RNA Sequencing Workflow: A Comprehensive Guide
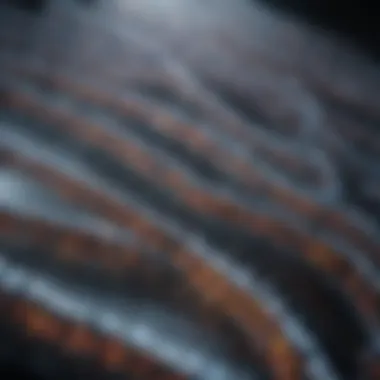
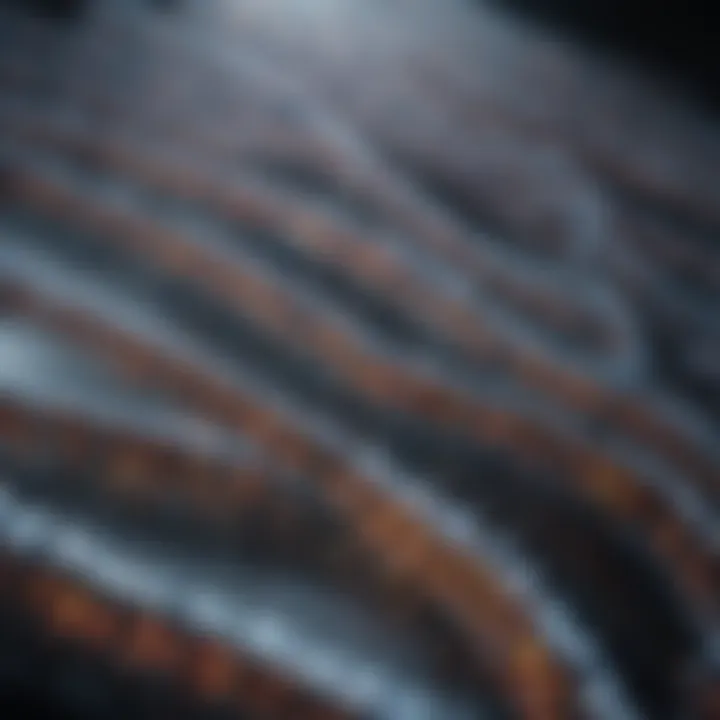
Intro
RNA sequencing has become an essential tool in genomic research, enabling profound insights into gene expression and regulation. Understanding how genes are expressed and regulated is vital for many scientific disciplines, including medicine, biology, and genetics. The workflow for RNA sequencing is intricate yet systematic, requiring careful planning and execution from sample preparation to data analytics.
This guide will take you through each phase of the RNA sequencing process, highlighting key steps and considerations. We will cover important methodologies, experimental designs, and the implications of the results garnered from this powerful technology. Designed for researchers, educators, and students alike, this overview emphasizes the significance of RNA sequencing in contemporary scientific inquiry.
Research Highlights
Key Findings
Research has established that RNA sequencing not only quantifies gene expression levels but also identifies novel transcripts, splicing variants, and non-coding RNAs. This capability provides a deeper understanding of the transcriptome, facilitating correlations between gene expression and phenotypic variations.
- RNA sequencing highlights dynamic changes in gene expression across various conditions.
- It uncovers previously hidden complexities of gene regulatory networks.
- The approach enables simultaneous analysis of protein-coding and non-coding RNA species.
Implications and Applications
The implications of RNA sequencing extend into several fields, from cancer research to developmental biology. It informs drug development, diagnostic strategies, and innovative therapeutic approaches. By elucidating gene functions and interactions, it also enhances our understanding of disease mechanisms and potential treatment avenues.
"RNA sequencing has transformed genomics by providing a comprehensive view of transcript dynamics, impacting both basic and applied sciences."
Methodology Overview
Research Design
The design of an RNA sequencing project typically follows a structured framework. Researchers must carefully select the sample type, RNA extraction method, and sequencing platform. Considerations include whether to focus on total RNA, polyadenylated RNA, or specific RNA subsets, which ultimately shapes the dataset generated.
Experimental Procedures
Experimental procedures involve several critical steps:
- Sample Preparation: Proper collection and stabilization of RNA are crucial for quality. Preservation methods such as using Trizol or RNA Later are often employed.
- RNA Isolation: Techniques like column-based purification or magnetic bead methods are commonly used to extract high-quality RNA.
- Library Construction: This involves preparing the RNA for sequencing, which includes fragmentation, reverse transcription, and adapter ligation.
- Sequencing: High-throughput platforms such as Illumina and Oxford Nanopore enable the sequencing of RNA libraries, producing vast amounts of data.
- Data Analysis: This phase includes alignment to a reference genome, quantification of gene expression, and differential expression analysis.
Through careful adherence to these detailed procedures, researchers can maximize the reliability and relevance of their RNA sequencing outcomes. Understanding each component of the workflow is crucial, as it directly influences the depth and quality of the insights derived from the research.
