Mechanisms of Converting Solar Power into Electricity
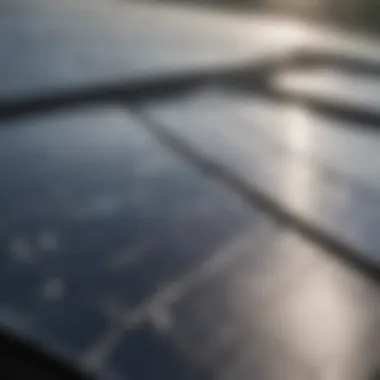
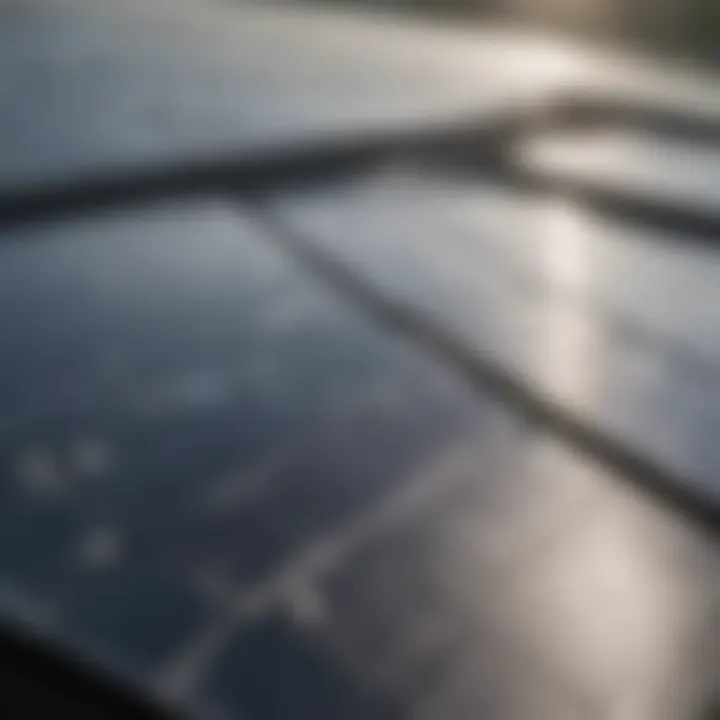
Intro
The quest for sustainable energy sources is becoming increasingly urgent as we confront the realities of climate change and dwindling fossil fuel reserves. Solar power stands as one of the most promising avenues for renewable energy conversion. Understanding how solar power can be effectively transformed into electricity is essential for both the advancement of technology and the reduction of our carbon footprint. This article explores the foundational mechanisms involved in this transformation, focusing on photovoltaics, inverters, energy storage methods, and the associated challenges we face.
Research Highlights
Key Findings
- Photovoltaic Fundamentals: Photovoltaics is based on the photoelectric effect, where photons collide with semiconductor materials, leading to the generation of electric current. This transformation is central to solar power technology.
- Types of Solar Panels: Different types of solar panels, such as monocrystalline, polycrystalline, and thin-film, each possess unique properties and efficiencies, impacting their effectiveness in various applications.
- Role of Inverters: Inverters play a crucial role in converting the direct current generated by solar panels into alternating current, which is the form of electricity typically used in homes and businesses.
- Energy Storage Needs: Effective energy storage solutions, such as lithium-ion batteries, are critical for managing the intermittent nature of solar power, allowing for energy use even when sunlight is not available.
Implications and Applications
The insights gained from understanding solar power conversion mechanisms have far-reaching implications. The global shift toward solar energy requires advancements in technology and grid integration. Improved solar technologies can foster energy independence, reduce greenhouse gas emissions, and create new economic opportunities in renewable energy sectors.
"The future of energy is not just about producing it; it's about harnessing natural sources in the most effective and sustainable ways."
Methodology Overview
Research Design
A multifaceted approach characterizes the study of solar energy conversion. This involves both theoretical frameworks and practical experiments aimed at improving efficiencies and innovations in solar technologies.
Experimental Procedures
Rigorous testing of solar panels under varying conditions informs understanding of their performance. Experiments consider factors like sunlight intensity, panel angle, and temperature, aiding in the development of optimized solar solutions.
