Protein A Affinity Column: Mechanisms and Applications
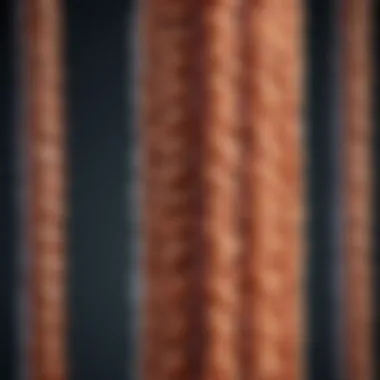
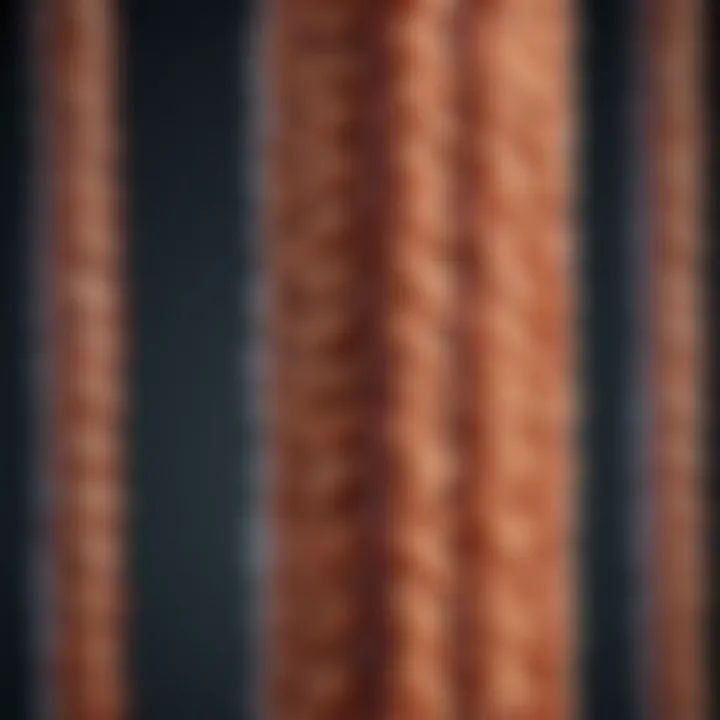
Intro
Protein purification is a fundamental process in biochemistry and molecular biology, pivotal for the study of proteins' functions and structures. Among various techniques, Protein A affinity chromatography stands out due to its specific interactions with immunoglobulins, enabling the isolation of antibodies and other proteins with high fidelity and yield. This succinct method not only streamlines the purification process but also presents a wealth of possibilities for expanding our understanding of complex protein dynamics.
Exploring the mechanisms underlying Protein A affinity columns reveals a fascinating interplay of biochemistry and practical application. The focus is on how proteins interact at a molecular level and how this knowledge translates into impactful laboratory practices. In this article, we aim to dissect the nuances of these affinity columns—from their design and operational protocols to the advanced challenges faced in real-world applications.
Our journey into the mechanisms and applications of Protein A affinity columns promises to unveil insights that stretch beyond traditional approaches. Whether you are a seasoned researcher or a budding student, the following sections are tailored to enhance your comprehension and spark deeper inquiry into the realm of protein purification technologies.
Preamble to Protein A Affinity Columns
In the realm of biochemistry and molecular biology, the significance of Protein A affinity columns cannot be overstated. They are a cornerstone technique for the purification of proteins, specifically monoclonal antibodies, which are pivotal in both research and therapeutic contexts. As the modern landscape of biotechnology continues to evolve, understanding the mechanisms and applications of these columns is essential for advancing both academic and commercial endeavors.
Definition and Overview
Protein A affinity columns utilize Protein A, a domain of the Staphylococcus aureus protein, which has a high affinity for the Fc region of immunoglobulins. This property makes these columns incredibly effective for isolating antibodies from complex mixtures. The basic principle is that sample proteins, primarily antibodies, are passed through a column filled with a matrix that is covalently linked to Protein A.
When the sample is introduced, the antibodies bind to the column while other proteins and contaminants are washed away. Subsequently, the bound antibodies can be eluted using specific buffers, typically involving changes in pH or salt concentration. This method allows for a relatively pure form of the desired antibody to be collected, which is crucial for further applications, including diagnostic tools, therapeutic agents, and research purposes.