Prelude to RNA Sequencing
RNA sequencing, often referred to as RNA-seq, stands at the forefront of genomic research. This innovative technology enables a comprehensive understanding of gene expression and regulation. It allows scientists to analyze the complete RNA transcriptome of a cell or organism, providing insights that traditional methods lack.
Definition and Overview
RNA sequencing is a next-generation sequencing (NGS) technique that captures and sequences RNA molecules. The process involves several stages: sample preparation, library construction, sequencing, and data analysis. By sequencing RNA, researchers can identify which genes are active, the levels of expression, and how this varies under different conditions.
This method offers a high-throughput approach that can generate vast amounts of data in a short period. It has transformed the way we study gene expression, allowing for more precise and expansive exploration than ever before. With RNA-seq, it is possible to detect both expressed genes and non-coding RNAs, recognizing the full complexity of the transcriptome.
Importance of RNA Sequencing
The importance of RNA sequencing cannot be overstated. It plays a crucial role in numerous fields, including developmental biology, cancer research, and personalized medicine. By unlocking the mysteries of gene expression, RNA-seq contributes to:
- Gene Expression Profiling: Understanding how genes are regulated across different tissues and developmental stages is fundamental for biological research.
- Transcriptome Characterization: This offers detailed insights into the full range of RNA molecules produced in a cell, enhancing our grasp of cellular functions.
- Disease Research: RNA-seq serves as a tool to identify disease-related alterations in gene expression, aiding in the understanding of conditions such as cancer.
- Biomarker Discovery: By comparing expression patterns under various conditions, potential biomarkers for certain diseases can be identified, leading to improved diagnostics and therapeutics.
In summary, RNA sequencing has become an essential tool in modern biology. Its ability to portray a holistic view of gene expression drives research forward, providing a base for new discoveries and innovations.
Fundamental Principles of RNA Sequencing
The fundamental principles of RNA sequencing lay the groundwork for understanding how gene expression and regulation are studied at a molecular level. By focusing on the various types of RNA and the technologies used in sequencing, researchers can harness detailed insights into the complexities of cellular processes. Each element of RNA contributes uniquely to the overall goal of this sequencing workflow, providing crucial information and guiding research methodologies. Understanding these principles allows for informed decisions when choosing the appropriate techniques for specific studies.
Understanding RNA Types
RNA is not a homogenous entity; its different forms serve varied functions within the cell. The primary types include mRNA, non-coding RNA, and rRNA.
mRNA
Messenger RNA (mRNA) plays a central role in the flow of genetic information. It serves as the intermediary between DNA and protein synthesis, transmitting instructions from the gene. The key characteristic of mRNA is its ability to be translated into proteins. This attribute makes mRNA an essential focus in RNA sequencing, as it provides direct insights into gene expression changes under various conditions. Its abundance in cells makes it a popular choice for quantitation and analysis. The unique feature of mRNA is that it reflects the active gene expression at any given time, allowing researchers to explore cellular responses to stimuli or changes in disease states. An advantage of focusing on mRNA is its well-established extraction and sequencing protocols; however, it may not capture the intricacies of regulatory networks involving non-coding RNAs.
Non-coding RNA
Non-coding RNAs (ncRNAs) do not code for proteins; however, they play essential regulatory roles in gene expression. These include various types like microRNAs and long non-coding RNAs. The key characteristic of ncRNA lies in their ability to influence gene activity. Their increasing recognition in functional genomics makes them an important aspect of RNA studies. Non-coding RNAs are beneficial for research into gene regulation and cellular pathways. The unique feature of ncRNAs is their diverse mechanisms of action, which range from chromatin remodeling to mRNA destabilization. While they provide a broader context for gene expression, their characterization can be more complex and requires advanced sequencing techniques.
rRNA
Ribosomal RNA (rRNA) is a critical component of the ribosome, the molecular machine that synthesizes proteins. Its primary role is structural and functional but it is also indispensable for the translation of mRNA into proteins. The key characteristic of rRNA is its stability and abundance, making it an essential target for many sequencing approaches. As an integral part of ribosomes, rRNA can be used to assess the quality of RNA samples collected for sequencing. Because of its fundamental role in protein synthesis, focusing on rRNA can provide insights into the efficiency of translation in various biological contexts. However, its predominance in samples can sometimes overshadow the less abundant but functionally significant mRNA and ncRNA.
Sequencing Technologies
The choice of sequencing technology is another critical consideration in the RNA sequencing workflow. Each technology offers unique benefits and challenges that can impact data quality and the subsequent analysis.
Illumina
Illumina sequencing is the dominant technology used in RNA sequencing. It is known for its high throughput and accuracy, making it a preferred choice for large-scale gene expression studies. The key characteristic of Illumina is its ability to generate millions of short reads, providing a comprehensive view of the transcriptome. This technology's unique feature is its sequencing by synthesis, which allows for real-time data collection. Advantages include cost-effectiveness and the support of a wide range of applications, though the shorter read lengths can complicate the assembly of transcripts.