Prolusion to Solar Power
Solar power represents a clean and renewable energy source that can play a pivotal role in addressing global energy demands. As traditional fossil fuels are tackled for their harmful environmental impacts, the evolution of solar technology offers paths toward sustainable energy solutions. Utilizing solar power to generate electricity has significance beyond mere technical processes; it encompasses economic, ecological, and social considerations that merit thorough exploration.
One of the most notable benefits of solar power is its sustainability. Unlike finite carbon-based energy resources, sunlight is abundant and inexhaustible, making solar power a cornerstone for a greener future. Additionally, solar power can enhance energy independence for nations and communities, reducing reliance on imported fuels and bolstering domestic job markets through the manufacture and installation of solar energy systems.
With the current global focus on climate change, solar energy serves as a crucial tool for reducing carbon emissions and promoting cleaner air. The advancements in technology are driving the efficiency and affordability of solar systems, making them more accessible to wider audiences.
Furthermore, the integration of solar power into existing energy grids denotes a significant transformation in energy consumption patterns. The ongoing research and innovations in solar technologies amplify its appeal by addressing issues such as energy storage and grid integration, which are vital for managing supply and demand effectively.
In summary, understanding solar power is essential for comprehending how it contributes to the broader context of renewable energy and its potential to mitigate climate change. Engaging with this subject allows stakeholders to recognize the powerful implications that harnessing solar energy could have on our planet's future.
Definition of Solar Power
Solar power refers to the energy derived from sunlight, which can be converted into usable electricity. This conversion occurs primarily through photovoltaic technology, where solar panels capture sunlight and generate electricity through the photovoltaic effect. Essentially, solar power transforms an abundant natural resource into a practical means of meeting energy needs.
Importance of Renewable Energy
The relevance of renewable energy like solar power cannot be overstated. As climate concerns mount, renewable sources are increasingly recognized as an essential alternative to fossil fuels. Their importance includes:
- Environmental Benefits: Lower greenhouse gas emissions, contributing to improved air quality and health outcomes.
- Energy Security: Reduced vulnerability to energy market fluctuations and geopolitical conflicts.
- Economic Opportunities: Job creation within the renewable energy sector as more investments flow into sustainable technologies.
- Innovative Solutions: Ongoing advancements that improve efficiency and lower costs, making renewable energy more viable for widespread adoption.
Basic Principles of Electricity Generation
Understanding the basic principles of electricity generation is crucial for grasping how solar power systems function. This section outlines key concepts that serve as the foundation for converting solar energy into electricity. Proficiency in these principles aids in recognizing the benefits of renewable energy and the efficient designs of solar power implementations.
Understanding Electricity
Electricity is primarily the flow of electrical charge, typically through conductors such as wires. The generation of electricity can be divided into two main types: direct current (DC) and alternating current (AC).
- Direct Current (DC) is a unidirectional flow of electric charge. Most solar panels produce DC electricity, as the photovoltaic cells generate it directly from sunlight.
- Alternating Current (AC) switches direction periodically. This is the form of electricity we commonly use in homes and businesses. Conversion from DC to AC is a significant step in the solar energy process, often performed by inverters.
