
- Integration enables multiparametric sensing, where multiple types of measurements can be conducted simultaneously.
- Photonic sensors often provide superior performance in challenging conditions, including high temperatures or corrosive environments.
This integration proves particularly useful in developing smart cities, where comprehensive real-time monitoring is essential for infrastructure management.
Quantum Computing and Photonics
Quantum computing represents a frontier in technology potentially revolutionizing information processing. Photonic integration opens pathways to creating scalable quantum systems using optical components. The ability to manage quantum bits or qubits efficiently can provide substantial advantages in computational speed and power.
Important elements include:
- The inherent speed of light in photonic systems can significantly enhance processing times.
- Integration allows for the implementation of sophisticated quantum algorithms in a more compact and reliable manner.
- Photons are less susceptible to environmental disturbances compared to other types of qubits, improving operational stability.
The combination of these factors paves the way for significant advancements in quantum technologies and strengthens their practical applications.
"The impact of photonic integration in applications is transformative, leading to new capabilities across industries."
Comparative Analysis
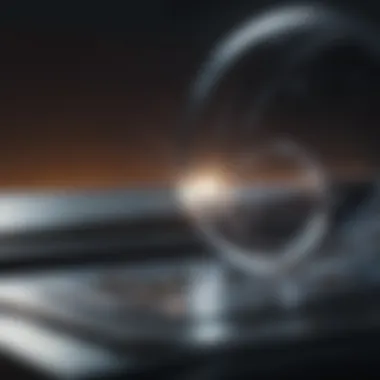
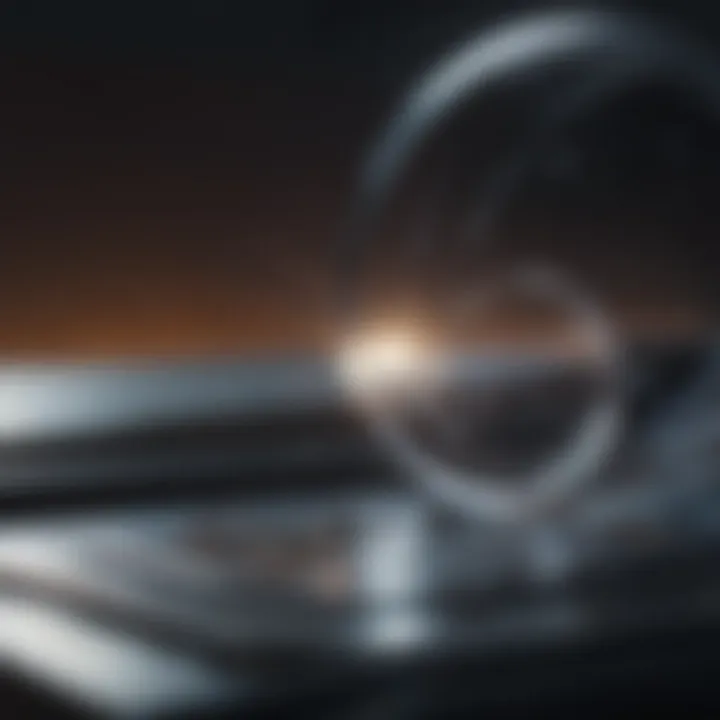
The comparative analysis of photonic integration is crucial in highlighting its advantages and limitations as compared to traditional optical technologies. Understanding this comparison provides insights into the evolution of photonic systems. In this section, we will explore two key areas: the benefits that photonic integration offers over conventional methods and the challenges that accompany such integration efforts.
Benefits over Traditional Methods
Photonic integration offers several advantages that position it above traditional optical technologies. These benefits include:
- Miniaturization: Photonic integrated circuits (PICs) can pack more functionality into a smaller space. This is important for devices used in telecommunications and sensing, where size and footprint play critical roles.
- Energy Efficiency: Integrated photonic devices generally consume less power than their discrete counterparts, leading to lower operational costs and enhanced sustainability. The efficient use of light minimizes energy loss, which is fundamental in applications like data centers.
- Enhanced Performance: Integrated systems can achieve higher performance levels. Reduced interconnect losses and faster signal processing are significant benefits, making photonic integration suitable for high-speed data transmissions.
- Cost-Effectiveness: While initial setup costs can be high, the long-term savings due to reduced manufacturing and operational expenses show a clear financial advantage. Mass production capabilities for integrated devices also drive down costs.
- Scalability: Photonic integration enables the efficient scaling of systems, allowing for easier adaptations and upgrades as technology evolves. This adaptability is essential in fast-paced technology sectors like telecommunications.
Challenges Faced in Integration
Despite the numerous advantages, there are also challenges that hinder the widespread adoption of photonic integration. Understanding these challenges is vital for addressing them effectively:
- Technical Complexity: The design and fabrication of integrated photonic devices are technically complex. Creating reliable and efficient interfaces among different materials and components is a challenging process that often requires advanced skills and tools.