PacBio
Pacific Biosciences (PacBio) sequencing offers longer read lengths compared to other technologies. This aspect is crucial for accurately capturing full-length transcripts and providing more comprehensive transcriptome characterization. The key characteristic of PacBio lies in its Single Molecule Real-Time (SMRT) sequencing technology, which facilitates the analysis of complex transcripts without amplification. Its unique feature is the production of long reads, enabling better resolution of isoforms. While it excels in resolving transcript structures, it tends to be more expensive and can have higher error rates than other methods.
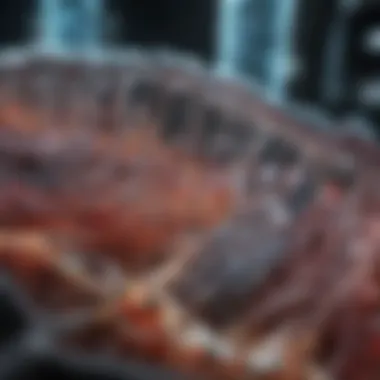
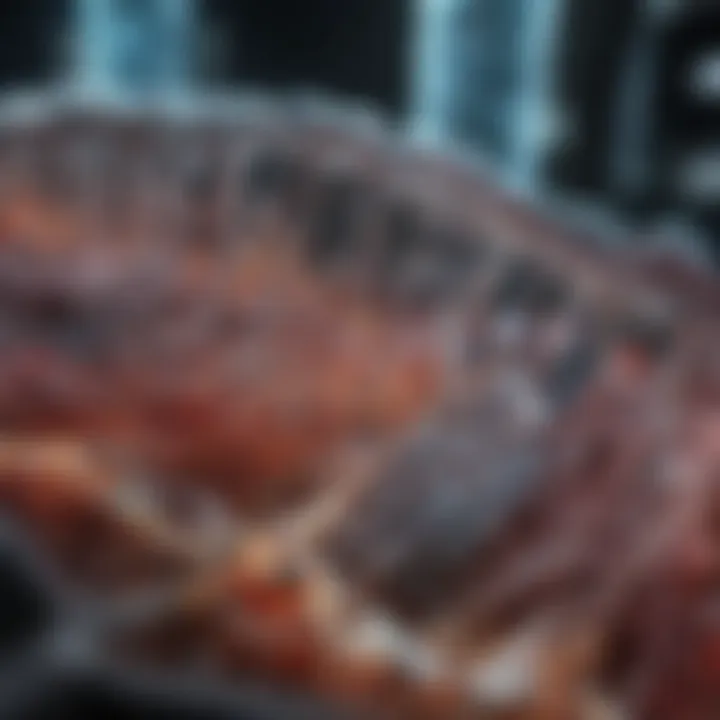
Ion Torrent
Ion Torrent technology is based on semiconductor sequencing, where changes in pH are detected as nucleotides are incorporated. The key characteristic of Ion Torrent is its rapid turnaround time for sequencing runs. This makes it advantageous in scenarios where quick results are necessary. The unique feature is its ability to perform real-time sequencing, providing instant access to data analysis. However, the accuracy may not match that of Illumina, potentially affecting the reliability of quantitative measurements in some applications.
Sample Preparation for RNA Sequencing
Sample preparation is a foundational step in the RNA sequencing workflow. The quality of RNA extracted from biological samples affects the outcome and reliability of the sequencing results. Proper preparation ensures that the RNA is intact and free from contaminants. Various factors can influence the efficiency of sequencing, making it crucial to adhere to best practices during this phase.
Tissue Collection and Storage
Tissue collection needs careful handling. Different types of biological tissues can have varied RNA stability, particularly in post-mortem samples. Timing is critical; rapid stabilization of the sample is essential to minimize degradation. Tissues should be stored at ultra-low temperatures using liquid nitrogen or stored in RNA preservation solutions to maintain sample integrity for downstream analysis. This method also avoids the loss of specific transcripts that may be more sensitive to environmental degradation.
RNA Isolation Techniques
Different techniques exist for isolating RNA, each with benefits and drawbacks. Two of the most commonly used methods include Phenol-Chloroform Extraction and Column-Based Methods, which have different efficiencies and uses depending on the sample type.
Phenol-Chloroform Extraction
Phenol-Chloroform Extraction is a classic method for RNA isolation. It involves the use of organic solvents to separate RNA from DNA and proteins effectively. One key characteristic of this method is its high yield, making it a favorable choice when dealing with challenging samples.
This method is beneficial because it allows for the extraction of high-purity RNA, which is crucial for accurate sequencing results. However, it can be hazardous due to chemical exposure, requiring strict adherence to safety protocols. One unique feature of this method is its adaptability; it can be modified to suit various sample types, though careful optimization is necessary to achieve efficient results.
Column-Based Methods
Column-Based Methods utilize silica membranes to isolate RNA through a series of wash and elution steps. This technique is known for its convenience and speed. It is increasingly popular in laboratories that require rapid processing. The key characteristic of column-based methods is their simplicity and ease of use, which reduces the likelihood of RNA degradation during isolation.