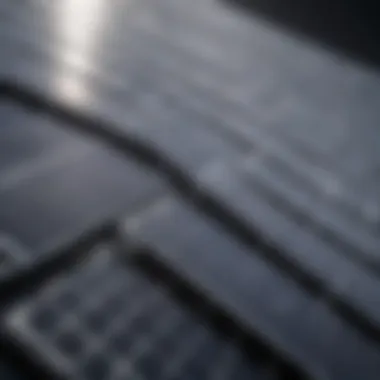
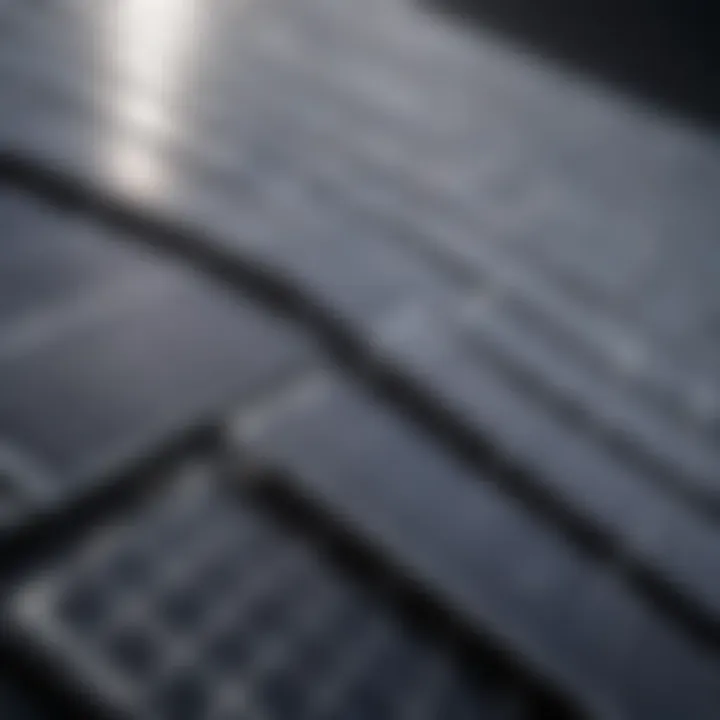
"Understanding these different forms of electricity is vital for anyone involved in energy solutions. It narrows down the varied processes that solar power must undergo to deliver usable energy."
Electricity generation relies on fundamental principles derived from physics, notably Ohm's Law and the concepts of voltage, current, and resistance. These components interact to dictate how energy flows through a system. This understanding contributes to optimizing the performance of solar power systems.
Energy Conversion Processes
Energy conversion processes are the methods through which energy is transformed from one form to another. In the context of solar power generation, the conversion primarily involves turning light energy from the sun into electrical energy. This transformation is achieved through several steps:
- Absorption of Solar Energy: Photovoltaic cells absorb sunlight and utilize it to excite electrons, resulting in the generation of electron-hole pairs.
- Generation of Electrical Current: The movement of these excited electrons creates a flow of electrical current.
- Direct Electricity Usage or Storage: The current generated can either be used directly to power devices or stored in batteries for future usage.
- Inversion if Necessary: If the generated electricity is in DC form, inverters convert it into AC for compatibility with household appliances.
These energy conversion processes highlight the complexity and efficiency of solar power systems. They not only account for the generation of electricity but also the integration of energy storage solutions that enable the use of solar power beyond daylight hours. Understanding these processes is essential for professionals in the energy sector, as they navigate advancements in technology and aim to maximize output from solar installations.
Photovoltaic Technology
Photovoltaic technology is a pivotal component in the conversion of solar power into electricity. It embodies the processes and methodologies responsible for harnessing sunlight and transforming it into usable electrical energy. Understanding this technology is essential because it lays the groundwork for various applications, from residential energy solutions to large-scale solar farms. Key benefits include the reduction of dependence on fossil fuels and increased sustainability. However, consideration must be given to various factors like efficiency and cost, which are crucial for optimizing solar energy deployment.
How Photovoltaics Work
Photovoltaics operate based on the photovoltaic effect, which involves the conversion of light into electricity at the atomic level. When sunlight hits a photovoltaic cell, it excites electrons, allowing them to flow and generate electricity. The basic building block of this technology is the semiconductor material, commonly silicon. Silicon cells absorb photons from sunlight, which gives enough energy to knock electrons loose, consequently creating an electric current. This process is controlled via specific layers in the cell that generate electric fields, pushing the free electrons toward conductive paths, which leads to electricity generation that can be captured for external use.
Types of Photovoltaic Cells
Different types of photovoltaic cells each have their distinct characteristics and applications, thereby contributing uniquely to solar power conversion.
Monocrystalline Solar Cells
Monocrystalline solar cells are known for their high efficiency and superior space efficiency. They are made from a single continuous crystal structure, allowing more electrons to flow freely, which results in higher energy generation per square meter. This characteristic makes them an excellent choice for applications where space is limited, like residential rooftops. However, they tend to come at a higher price point compared to other types. The unique feature of their uniform appearance indicates a higher purity level in silicon, which leads to fewer impurities. Their main disadvantage is that they produce less energy in shaded conditions compared to other types of cells.