Historical Development of Affinity Chromatography
The journey of affinity chromatography has been quite remarkable. Initially, in the mid-20th century, researchers were exploring ways to efficiently separate biomolecules from complex mixtures. The first mention of affinity chromatography dates back to the 1960s, achieving prominence in the 1970s as the technique was refined.
One of the early developments was the coupling of enzymes or ligands to adsorbents, but it was not until the pioneering efforts of Paul Berg and others in the early 1980s that the principles of Protein A affinity were effectively applied to immunoglobulin purification. As the field advanced, the technology behind Protein A became more sophisticated, leading to the development of new resin materials and improved methodologies that enhance binding efficiency and reduce processing times.
"The evolution of affinity chromatography reflects the dynamic nature of scientific inquiry and technological advancement, enabling researchers to harness the power of antibodies for varied applications."
Today, Protein A affinity columns are widely utilized in both research laboratories and large-scale production environments. Their use in biopharmaceutical manufacturing, especially for the production of monoclonal antibodies, is standard practice, showcasing the essential nature of this technique in the ongoing development of biologics.
As we continue through this article, expect to delve deeper into the mechanics behind Protein A interactions, the specific design of these affinity columns, and how they can be optimized for diverse biochemical applications.
Theoretical Foundation of Protein A Interaction
The theoretical foundation of Protein A interactions is crucial to understanding how this affinity chromatography technique works. It provides insights into the biology of proteins and their circulations, establishing the basis for effective purification strategies. Comprehending this background helps researchers and practitioners alike to exploit the full potential of Protein A affinity columns, ensuring more efficient protocols while minimizing potential pitfalls during operation.
Protein Structure and Function
Proteins are dynamic molecules, heavily dependent on their structure for functionality. The tertiary structure of a protein, often referred to as its conformation, dictates how it interacts with other molecules. This notion is not mere scientific jargon; it’s the very essence that underpins the mechanisms of Protein A affinity columns. Protein A specifically binds to the Fc region of antibodies. This unique interaction arises due to the presence of constant domains in the IgG (immunoglobulin G) class of antibodies.
Not all proteins follow the same structural blueprint, nor do they yield the same results in purification processes. Variations in amino acid composition and modifications, such as glycosylation, can influence how well proteins bind to the resin. This complexity demands a well-rounded understanding of both protein chemistry and affinity interactions. If one is not attuned to these subtleties, it’s easy to shoot oneself in the foot when attempting purification.
Mechanisms of Binding
The effectiveness of Protein A affinity chromatography hinges significantly on precise mechanisms that govern binding. These mechanisms encompass binding strength, specificity, and the influence of external factors.
Affinity and Specificity of Protein A
Affinity and specificity are hallmarks of Protein A’s binding characteristics. Affinity refers to the strength of the interaction between the Protein A resin and the antibody, whereas specificity concerns how selectively Protein A binds to the antibody without engaging other proteins. This specificity reduces the chances of non-specific interactions that might muddy the results during purification.
The high affinity of Protein A for antibodies is primarily due to the unique structure of its binding sites, which interact favorably with certain regions of the antibody's Fc domain. This contribution to the purification process cannot be understated, as it ensures that most of the desired proteins are effectively isolated from the cellular milieu. The decision to use Protein A in purification protocols often arises not just from a technical necessity, but from a strategic choice based on how reliably it can deliver compelling results.
Despite its advantages, reliance on affinity can also lead to complications. If the conditions of purification are not meticulously controlled, issues, such as elution of contaminants alongside antibodies or incomplete washing, can arise. Therefore, while the attractiveness of high specificity is undeniable, so is the need for rigor in its application.
Impact of Environmental Factors
Environmental factors—things like pH, temperature, and ionic strength—play a pivotal role in the Protein A binding process. The interplay of these factors can make or break the efficiency of the purification. For example, varying the pH can affect the charge distribution on the protein surface, potentially enhancing or diminishing the binding capabilities of Protein A.
Temperature can also influence binding kinetics. Typically, higher temperatures can accelerate reactions; however, for proteins, higher temperatures might lead to denaturation, losing their functional shape. Thus, balancing these environmental conditions is key to optimizing binding protocols.
Notably, Ionic strength is another factor that can drastically change how Protein A binds. Adjustments to salt concentrations, for example, influence electrostatic interactions, which can either strengthen or weaken binding.