However, there can be limitations regarding the yield when using low-abundance samples. A unique advantage of this technique is that it minimizes contamination risks, maintaining sample integrity.
Quality Assessment of RNA
Quality assessment is vital following RNA isolation. Evaluating RNA quality ensures that samples are suitable for sequencing, as degraded RNA can lead to unreliable results.
NanoDrop Spectrophotometry
NanoDrop Spectrophotometry is a quick and reliable method for assessing RNA concentration and purity. This technique uses a small volume of sample for analysis and provides rapid feedback regarding the purity of RNA, indicating the presence of contaminants like proteins or phenol.
It is a beneficial choice for initial screening of RNA samples. However, it does not provide detailed information about RNA integrity. Its unique feature is the minimal sample volume requirement, which is helpful when working with limited material.
Bioanalyzer
The Bioanalyzer is another powerful tool for RNA quality assessment, providing detailed information about RNA integrity and size distribution. It uses microfluidics and dye staining to visualize the RNA, allowing for comprehensive analysis.
This method is advantageous as it gives a clear view of RNA quality, helping researchers identify potential issues before sequencing. However, it requires specialized equipment and can be cost-prohibitive, which may limit its accessibility.
In summary, sample preparation for RNA sequencing significantly impacts the overall workflow, influencing both data quality and experimental outcomes. Selecting appropriate techniques for RNA isolation and quality assessment ensures the generation of reliable and reproducible results.
Library Preparation
Library preparation is a crucial phase in RNA sequencing. It transforms the isolated RNA into a format suitable for sequencing machines. This process involves several intricate steps, including complementary DNA (cDNA) synthesis, amplification, and quality control. Each element plays a significant role in ensuring accurate and reliable sequencing results, which ultimately impacts the interpretations and applications of the data.
cDNA Synthesis
The first stage of library preparation is cDNA synthesis, where the RNA is converted into complementary DNA. This step is pivotal because DNA is more stable than RNA, making it easier to manipulate in subsequent processes. During cDNA synthesis, the enzyme reverse transcriptase is used to synthesize cDNA from the mRNA templates. The quality of the cDNA directly influences downstream analysis; therefore, it is critical to use high-quality reagents and maintain ideal reaction conditions.
The efficiency of this process can significantly affect the amount of cDNA produced. Several factors, including the integrity of the RNA and the specific reverse transcriptase enzyme used, can impact the synthesis. Hence, optimizing the reaction conditions is essential for obtaining sufficient cDNA yield for amplification and analysis. Moreover, incorporating unique barcodes can help label different samples, making it easier to multiplex several samples in one sequencing run.
Library Amplification and Purification
Following cDNA synthesis, amplification and purification steps are performed. Amplification uses polymerase chain reaction (PCR) to increase the amount of cDNA, facilitating adequate quantities for sequencing. Effective amplification results in sufficient DNA quantities needed for generating high-quality sequence data.
Purification of amplified cDNA is also essential. This process removes residual primers, enzymes, and other reagents used during amplification. Techniques such as magnetic bead-based purification or gel extraction are often employed. The goal is to achieve a clean library that minimizes potential contaminants that could interfere with sequencing. High-quality libraries result in more reliable reads and accurate representation of the original RNA.
Library Quantification and Quality Control
Once the library is prepared, quantification and quality control follow. This step assesses the concentration and size distribution of the final library. Accurate quantification is essential to ensure that the correct amount of the library is loaded onto the sequencer. Methods such as qPCR or fluorometric assays can provide reliable measurements of library concentration.
Quality control ensures that the library adheres to desired specifications. Techniques such as bioanalyzer or TapeStation can be employed to evaluate library size distribution and assess integrity. Quality metrics help researchers determine whether the prepared library is suitable for sequencing.
Effective library preparation improves the overall RNA sequencing workflow. Each step from cDNA synthesis to quality control is interconnected and requires careful attention to detail. Proper library preparation underpins successful RNA sequencing, setting the foundation for accurate data collection and meaningful analysis.
Sequencing Process
The sequencing process is a pivotal phase in RNA sequencing, determining both the quality and accuracy of the data obtained. This stage is essential for transforming the prepared libraries into usable sequence information that reflects the underlying gene expression profile. There are several important factors to consider during this process, including the types of sequencers used, the preparation of the libraries, and the approach to data collection.
Loading Libraries onto the Sequencer
Loading libraries onto the sequencer represents a critical step that must be executed with precision. Prior to loading, it is necessary to ensure that the libraries are of proper concentration and quality. This often requires the use of fluorometric methods, typically utilizing instruments like the Qubit, to quantify the concentration accurately.
It is essential to follow the specific guidelines provided by the sequencer manufacturer for library loading. For example, with an Illumina sequencer, the user must prepare a loading mixture that is optimized for the sequencer's specifications. Typically, this involves dilution of the libraries in a buffer solution to achieve the desired concentration suitable for sequencing. Once done, the libraries can be pipetted into the appropriate flow cell lanes. This process must be done carefully to avoid contamination or air bubbles that could affect the sequencing run.