Polycrystalline Solar Cells
Polycrystalline solar cells are manufactured using multiple silicon crystals, which lowers production costs. This cost-efficiency is their key advantage and makes them a popular choice for larger installations. They are slightly less efficient than monocrystalline cells, converting around 15-20% of solar energy into electricity. The appearance of these cells is characterized by a speckled blue color due to the multiple crystals. While they are more affordable, they do take up more space to produce the same amount of energy as monocrystalline cells. This slightly lower solar conversion efficiency can limit their use in highly space-constrained applications.
Thin-Film Solar Cells
Thin-film solar cells are lightweight and flexible, made by depositing photovoltaic material onto surfaces like glass, plastic, or metal. Their lightweight nature allows for a wide range of applications, including portable solar devices and building-integrated photovoltaics. They are less efficient than both monocrystalline and polycrystalline cells, typically averaging around 10-12%, but their adaptability is a significant advantage. These cells can be integrated into various structures without the need for significant alteration. A disadvantage is that they require more space for installation in order to generate equivalent power outputs as the other types, which could be a limiting factor in certain locations.
Solar Panel Assembly
The assembly of solar panels brings together various elements, including the photovoltaic cells, glass, and framework. The design aims to protect the cells while maximizing exposure to sunlight. First, photovoltaic cells are arranged in a grid-like format and encapsulated, often with a protective layer of glass. This assembly is critical, as it impacts overall performance and durability. Proper assembly ensures that panels have a long lifespan and withstand environmental stresses. Depending on the application, solar panels can be mounted using fixed mounts or advanced tracking systems that follow the sun. The choice of assembly method directly influences the efficiency and effectiveness of the solar power system.
Components of Solar Power Systems
Components of solar power systems play a crucial role in efficiently converting solar energy into usable electricity. Understanding these components not only enhances our appreciation of solar technology but also informs potential users about the options available to optimize their solar energy systems. Each element of these systems contributes to harnessing sunlight, converting it, and ensuring that the generated power fits into the grid or serves local demand.
Inverters and Their Role
Inverters are essential for converting the direct current (DC) produced by solar panels into alternating current (AC), which is the form of electricity most frequently used in homes and businesses. They facilitate the integration of solar power into existing electrical grids and make the energy produced by solar panels usable for everyday appliances. The performance and efficiency of an inverter directly affect the overall energy output of the solar power system.
Inverters come in various types, including string inverters, microinverters, and power optimizers. Choosing the correct inverter type can enhance energy yield, offer monitoring capabilities, and ensure better performance in shaded conditions or challenging environmental factors.
Mounting Systems for Solar Panels
Fixed Mounts
Fixed mounts are among the most basic and widely used mounting systems for solar panels. These systems keep the solar panels stationary, oriented generally toward the sun's trajectory. One of the key characteristics of fixed mounts is their simplicity which makes them easy to install and maintain.
The unique feature of fixed mounts is that they do not track the sun's movement, leading to fewer mechanical parts involved. This simplicity translates into lower costs for both installation and maintenance. However, a significant disadvantage of fixed mounts is that they only maximize energy production for certain conditions, often leading to lower overall efficiency compared to tracking systems.