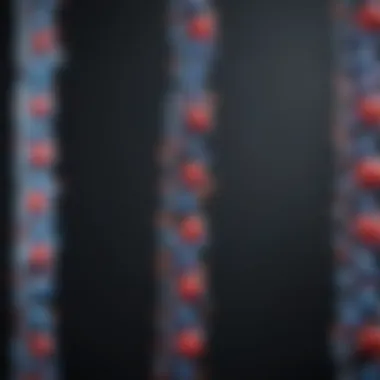
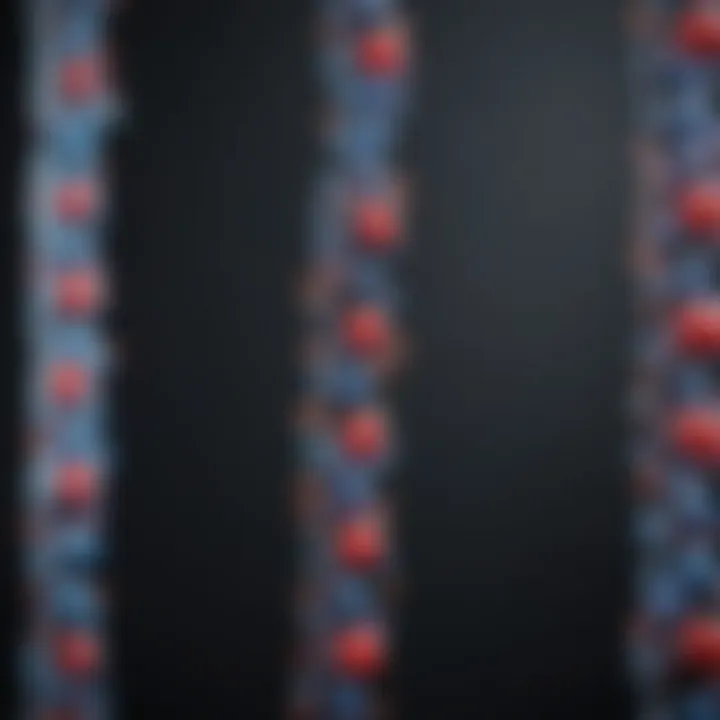
It's essential to approach these factors as facilitatory tools rather than constraints, adapting protocols accordingly for optimal outcomes.
"Understanding the theoretical foundation of interactions provides a roadmap for navigating complex purification challenges."
Design and Composition of Protein A Affinity Columns
The design and composition of Protein A affinity columns is a cornerstone of effective protein purification. It’s not just about slapping together some materials and calling it a day; the specificity and efficiency of the purification process hinges on meticulously selecting components that work in concert. The interplay of materials used and the construction techniques followed can mean the difference between a productive run and hours wasted, chasing elusive proteins.
Materials Used
Resin Types
When we think about resin types, what comes to mind immediately is their pivotal role in capturing the target proteins. These resins are often made from cross-linked agarose or polymer systems. The affinity interaction between the resin and the protein of interest is fundamental for a successful separation process. One key characteristic that sets resins apart is their binding capacity, which directly impacts the efficiency of the purification strategy. For example, immobilized protein A on agarose resins allows high capacity due to the large surface area available for binding.
However, it’s not all cut and dry. The composition of the resin can influence not only binding capacity but also the overall stabilization of proteins during purification. Some resins can bind more non-specifically to contaminants, which complicates the purification workflow. This brings us to the heart of the matter: carefully choosing the right resin based on the protein’s properties can significantly enhance purity and yield, making it a beneficial choice in biochemistry labs across the board.
Linker Materials
Equally as crucial in the assembly of Protein A affinity columns are the linker materials. These are the connectors that bind the resin and protein A, and they are often overlooked but hold immense importance in the binding process. A standout characteristic of linker materials is their flexibility; this impacts how well the protein can approach the resin, influencing its ability to bind effectively. Popular choices often include hydrophilic and hydrophobic linkers that can either encourage or discourage interactions depending on the context of the purification.
The unique feature of linker materials is their varied lengths and chemistries, which adapt the interaction surface area for different proteins. This adaptability not only increases efficiency but also decreases the chances of non-specific binding. However, one downside is that some linker arrangements might eventually lead to steric hindrance, where a bulky design may inhibit proper enzyme accessibility. Thus, careful attention to choosing linker materials will pay dividends, making it an integral aspect of column design in this article's context.