Sequencing Workflow Execution
The execution of the sequencing workflow is where the magic happens. This step involves numerous automated processes in which the sequencer reads the nucleotide sequences that make up the RNA libraries. The workflow is often segmented into cycles of sequencing, each representing a round of base incorporation.
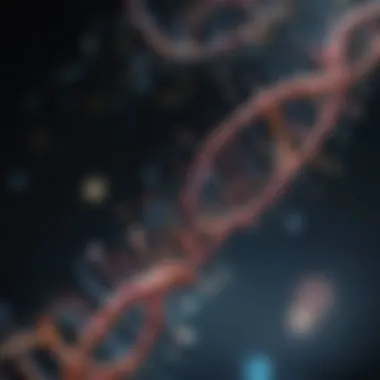
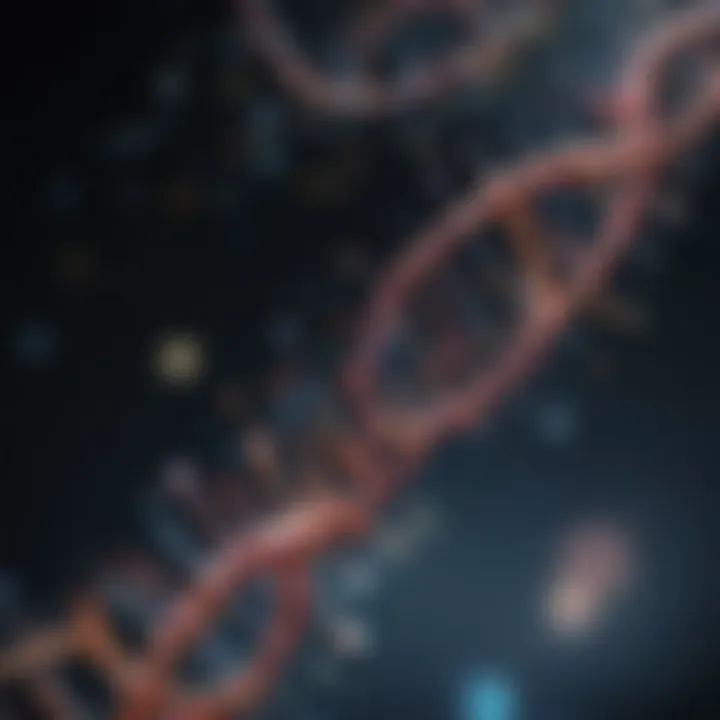
During this phase, high-throughput sequencers like those from Illumina use a technique called sequencing by synthesis. In this method, each cycle incorporates a labeled nucleotide complementary to the template strand. The emitted signals, captured by cameras on the sequencer, are converted into digital data for each read. This process can generate millions of reads in a single run, enabling extensive data collection.
Proper management of the sequencing workflow is crucial. Parameters such as read length, sequencing depth, and run time need to be optimized based on the specific goals of the experiment. For instance, a deeper sequencing depth might be necessary for rare transcripts, while shorter read lengths may suffice for high-abundance genes.
In summary, the sequencing process not only gathers data but also influences the subsequent analysis phases, shaping the overall results and interpretations.
Overall, understanding the sequencing process, from loading libraries to execution, is fundamental for generating reliable and actionable RNA sequencing data. This step impacts the capabilities of researchers in revealing the complexities of gene expression in various biological contexts.
Data Processing and Analysis
Data processing and analysis is a critical component of the RNA sequencing workflow. This stage encompasses converting raw sequencing data into meaningful biological insights. The importance of data processing lies in its ability to extract significant information from massive datasets generated during sequencing. A systematic approach to data processing helps in identifying gene expressions, alternative splicing events, and potential biomarkers associated with various conditions. Researchers must navigate challenges in data quality and variations due to biological factors.
Raw Data Generation
The first step of data processing involves raw data generation. Once the sequencer completes its run, it produces vast amounts of raw data represented in formats such as FASTQ. This data includes base calls and quality scores, ensuring each read's accuracy. Raw read files can be large, sometimes exceeding terabytes. Therefore, efficient data handling techniques are essential to manage storage and access without losing critical information.
Quality Control of Sequencing Data
Quality control is essential before any further analysis. Poor-quality data can lead to incorrect biological conclusions. Among the tools utilized for this purpose are FastQC and Trim Galore, each playing a unique role in ensuring data integrity.
FastQC
FastQC is a widely-utilized tool for assessing the quality of sequencing reads. Its primary function is to evaluate various aspects, including read length distributions, GC content, and duplication levels. FastQC quickly provides visualization of these parameters, which helps researchers identify potential issues early in the workflow. One significant characteristic of FastQC is its user-friendly output that allows rapid interpretation. This tool is a preferred choice in RNA sequencing data analysis because it helps eliminate low-quality reads before alignment.
FastQC is crucial for maintaining data integrity during the RNA sequencing workflow.