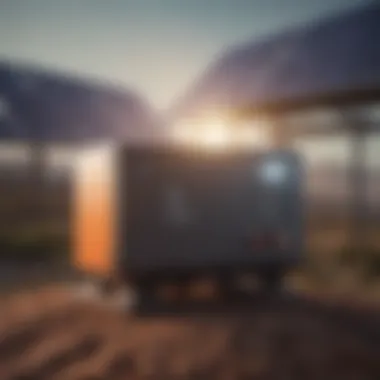
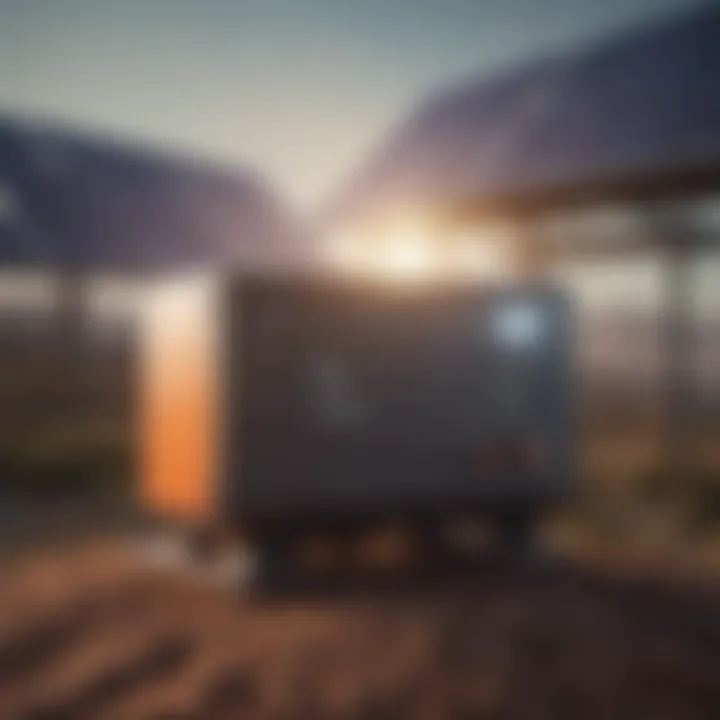
Tracking Systems
Tracking systems enable solar panels to follow the sun's path across the sky. This capability significantly enhances energy capture by maintaining optimal angles throughout the day. The key characteristic of tracking systems is their ability to adjust positioning based on the sun's movement, resulting in a potential increase in energy output by as much as 25 to 40% compared to fixed mounts.
However, tracking systems come with their complexities. They typically require more maintenance and are more expensive to install due to the additional mechanical components. The unique feature of these systems lies in their dynamic response to daily solar patterns, providing notable advantages in energy generation, yet they also pose challenges in terms of cost and reliability in extreme weather conditions.
Energy Storage Solutions
Energy storage solutions are integral for managing solar power reliability and efficiency. They allow excess energy generated during peak sunlight to be saved for later use, thus reducing reliance on the grid. This system effectively smoothes out the inconsistencies of solar energy outputs, especially during periods of high demand or bad weather.
Batteries
Batteries are the most recognized form of energy storage in solar power systems. Their ability to store energy for later use makes them a popular choice among residential and commercial solar installations. Lithium-ion batteries, in particular, have become the dominant technology, attributed to their high energy density and extensive cycle life.
A significant characteristic of batteries is their capacity; larger batteries can store more energy, improving system reliability during periods of low solar production. However, costs associated with advanced battery technologies and their limited lifespan can be noted as disadvantageous, necessitating regular replacements and maintenance.
Grid Integration
Grid integration refers to the connection of solar power systems to existing power grids. This allows for the export of excess energy generated by solar installations, providing financial benefits through net metering. A key characteristic of grid integration is flexibility; it allows users to access power from the grid when solar energy is insufficient and send excess energy back when production exceeds personal or local needs.
While grid integration offers numerous benefits, it is not without challenges. Infrastructure compatibility, regulatory barriers, and the need for smart grid technologies can complicate effective integration. Despite these challenges, the potential for improved energy production and sustainability makes grid integration a critical aspect of modern solar power systems.
"The integration of various components in solar power systems is vital for maximizing energy efficiency and taking advantage of the abundant solar resource available."
Efficiency of Solar Power Systems
The efficiency of solar power systems is a cornerstone of the discussion surrounding the conversion of solar energy into electricity. In an era where energy demands are escalating and the climate crisis looms large, understanding what affects the efficacy of solar power systems is crucial. High efficiency means more power derived from a similar amount of sunlight, leading to optimized energy production and reduced costs per unit of energy generated.
Moreover, efficient systems enhance the overall feasibility and adoption of solar power. Increasing efficiency translates to less space needed for solar panels and minimizes the financial investment required for installation and maintenance. It is not merely a technical statistic but also a measure of how harnessing solar energy can effectively contribute to global energy solutions.