Column Construction Techniques
Constructing a Protein A affinity column isn’t merely a mechanical task; it requires an understanding of how each component interacts at a molecular level. There are several techniques available, including packing resins into a column format using gravity or pressure methods. One must consider not only the alignment of conditions conducive for optimal binding but also how to achieve a uniform packing density to prevent undesirable channeling effects during flow.
From the outset, ensuring that the resin bed is not overly compact is critical. An overpacked column might restrict flow rates and lead to poor recovery of proteins, creating a frustrating bottleneck in the process. All in all, the art of constructing a Protein A affinity column combines science and skill, a dance of precise techniques tailored towards successful protein isolation.
Protocols for Protein Purification
Protocols for protein purification are crucial in leveraging the benefits of Protein A affinity columns. These strategies define the steps taken to isolate, purify, and analyze proteins effectively. Not only do they underscore the importance of systematic approaches in biochemical research, they also facilitate reproducibility and yield consistent results. Failing to follow established protocols can lead to suboptimal recovery of the desired proteins, which is a concern that researchers strive to avoid at all costs.
General Workflow
The general workflow in protein purification through Protein A affinity columns begins with sample preparation, where biological samples such as serum or cell lysates are obtained. The aim is to harvest the proteins of interest while minimizing contaminants. In the next step, the sample is loaded onto the affinity column containing Protein A-ligated resin. The affinity interaction ensures that target proteins, typically antibodies, bind to the column while others do not. This step is akin to fishing with a net that captures only the larger fish while letting the smaller ones swim away.
To enhance efficiency, washing steps are included. These washes help eliminate non-specifically bound proteins, refining the purity of the captured analytes. After this, the elution step is essential, which frees the target proteins from their solid-phase capture. The protocols should detail the nature of the elution buffers used, as inappropriate elution conditions can damage the protein of interest or reduce yield significantly.
Optimization of Binding Conditions
Optimizing binding conditions is key for enhancing the performance of Protein A columns. This involves adjusting parameters such as pH, ionic strength, and temperature. For instance, ensuring that the pH is at optimal levels can vastly improve the binding efficiency of Protein A to IgG. A common practice is to start establishing these conditions during exploratory runs and iteratively refine them based on results.
Moreover, employing different salt-concentration gradients in the binding buffer can help create a more conducive environment for protein attachment to the column. An imprecise setup can lead to issues such as poor binding, exemplifying the need for comprehensive consideration during this phase.
Elution Strategies
Elution strategies are pivotal in determining how proteins are recovered from the affinity column. There are various methods to elute the bound proteins, with Low pH Elution and High Salt Elution being two prominent strategies.
Low pH Elution
Low pH elution is a widely recognized method where an acidic buffer, often below pH 3, is used to release the proteins from the column. This strategy leverages the weak interactions formed between the proteins and Protein A, effectively breaking the bond without causing significant structural damage to the target protein. This is especially popular because many antibodies remain stable under such low pH conditions. What makes this approach stand out is its simplicity and efficiency, making it easy to integrate into established protocols. However, care must be taken, as prolonged exposure to low pH can lead to denaturation of some proteins. Thus, the timing of exposure is vital.
High Salt Elution
On the other hand, High Salt Elution employs a buffer containing high concentrations of salt to disrupt the ionic interactions. This method is often classified as a gentle way to elute proteins while avoiding extreme changes in pH, making it suitable for sensitive proteins. The most striking feature of this technique is its versatility; it allows researchers to tailor the elution conditions to the specific needs of their protein. However, one must be cautious about the potential for salt precipitation in subsequent processing steps, which can complicate further purification processes.
In summary, the protocols for protein purification through Protein A affinity columns are multifaceted and demand careful attention to detail. Each step, from preparing the sample to optimizing binding conditions and executing elution strategies, plays a crucial role in the successful isolation of proteins.
Applications in Biochemistry and Biotechnology
The role of Protein A affinity columns in biochemistry and biotechnology cannot be overstated. These columns have found their niche in various applications, driving innovation in both therapeutic and research settings. Understanding their benefits and considerations not only enhances their use but also paves the way for improved techniques in protein purification.