Trim Galore
Trim Galore is designed to improve the quality of sequencing data by trimming unwanted sequences from the ends of reads. It combines the functionality of Cutadapt and FastQC to retain high-quality data. The primary strength of Trim Galore lies in its ability to remove adapters and low-quality bases, which significantly enhances result accuracy. This tool is beneficial because it reduces the amount of data processed in subsequent steps, thus improving computational efficiency.
Read Alignment Techniques
After quality control, reads are aligned to a reference genome. This step is vital for identifying which genes are active under various conditions. STAR and HISAT2 are two widely-used algorithms in this context.
STAR
STAR is an aligner known for its speed and accuracy. It is particularly effective for mapping RNA-seq reads to a reference genome. STAR uses a novel algorithm that allows for efficient handling of large datasets. Its key characteristic is its ability to recognize splicing events, making it a favored choice for RNA sequencing projects. However, STAR requires sufficient memory and may struggle with datasets that exceed its limits.
HISAT2
HISAT2 is another robust read aligner that utilizes a fast and sensitive approach to align reads. Its unique feature is its ability to manage spliced reads by using a graph-based alignment method. This can provide a significant advantage when dealing with complex genomes. HISAT2 is popular due to its speed and lower memory requirements compared to some alternatives. Nonetheless, users must consider its sensitivity to input quality when aligning reads.
Quantification of Gene Expression
Quantifying gene expression involves determining the expression levels of active genes from aligned reads. This phase translates count data into functional insights about the biological process being studied. Accurate quantification is vital for downstream analyses and comparative studies. Multiple tools can perform this task, each with unique strengths depending on the specific requirements of the dataset. Proper computational tools and methods are essential to ensure that the results are both reliable and biologically significant.
Interpretation of Results
Interpreting results from RNA sequencing is critical in understanding gene expression and its biological implications. This process involves analyzing the data obtained from sequencing to derive meaning and insights related to cellular functions and behaviors. Accurate interpretation can influence research outcomes, allowing scientists to identify potential pathways involved in health and disease.
One of the key elements in interpreting RNA sequencing results is the biological significance of findings. This entails linking expression levels of genes to biological processes, understanding how these genes interplay in signaling pathways, and how their roles relate to phenotypic variations. Biologists strive to connect experimental data to physiological relevance, guiding hypotheses and future experiments.
Another important facet is comparative analysis. By comparing results from different conditions or experimental setups, researchers can dissect the differences in gene expression patterns. This approach allows for the identification of differentially expressed genes, which can be markers of disease states or response to treatment. Conducting comparative studies can also illuminate evolutionary changes and species-specific adaptations.
Key Takeaway: The interpretation of RNA sequencing data is a sophisticated process that requires a deep understanding of biology and statistical analysis.
Biological Significance of Findings
The biological significance of findings arises from the essential relationship between gene expression and cellular behavior. Each gene's expression level can have profound implications for cellular function, influencing overall health and disease states. Understanding which genes are upregulated or downregulated allows researchers to piece together the biological narrative from their data.
For instance, in cancer research, identifying overexpressed oncogenes can provide targets for therapeutic intervention. Likewise, understanding the downregulation of tumor suppressor genes can shed light on the mechanisms driving tumorigenesis. Furthermore, correlating gene expression with clinical outcomes is essential. By doing so, one can develop prognostic markers that may assist in patient management.
Comparative Analysis
Comparative analysis in RNA sequencing allows researchers to systematically evaluate how gene expression changes across different conditions. This could involve comparisons between healthy and diseased tissues, contrasts between treatment responses, or variations across different developmental stages.
A few key points to consider include:
- Differential Gene Expression: Utilize statistical methods to identify genes that show significant differences in expression levels.
- Biomarker Discovery: Identifying genes that are consistently expressed differently in specific conditions can lead to the discovery of novel biomarkers.
- Contextual Insights: Exploring how gene expression profiles shift can provide insights into biological pathways and mechanisms underlying diseases.
In summary, the interpretation of RNA sequencing results is multifaceted and requires thorough analytical approaches. By focusing on biological significance and engaging in comparative analysis, researchers can extract meaningful insights that advance the field of genomics.
Applications of RNA Sequencing
RNA sequencing provides valuable insights in various scientific areas. Its applications have become essential tools in genomics, molecular biology, and medical research. Understanding the diverse uses of RNA sequencing enhances the knowledge and practices of students, researchers, and professionals alike.
Gene Expression Profiling
Gene expression profiling involves measuring the activity of genes in different conditions. RNA sequencing allows researchers to quantify and compare the expression levels of thousands of genes simultaneously. This comprehensive view enables the identification of which genes are active under specific scenarios, such as development stages or environmental responses.
Benefits include:
- High sensitivity and specificity in detecting low abundance transcripts.
- Ability to discover novel transcripts and splice variants.
- Precise quantification of gene expression changes between samples.