Factors Influencing Efficiency
The efficiency of solar power systems depends on several intertwined factors:
- Quality of Materials: The materials used in photovoltaic cells play a critical role in determining their efficiency. For instance, monocrystalline solar cells typically offer higher efficiency rates than their polycrystalline counterparts.
- Temperature: Solar panels operate efficiently within an optimal temperature range. Higher temperatures can decrease the output, necessitating understanding of climate effects on performance.
- Solar Irradiance: The amount of sunlight striking the panels is fundamental. Areas with high solar irradiance yield more electricity compared to those with lower sunlight exposure.
- Angle and Orientation: Proper alignment of solar panels can significantly enhance energy capture. Panels facing the sun directly will generally perform better.
- Shading: Even partial shading from trees or buildings can drastically reduce the operational efficiency of a solar array. It is vital to assess shading during installation.
These elements intertwine to create a complex web of interactions. By optimizing them, manufacturers and users alike can improve the overall efficiency of their solar systems.
Measuring Solar Panel Efficiency
Measuring the efficiency of solar panels involves analyzing how effectively they convert sunlight into electricity. The general formula for measuring efficiency is:
Where power output refers to the electricity produced under standard test conditions, while power input is the solar energy incident on the panel during that same period.
Here are a few common metrics used in evaluating efficiency:
- Standard Test Conditions (STC): These conditions include a solar irradiance of 1000 W/m², a panel temperature of 25°C, and an air mass of 1.5. Testing under these conditions helps standardize comparisons.
- Temperature Coefficient: This indicates how much a panel's efficiency drops as temperatures rise. This aspect is vital for installations in hotter climates.
- Performance Ratio (PR): The performance ratio measures the quality of the solar system’s installation and management. It is calculated as the ratio of actual energy produced to the expected energy production, showing how well the system operates relative to its potential.
Each of these aspects provides valuable insights into the functionality and viability of solar technologies, aiding consumers and industry professionals in making informed decisions.
Advancements in Solar Technology
The field of solar technology is witnessing rapid advancements that significantly enhance the efficiency and applicability of solar power systems. This evolving landscape is crucial for optimizing solar energy conversion processes. As solar power becomes increasingly integral to our energy mix, these innovations contribute to greater reliability and lower costs, making renewable energy more feasible for widespread adoption.
Emerging Solar Technologies
Emerging solar technologies are at the forefront of improving how solar power is harvested, converted, and utilized. These include innovative concepts like solar skins and building-integrated photovoltaics (BIPV). Such advancements merge functionality with aesthetics.
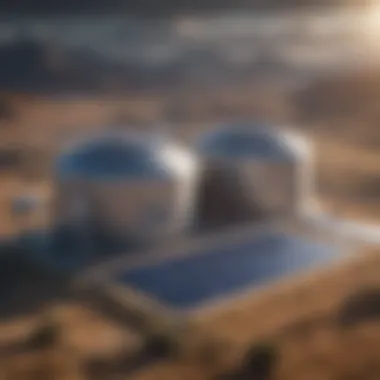
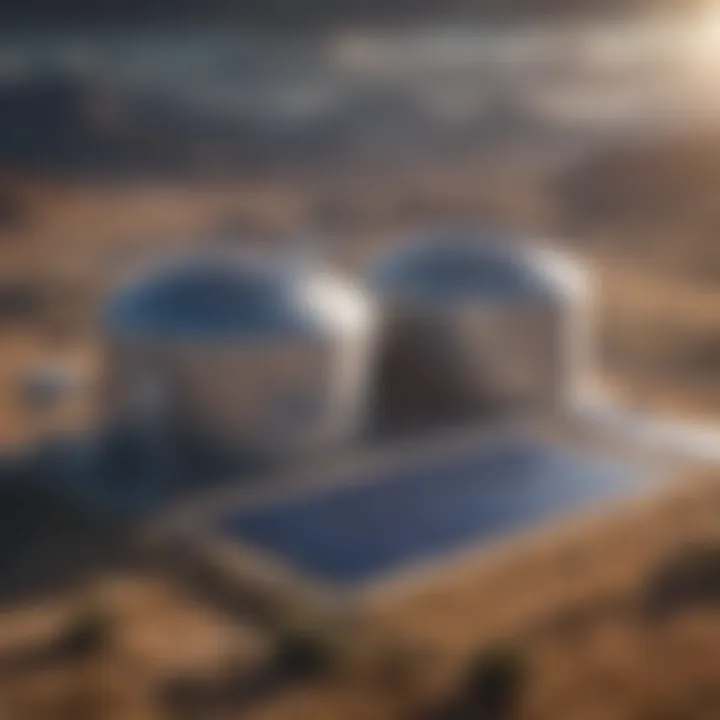
- Solar Skins allow for customizable solar panel designs, enabling homeowners to maintain aesthetic appeal while generating electricity.