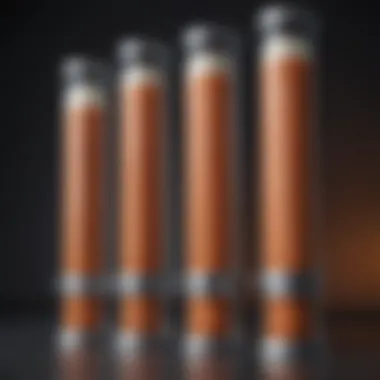
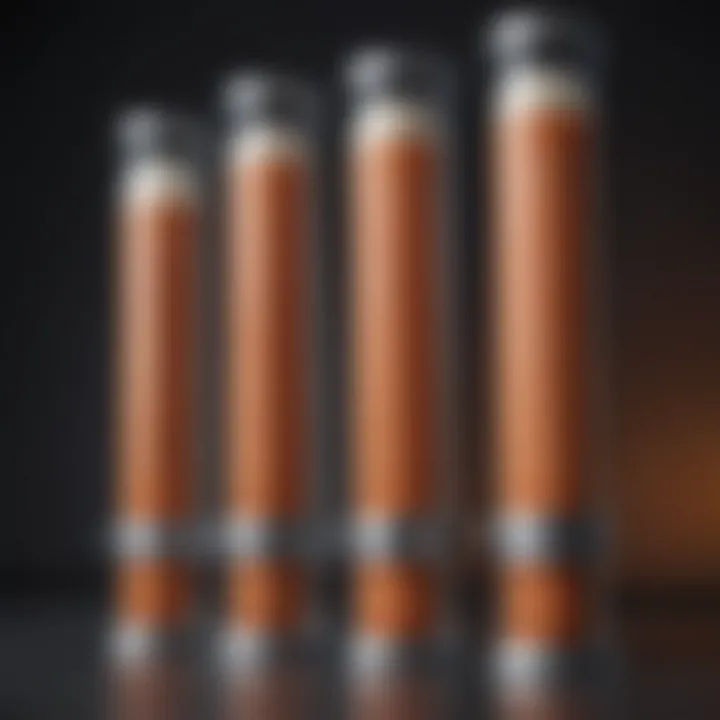
Monoclonal Antibody Purification
Purifying monoclonal antibodies is one of the flagship applications of Protein A affinity columns. Monoclonal antibodies are pivotal in disease treatment, particularly in cancers and autoimmune disorders. They have typically been used in therapeutic protocols, and their purification is crucial to ensure efficacy and safety for clinical application.
- Specificity: Protein A specifically binds to the Fc region of antibodies, allowing for a high yield of purified product.
- Efficiency: The process can be relatively quick compared to other methods. By running the sample through a Protein A column, researchers can achieve significant purification in a single step.
- Scalability: These columns can be scaled up for large batch operations, making them suitable for industry needs as well as laboratory settings.
While the advantages are clear, nuances, such as the need for appropriate elution conditions, cannot be neglected. If the elution is not performed under optimal conditions, there might be losses in antibody function or yield. The fine balance between yield and purity must be handled meticulously.
Recombinant Protein Recovery
In the world of recombinant proteins, where the aim is to express and purify proteins artificially, Protein A affinity columns again come into play. Here, the implications stretch beyond just simple purification; they involve enhancements in research and development.
- Yield Maximization: Incorporating these affinity columns into protocols facilitates the recovery of large amounts of recombinant proteins. Proteins that are often difficult to isolate with standard techniques can be efficiently captured.
- Complex Solutions: In many cases, recombinant proteins are expressed in mixed solutions. Protein A can effectively distinguish target proteins from contaminants, streamlining the purification process.
- Detection and Analysis: The application of Protein A columns also includes the direct analysis of the recovered proteins, contributing to advancements in proteomics.
However, it’s important to note that the success of this application largely hinges on the expression system used and the protein's characteristics, which may affect its behavior in the column.
Characterization of Protein Interactions
Protein interactions define molecular biology's core, and understanding them is vital. Protein A affinity columns aid in characterizing these interactions by offering ways to isolate and analyze proteins of interest. This not only serves the research community but also has implications for therapeutic developments.
- Binding Kinetics: Researchers can study binding kinetics through elution profiles obtained from the columns. By running experiments under varied conditions, insights into the dynamic processes can be gathered.
- Co-immunoprecipitation: The columns can assist in co-immunoprecipitation experiments, helping researchers observe interactions in protein complexes, which is essential in signal transduction studies.