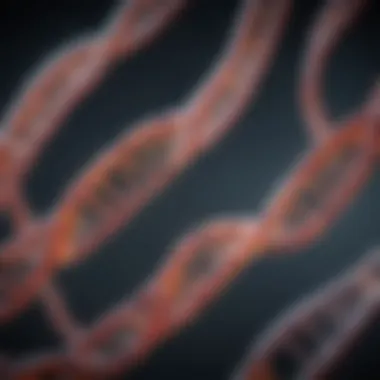
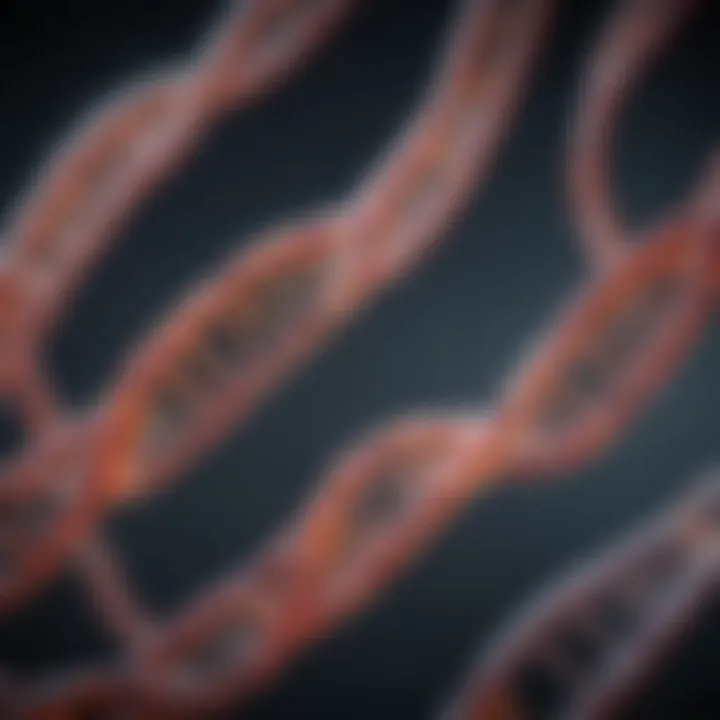
Such detailed profiling is instrumental in cancer research, where understanding gene expression differences between tumor cells and normal cells can guide treatment strategies.
Transcriptome Characterization
The transcriptome encompasses the entire set of RNA transcripts produced by the genome at any given time. RNA sequencing effectively captures the complexity of the transcriptome, including mRNA and non-coding RNA populations. This understanding is crucial for functional genomics applications, enabling a whole-genome perspective in gene regulation and expression.
Considerations for transcriptome characterization include:
- Assessing alternative splicing events which can affect protein diversity.
- Evaluating non-coding RNAs which play roles in gene regulation and cellular processes.
Such insights provide foundational knowledge for systems biology, enhancing our comprehension of cellular functions.
Applications in Disease Research
RNA sequencing has enabled significant advancements in disease research. By analyzing the transcriptomes of diseased versus healthy tissues, researchers can identify biomarkers, understand disease mechanisms, and explore therapeutic targets. This approach has been pivotal in cancer, neurological disorders, and infectious diseases.
Key aspects include:
- Discovery of disease-specific transcripts which may serve as potential diagnostic markers.
- Insights into the alterations in cellular pathways during disease progression.
The ability to discern these changes fuels the development of personalized medicine, customizing treatment based on individual gene expression profiles.
Biomarker Discovery
Biomarkers are biological indicators that signal a condition or disease state. RNA sequencing contributes extensively to biomarker discovery through its capacity to profile gene expression and identify changes associated with specific diseases. This application paves the way for developing diagnostic tools and therapeutic interventions.
Important points in biomarker discovery are:
- Identification of stable RNA molecules that can be detected in various biological fluids.
- Validation of biomarkers through comprehensive computational analyses and wet lab experiments.
This data-driven process holds promise for improving patient outcomes and advancing precision medicine strategies.
Challenges and Limitations
The field of RNA sequencing has revolutionized our understanding of gene expression and regulation. However, this complex methodology does not come without its challenges and limitations. These aspects are crucial for researchers to understand, as they can significantly affect the outcomes of RNA sequencing experiments. Addressing these challenges is necessary to ensure reliable and reproducible results, which is vital for any scientific research.
Technical Challenges
One of the primary technical challenges in RNA sequencing is related to sample quality. The integrity of the RNA prepares for sequencing can influence the generation of accurate data. Contamination during the isolation and preparation phases can lead to misleading results. For instance, the presence of genomic DNA in samples can compromise the accuracy in quantifying mRNA levels. Thus, using proper techniques for RNA isolation and rigorous quality control is paramount.
Another technical aspect is the choice of sequencing platform. With various technologies like Illumina, PacBio, and Ion Torrent, each has its inherent limitations concerning read length, error rates, and throughput. Selecting the wrong platform for a specific research question can potentially lead to inadequate data. Additionally, as sequencing depth increases, so does the cost and complexity involved in data handling and analysis.