- BIPV integrates solar cells into building materials like windows and roofs, reducing the need for traditional solar setups. This creates opportunities for energy generation in structures that may not have dedicated roof space.
Another noteworthy innovation is the development of perovskite solar cells. Perovskites offer a higher efficiency potential at a lower cost compared to traditional silicon cells. Their properties allow for easier manufacturing and integration into various environments. This could potentially revolutionize solar harvesting in both residential and commercial sectors.
"Emerging technologies in solar power can lead to a more decentralized and diverse energy system."
Innovations in Materials
The materials used in solar technology have a direct impact on efficiency, durability, and cost. Recent innovations have focused on enhancing the performance and longevity of solar panels.
- Bifacial solar panels, which can capture sunlight on both sides, have emerged as a popular option, as this increases energy production without a proportional increase in footprint.
- Advanced materials like graphene and transparent conductive oxides are being researched for use in new solar cells. These materials may offer improved electrical conductivity while maintaining transparency, which is vital for applications such as windows or surfaces that require visibility.
In addition, the integration of nanotechnology in solar cells has opened new avenues for efficiency improvements. By manipulating materials at the nanoscale, researchers aim to reduce energy loss and enhance light absorption. Though many of these technologies are still in the developmental stage, their successful implementation could dramatically influence the solar industry's future.
Challenges in Solar Energy Adoption
The adoption of solar energy is not without its challenges. Understanding these obstacles is crucial for stakeholders in the renewable energy sector, as they influence the implementation and scaling of solar technologies. Solar energy is recognized as a clean and sustainable power source, but the various challenges associated with it can hinder progress. This section will explore two major issues: the intermittency of solar power and the environmental impact of manufacturing solar panels.
Intermittency of Solar Power
One of the significant challenges in solar energy adoption is its inherent intermittency. Solar power generation is highly dependent on weather conditions and time of day. During cloudy days or nighttime, energy production drops significantly. This variability poses a problem for grid reliability and necessitates the need for backup systems.
To address this issue, energy storage solutions, such as batteries, play a vital role. They allow excess energy produced during sunny periods to be stored and used during times of low production. However, the current technology for energy storage is not yet fully capable of meeting all users' demands efficiently.
Additionally, integrating solar power into existing energy grids requires sophisticated management systems. These systems must balance generation and consumption in real time, which can be complex and costly to implement. Recognizing and investing in responsive grid technology is important for improving the reliability of solar energy.
Environmental Impact of Manufacturing
The production of solar panels also raises environmental concerns. The process involves the use of various raw materials, some of which can be harmful to the environment. For example, the extraction of silicon, which is a fundamental component in most photovoltaic cells, can result in habitat destruction. Furthermore, the manufacturing process itself can contribute to air and water pollution if not managed properly.
Efforts are underway to mitigate these impacts. Recycling solar panels can reduce waste and recover valuable materials. Research into alternative materials that have a lower environmental footprint is also being pursued. For instance, perovskite solar cells are emerging as a potential option with less environmental impact.
"By addressing the challenges associated with solar energy adoption, we can unlock its full potential as a key player in sustainable energy solutions."