- Specific Assays: Protein A columns can be integrated into specific assays, allowing for the quantitative analysis of protein interactions in cellular contexts.
Understanding these interactions can unlock pathways for drug development, improving therapeutic strategies and leading to more targeted interventions.
"The impotence of knowledge becomes apparent when we see the potential for Protein A affinity columns to push boundaries in both clinical and research contexts."
In summary, the applications of Protein A affinity columns in biochemistry and biotechnology are multifaceted, with each application offering unique benefits and challenges. The continued exploration of these columns is vital for refining techniques and advancing the field.
Challenges in Protein A Affinity Chromatography
In the realm of protein purification, while Protein A affinity chromatography stands as a cornerstone technology, it doesn’t come without its hurdles. Understanding the challenges faced during its application is crucial for researchers and practitioners alike. These difficulties not only affect the efficacy of the purification process but can also impact the quality of biomolecules that researchers aim to isolate. An awareness of these challenges can lead to better experimental design and optimization strategies, ultimately contributing to more efficient purification protocols.
Non-Specific Bindings
One prominent challenge in Protein A affinity chromatography is the issue of non-specific bindings. This phenomenon occurs when proteins or other biomolecules adhere to the resin that are not intended targets. Such undesired interactions can skew the results and lower the purity of the isolated proteins.
To elaborate, proteins often display surface properties that can engage in non-specific interactions. These interactions primarily arise due to:
- Hydrophobic interactions: Many proteins have hydrophobic patches which can lead them to stick to resin surfaces unintentionally.
- Electrostatic interactions: Variances in pH and ionic strength can affect protein charge, leading to unwanted binding to negatively or positively charged sites on the resin.
To minimize non-specific bindings, a few strategies can prove effective:
- Optimization of buffer conditions: Adjusting the buffer's pH and salt concentration can help maintain specific protein interactions while washing away contaminants.
- Blocking agents: Using agents that can saturate non-specific sites on the resin can also help displace unwanted proteins from binding.
- Careful selection of the host cell line: The choice of cell line used to produce the target protein can influence non-specific interactions. Some cell lines may yield proteins with a lower propensity for non-specific bindings due to their post-translational modifications.
"By acquiring a solid grasp on binding interactions, one can navigate the murky waters of non-specific bindings with greater confidence."
Column Fouling Issues
Another substantial challenge is column fouling. This refers to the gradual blockage of the column due to the accumulation of proteins and other biomolecules that are not eluted effectively during the purification process. Fouling can lead to a decrease in flow rates, increased back pressure, and in severe cases, complete blockage of the resin.
Problems of fouling may stem from:
- Aggregates and precipitates: Proteins can aggregate under certain conditions, leading to larger complexes that can clog the resin beads.
- Lipids and detergents: These substances can co-purify with proteins, further complicating the purification process and causing fouling.
To tackle column fouling, one might consider the following approaches:
- Regular cleaning cycles: Implementing cleaning protocols using specialized detergents or high salt buffers can help dislodge and remove foulants from the column.
- Alteration of flow rates: Reducing flow rates during the binding phase can minimize shear stress and decrease the likelihood of protein aggregation within the column.
- Use of pre-filters or filtration aids: Incorporating a pre-filter can help to catch larger particulates before they enter the column, thus preserving the integrity of the resin.