Finally, processing and analyzing vast amounts of sequencing data also present technical hurdles. Proper software tools must be employed for data alignment and quantification, and ensuring that these tools are suited for the specific type of RNA data can be complicated.
Biological Variability
Biological variability is another critical challenge in RNA sequencing. Variations among biological specimens, even within the same species or tissue type, can significantly affect gene expression profiles. Factors such as age, sex, environmental influences, and genetic background must be considered when interpreting data. These variables can lead to confounding results, which complicate comparisons across studies.
Moreover, the dynamic nature of RNA itself means that gene expression can fluctuate over time or in response to external stimuli. This variability necessitates careful experimental design, often requiring replicate samples or time-course studies to achieve more accurate and robust conclusions.
Understanding both technical challenges and biological variability is essential for designing informative RNA sequencing experiments.
These challenges are not merely obstacles but also opportunities for researchers to refine their methodologies. By proactively addressing these issues, scientists can enhance the quality and interpretability of their RNA sequencing findings.
Future Directions in RNA Sequencing
The field of RNA sequencing continues to evolve rapidly. Innovations in technology and methodology promise to enhance our understanding of transcriptomics. As RNA sequencing becomes increasingly accessible, it opens new avenues for research across various scientific disciplines. Understanding the future directions in this field is essential for students, researchers, and professionals. It allows them to stay updated and apply the latest techniques to their work.
Innovations in Sequencing Technology
The pace of change in sequencing technology is astounding. New platforms are being developed that increase throughput and accuracy. For instance, single-cell RNA sequencing has emerged as a powerful tool. This method allows researchers to analyze gene expression at the individual cell level, revealing variability that bulk sequencing cannot provide. Recent advances, such as the introduction of long-read sequencing technologies, can capture full-length transcripts, consequently improving the detection of isoforms and novel genes.
Some of the key innovations include:
- Rapid sequencing methods: Techniques that reduce the time needed for sequencing have gained traction. Devices like the Oxford Nanopore MinION allow for real-time analysis, which is beneficial in many applications, like pathogen detection.
- Improved data analytics: Enhanced computational tools enable more sophisticated analysis of the vast data generated. Algorithms that better manage noise in sequencing data improve the reliability of results.
- Cost reduction: As technology advances, the cost of sequencing decreases, making it more accessible to a wider range of researchers and institutions.
These innovations not only improve the efficiency of conducting research but also enhance the quality of data. Emerging technologies will facilitate a deeper understanding of gene expression and regulation.
Integration with Other Omics Data
As the field progresses, integrating RNA sequencing data with other omics data becomes increasingly important. This integrated approach provides a more holistic view of biological systems. Combining genomic, transcriptomic, proteomic, and metabolomic data allows researchers to explore the complex interactions within cells.
Benefits of this integration include:
- Comprehensive insights: By analyzing multiple layers of biological information, researchers can identify correlations that might not be visible through singular omics data.
- Disease understanding: Integration can lead to revelations about disease mechanisms. For example, some studies combine transcriptomic data with proteomic analysis to better understand cancer biology. This can inform targeted therapies.
- Systems biology applications: By pooling various omics data, researchers can create predictive models of complex biological processes. This is essential for advancing personalized medicine.
In summary, the future of RNA sequencing is bright, with numerous innovations on the horizon. Sequencing technology is becoming faster and more accurate. Integration with other omics is paving the way for breakthroughs in biomedical research. Keeping pace with these trends is crucial for any researcher involved in genomics.
Finale
In any comprehensive exploration of RNA sequencing, the conclusion holds significant weight. It reinforces the key themes and findings discussed in the article. This section serves as a reflection on the cumulative insights gained throughout the RNA sequencing workflow. The necessity of reiterating the importance of understanding RNA sequencing cannot be overstated. It provides clarity on gene expression and regulation, two critical components in molecular biology.
The benefits of RNA sequencing are diverse. For researchers and practitioners, it emphasizes the practical applications in various fields such as genomics, personalized medicine, and disease research. Moreover, it serves as a reminder of the continuous evolution of sequencing technology and methods, which is paramount for advancing biological research.
Considerations about RNA sequencing also deserve attention. The intricate nature of RNA, the technical challenges involved, and the biological variability can influence study outcomes significantly. Thus, strategic planning and robust methodologies are essential components that must not be overlooked.
Summary of Key Points
- Importance of RNA Sequencing: Recognizes RNA sequencing as a pivotal tool for understanding gene expression.
- Workflow Overview: Outlines step-by-step processes, from sample preparation through to data analysis.
- Technological Advances: Highlights innovations that are shaping RNA sequencing practices.
- Applications in Science: Discusses real-world implications such as gene profiling and biomarker discovery.
- Challenges: Addresses both technical and biological hurdles faced during RNA studies.
This conclusion encapsulates the essence of RNA sequencing—its relevance, applications, and the need for meticulous execution in handling the intricate biological data it provides. In a rapidly advancing field, keeping abreast of developments and methodologies will only enhance the impact of RNA sequencing in scientific exploration.