Future of Solar Power
The future of solar power plays a crucial role in understanding the ongoing evolution of energy generation. Advances in technology are reshaping how we harness energy from the sun. As concerns about climate change grow and fossil fuel resources deplete, the transition towards renewable energy sources like solar power enfolds as an imperative. The integration of solar power into global power grids reduces dependency on nonrenewable sources, promoting sustainable practices. The benefits of solar energy chiefly include its cleanliness and accessibility.
Predicted Trends in Solar Technology
Innovations in solar technology are continually reshaping the landscape of electricity generation. Some notable trends expected to shape the future include:
- Bifacial Solar Panels: These panels capture sunlight on both sides, improving efficiency by maximizing energy capture on reflective surfaces. This enhances overall energy output, especially in regions with high albedo.
- Building-Integrated Photovoltaics (BIPV): This approach integrates solar panels into building materials, such as windows and roofs. BIPV allows for aesthetically pleasing installations that do not compromise the building design.
- Enhanced Energy Storage Solutions: As reliance on solar power increases, effective energy storage becomes essential. Advancements in battery technology, including lithium-sulfur and solid-state batteries, will improve energy retention, addressing intermittency issues associated with solar power.
- Artificial Intelligence and Smart Grids: The adoption of AI in monitoring and managing solar energy systems optimizes performance and enhances grid resilience. Smart grids will allow seamless integration of solar energy, enabling real-time data analysis for improved energy distribution.
- Solar Tracking Devices: Technologies that allow solar panels to follow the sun's path throughout the day can substantially increase energy yield. This dynamic adjustment to the sun's position permits optimal alignment, maximizing energy absorption.
Roles in Global Energy Transition
The transition to solar energy is a vital aspect of moving towards a more sustainable global energy framework. Solar power contributes to energy diversification, enhancing the resilience of energy systems. It allows developing nations to jump-start their electricity generation while reducing carbon footprints. The role of solar energy extends beyond production; it supports job creation in manufacturing, installation, and maintenance of solar technologies.
Some key aspects of solar power’s role include:
- Decentralization of Energy: Solar energy allows local energy production, which diminishes transmission losses and vulnerabilities associated with centralized power sources.
- Global Collaboration: Encouraging shared knowledge across borders leads to better platform improvements. International partnerships can accelerate solar projects, ultimately expanding access to renewable energy.
- Policy and Investment Incentives: Government policies that favor solar energy development can stimulate investment. Financial support through subsidies or tax incentives will enhance accessibility and spur growth.
- Adaptability to Local Needs: Solar energy systems can be tailored to different geographical contexts. In regions with high solar potential, they can be deployed efficiently.
"By investing in solar technology, communities can move toward energy independence while simultaneously addressing climate change."
The End
Summary of Key Points
- Understanding of Photovoltaics: The article outlined how photovoltaic technology harnesses sunlight to generate electricity. Different types of solar cells, such as monocrystalline and polycrystalline, have distinct efficiencies and costs, thus influencing their application.
- Role of Inverters: Inverters convert the direct current (DC) produced by solar panels into alternating current (AC), which is essential for powering homes and businesses. This section highlighted their fundamental importance in a functional solar power system.
- Energy Storage Solutions: The ability to store solar energy is critical, especially for users in less sunny areas or for night-time use. Thus, understanding options like lithium-ion batteries can guide informed choices for homeowners and businesses.
- Efficiency Factors: The examination of the elements impacting solar efficiency, such as angle, orientation, and technology used, provided insights into maximizing energy output.
- Challenges in Adoption: Addressing intermittency and environmental concerns related to manufacturing sheds light on the hurdles that need overcoming to facilitate broader adoption of solar energy.
Final Thoughts on Solar Energy
Solar energy stands as one of the most promising solutions in the quest for sustainable energy. As technology continues to advance, more efficient methods for harnessing solar power emerge. Innovations in materials and manufacturing processes hold the potential to reduce costs and increase the accessibility of solar energy systems.
The future of solar energy is bright, yet it is intertwined with the collective effort to address the challenges discussed. By fostering a deeper understanding of solar technologies and their implications, stakeholders, from students to professionals, can contribute to a more sustainable energy future. This journey towards cleaner energy is not just beneficial; it is necessary for a sustainable world.