Recent Advancements and Future Directions
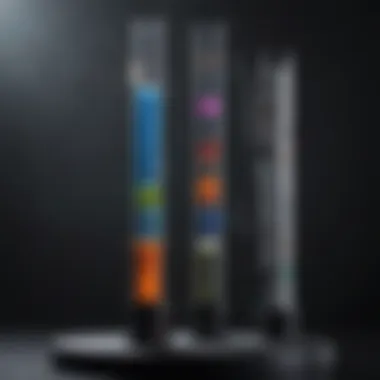
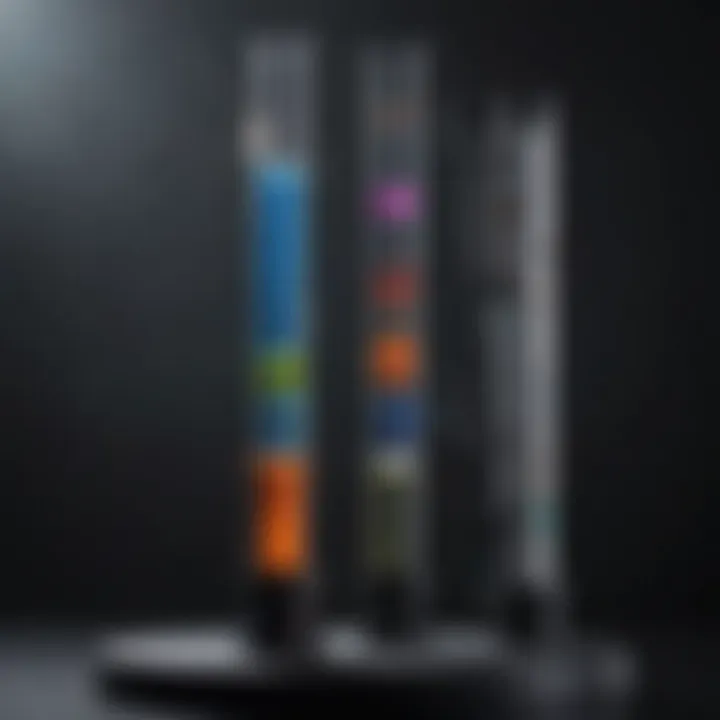
In the realm of biochemistry and protein purification, recent advancements and future directions embody the pulse of scientific innovation. The exploration of Protein A affinity columns has not stagnated; rather, it has been invigorated by cutting-edge research and technological breakthroughs. These advancements not only refine existing methodologies but also open up expansive avenues for future discoveries and applications. As practitioners and researchers grapple with increasing demand for high-purity proteins, understanding these evolving technologies is crucial.
Innovations in Column Design
Column design has witnessed a renaissance characterized by a focus on efficiency and yield. Traditional columns, often plagued by issues such as low throughput and lengthy processing times, are now being supplanted by novel designs that optimize flow dynamics and binding capacities.
- Modular Designs: Today’s columns are frequently modular, allowing researchers to customize setups based on specific needs. This approach enhances versatility, enabling users to adapt their systems without starting from scratch.
- Monolithic Columns: These have gained traction, where a single piece of porous material functions as the stationary phase. The benefits? Higher mass transfer rates and reduced backpressure, fostering more efficient separations.
- Microfluidic Systems: Innovation has carried over into micro-scale systems, capturing interest in both research and industrial settings. These systems allow for rapid purification processes with minimal sample requirements.
"Innovative column designs not only increase throughput but also ensure that protein interactions are more reliable and reproducible."
Additionally, surface modifications on column materials are enhancing the interactions specific to the desired target proteins, thereby improving overall purification efficiency. New binding capacities and specificities are opening up possibilities for dealing with more complex biological samples.
Emerging Technologies in Protein Purification
As the field evolves, emerging technologies are reshaping the landscape of protein purification.
- Artificial Intelligence: Employing AI in optimizing purification processes is becoming increasingly common. Algorithms can analyze data from previous runs to predict effective conditions, trimming down trial-and-error approaches.
- Continuous Flow Techniques: Moving away from batch processing to continuous flow methods dramatically streamlines the purification process. This constant feed allows for real-time adjustments and often leads to improved yield rates and consistency.
- Single-Use Technologies: These systems are designed for one-time use, minimizing cross-contamination risks. They not only facilitate compliance with standards but also reduce the time spent on cleaning and sterilization.
- Smart Monitoring Systems: The integration of IoT gadgets in purification setups is another revolutionary stride. They enable real-time monitoring of parameters like pH and conductivity, allowing for immediate adjustments and reducing the risk of process deviations.
In sum, the dialogue surrounding Protein A affinity columns is not merely about improving existing techniques—it's a reflection of a vibrant discipline continuously evolving with technological advancements. The focus now is not only on enhancing efficacy and efficiency, but also on anticipating future research needs and regulatory requirements. The journey doesn’t end here; it merely marks the beginning of an exciting chapter in protein purification.
Ending
In the realm of biochemical research, the significance of Protein A affinity columns cannot be understated. As a fundamental tool for protein purification, these columns offer unparalleled efficiency and specificity when it comes to isolating target proteins, particularly monoclonal antibodies. This article has thoroughly explored the various mechanisms underlying the interactions between Protein A and IgG, showcasing the importance of understanding these principles to enhance purification protocols.