- Material Limitations: Current materials used in photonic integration, like silicon, have inherent limitations in their optical properties. This impacts the performance of devices, especially in specific wavelength ranges.
- Integration with Existing Systems: Combining photonic systems with established electronic technologies presents integration issues. Achieving compatibility between different systems often requires additional research and development.
- Manufacturing Standards: The absence of standardized manufacturing processes for photonic devices leads to variability in quality and performance. Establishing industry standards will be necessary to gain wider acceptance.
- Market Readiness: The market for integrated photonic technologies is still developing. Businesses may be hesitant to transition away from established technologies without a clear market-driven incentive.
Integrating photonic technologies holds transformative potential but requires overcoming significant hurdles.
Future Trends in Photonic Integration
As photonic integration continues to evolve, understanding its future trends becomes imperative. The ongoing development in this field indicates a shift towards more sophisticated and efficient optical technologies. These trends are not merely incremental; they hold significant implications for industries and applications where speed, accuracy, and miniaturization are paramount. By focusing on emerging technologies and their potential market impacts, we can better grasp the transformative nature of this discipline.
Emerging Technologies
In the realm of photonic integration, several emerging technologies are starting to capture the attention of researchers and industry leaders alike. Some key areas include:
- Integrated photonics: This involves the integration of passive and active optical components onto a single chip. Such platforms can drastically reduce size and costs, making them ideal for telecommunications and other applications.
- Silicon nanophotonics: This technology leverages silicon for manipulating light at the nanoscale. The advantages include high compatibility with existing semiconductor processes and potential for high-volume manufacturing, fundamentally changing the landscape of photonic devices.
- Quantum photonics: This area focuses on the integration of quantum bits into photonic systems. It has significant implications for secure communication and advanced computing capabilities, paving the way for the next generation of quantum technologies.
"Emerging photonic technologies are set to redefine the boundaries of computation, communication, and sensing capabilities."
Each of these technologies presents unique challenges while offering promising solutions that will define future innovation in the industry. Addressing the engineering hurdles, while fostering interoperability among diverse components, will be essential in advancing these technologies further.
Potential Market Impacts
The implications of these emerging technologies extend beyond mere technical advancements. The potential market impacts are profound:
- Cost Reduction: By integrating components and reducing reliance on multiple individual parts, the overall costs associated with manufacturing and deployment will decrease.
- Increased Performance: With technological advancements, the efficiency of optical devices will improve. This will have positive repercussions in various fields, particularly in data centers and telecommunications where speed is critical.
- Market Expansion: As photonic integration becomes more accessible, new markets will likely develop, enhancing possibilities for small and emerging companies while revitalizing large corporations.
- Sustainability: Emerging technologies often focus on energy efficiency. Photonic integration can lead to designs that consume less power compared to traditional electronic counterparts, aligning with global sustainability goals.
In summary, the future of photonic integration is marked by significant technological innovations and market transformations. By recognizing these trends and their implications, stakeholders can better position themselves to leverage the advancements in optical technologies.
Epilogue
The conclusion of this article serves as a pivotal wrap-up to the extensive discussion on photonic integration. This topic is paramount in understanding how optical technologies are advancing through innovative integration strategies. The key elements found in this exploration include the synthesis of foundational principles with cutting-edge applications across various fields.
By integrating distinct photonic components into a singular platform, there is a significant enhancement in performance and efficiency. Such advancement not only boosts the capabilities of telecommunications but also fosters innovations in sensing technologies and quantum computing.
Summary of Findings
In summary, photonic integration has emerged as a linchpin for the future of optical technologies. It harnesses the interplay between light and matter to create faster, more reliable systems. Key findings include:
- Efficiency Gains: Multi-functional devices can handle multiple tasks simultaneously, reducing bulk and improving performance.
- Cost Reduction: By utilizing silicon platforms, fabrication costs decrease while maintaining high standards of quality.
- Versatility Across Industries: The applications of photonic integration cut across multiple sectors, enhancing capabilities in communication, sensing, and computing before unseen.
Overall, the synthesis of these findings illustrates the transformative potential of photonic integration and its role in driving the next phase of technology.
Call for Future Research
Looking ahead, the call for future research in photonic integration is crucial. As technologies evolve, there are several areas ripe for exploration:
- Enhancement of Fabrication Techniques: New methods can lead to improved precision and scalability, effecting a further drop in costs.
- Cross-Disciplinary Applications: Investigating how photonic integration can be combined with other technologies like AI may yield innovative solutions and applications.
- Sustainability Concerns: Research addressing the environmental impact of materials and processes in photonic integration needs to be heightened.
Addressing these areas will not only promote scientific progress but also ensure that the integration of photonics remains relevant and impactful in an ever-evolving technological landscape.
As photonic technologies continue to develop, they promise to redefine applications from telecommunications to advanced computing, making ongoing research an endeavor of great significance.