One of the chief advantages of utilizing Protein A affinity columns is the high yield of purified proteins, which subsequently supports further applications such as drug development and therapeutic production. Understanding the nuances of column design, binding conditions, and elution strategies has been emphasized throughout the article, providing readers ample insights into optimizing these processes for various research scenarios.
Furthermore, the challenges associated with Protein A chromatography, such as non-specific bindings and column fouling, were addressed to equip practitioners with the knowledge to mitigate these issues. As the field continues to evolve with advancements in technology and methodologies, retaining a critical perspective on these challenges seems prudent for anyone invested in protein purification.
"Knowledge is power but only when it is applied effectively in practical settings."
This conclusion serves not only as a summary of the material discussed but also as a stepping stone toward future exploration in the fascinating landscape of protein purification technology. The fusion of traditional methods with emerging innovations creates a fertile ground for research endeavors aimed at enhancing the efficiency of protein purification.
Summary of Key Points
- Essence of Protein A Affinity Columns: Central to efficient protein purification, significantly impacting the efficiency of isolating monoclonal antibodies.
- Mechanistic Understanding: The interactions between Protein A and immunoglobulins is pivotal for optimizing purification protocols.
- Practical Challenges: Non-specific interactions and fouling issues necessitate informed practices for effective purification.
- Future Directions: Continuing advancements and innovative approaches will further refine the application of Protein A chromatography in research settings.
Implications for Future Research
Moving forward, the trajectory of Protein A affinity chromatography is bright, yet researchers face the ongoing challenge of improving the specificity and efficiency of these techniques. Exploring alternative ligands or composite materials may pave the way for even better purification methods.
Moreover, the integration of technological advancements such as real-time monitoring systems for binding and elution can provide a more dynamic approach to protein purification. Research into how environmental factors, like pH and ionic strength, can be systematically manipulated will undoubtedly enhance the efficiency of Protein A columns.
Thus, as the biochemistry field progresses, the development of more nuanced and sophisticated techniques will broaden the understanding of protein interactions and continue to support the creation of high-quality therapeutic proteins.
Further Reading and Resources
In the realm of biochemical research, deepening one's understanding of Protein A affinity chromatography stands as a vital endeavor. The significance of the Further Reading and Resources section cannot be overstated; it serves as a gateway for researchers and students alike, ushering them into a world buzzing with knowledge and innovation.
Diving into this topic, one can find that extensive literature provides background context, deepens understanding, and showcases advanced techniques that are crucial to successful applications in the lab. Engaging with this material helps in grasping the nuances of column design, the mechanics behind protein interactions, and optimization strategies that can dramatically improve purification outcomes.
Key Texts and Literature
A curated collection of essential texts creates a strong foundation for anyone exploring Protein A affinity columns. Some highly recommended reads include:
- "Affinity Chromatography: Methods and Protocols" – This book delves into various chromatography techniques, detailing protocols that are critical for effectively utilizing Protein A.
- "Protein Purification: Principles, High-Throughput Techniques, and Applications" – It provides insights into multiple purification methods while focusing on protein interactions.
- "Essential Techniques in Biochemistry and Molecular Biology" – A broad yet informative resource, this text covers fundamental techniques with applications in protein purification.
- "Introduction to Protein Structure" – Understanding protein structure is essential; this book serves as a primer on how structure impacts function and interaction.
These texts not only offer theoretical insight but also practical applications, ensuring readers acquire both knowledge and actionable skills.
Online Resources and Databases
The digital age provides a myriad of online resources that can significantly enhance knowledge and efficiency in the lab. Here are some noteworthy platforms:
- Wikipedia on Affinity Chromatography – A comprehensive overview of affinity chromatography, detailing its history and variations.
- Britannica on Proteins – A reliable source for understanding what proteins are and their importance in biological systems.
- Reddit: r/Biochemistry – A community where professionals share insights, ask questions, and discuss recent findings in biochemistry, including protein purification.
- Facebook Groups related to Biochemistry – Various groups are dedicated to discussions ranging from formal education to informal sharing of techniques among peers.
Utilizing these online databases not only enriches one's knowledge but also fosters community engagement that can lead to collaborative efforts in research and application.
Engaging with further readings and online resources stands as a cornerstone for anyone keen on mastering the intricate art of Protein A affinity chromatography. With so much information readily available, the path towards proficiency is clearer than ever.