Understanding Transcription in Protein Synthesis
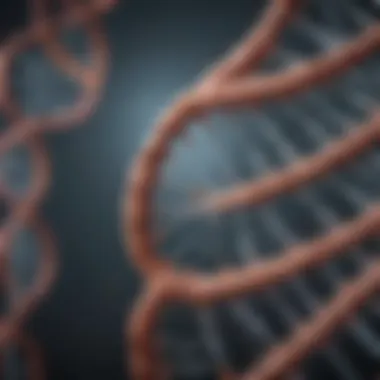
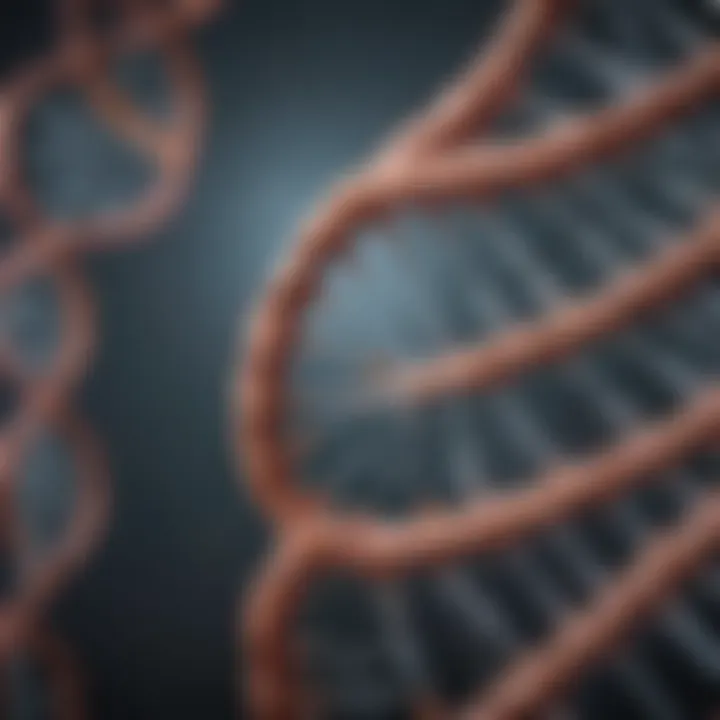
Intro
Transcription is crucial in the central dogma of molecular biology, which details the flow of genetic information within a biological system. It serves as the first step in protein synthesis, where genetic instructions encoded in DNA are converted into messenger RNA (mRNA). This process underpins not only gene expression but also influences cellular functionality and the overall organism's phenotype. Here, we explore the intricate details of transcription, emphasizing its mechanisms, components, and regulatory aspects.
Research Highlights
Key Findings
Transcription involves numerous molecular players, mainly DNA, RNA polymerase, and ribosomes. Recent findings indicate that understanding the interactions among these molecules can elucidate the production of proteins more effectively. Key insights include:
- The initiation stage, where transcription factors facilitate RNA polymerase binding to the promoter region of a gene.
- The elongation phase, where RNA polymerase synthesizes the RNA strand by adding nucleotides complimentary to the DNA template.
- Termination, where RNA polymerase disengages from DNA upon reaching specific terminator sequences.
This highlighted the complexity and precision necessary for successful transcription.
Implications and Applications
The regulation of transcription has profound implications in biotechnology and medicine. For instance, manipulating transcription factors can potentially enable tailored therapeutic strategies for diseases such as cancer. Furthermore, advancements in synthetic biology frequently utilize transcriptional regulation to engineer organisms capable of producing desired proteins.
Methodology Overview
Research Design
The studies on transcription often employ molecular biology techniques, such as ChIP-seq (Chromatin Immunoprecipitation sequencing) and RNA-seq (RNA sequencing), to analyze the dynamic interactions between RNA polymerase and DNA. These tools enable researchers to identify the binding sites of RNA polymerase, as well as the different types of RNA produced during transcription.
Experimental Procedures
Typical experimental procedures include:
- Isolating DNA from specific cells or tissues.
- Using PCR (Polymerase Chain Reaction) to amplify DNA segments of interest.
- Employing RNA polymerase to synthesize mRNA from the DNA template.
- Analyzing the resulting mRNA using sequencing techniques to assess transcriptional activity.
These methodologies ensure a thorough understanding of the transcription process and its regulatory mechanisms.
Epilogue
In summary, transcription plays a fundamental role in protein synthesis, acting as the critical bridge between DNA and functional proteins. Understanding transcription not only provides insights into gene expression but also underlines its significance in cellular processes and potential applications in therapeutic realms. The meticulous mechanisms, regulatory pathways, and the continuous advancement in research tools further accentuate the importance of this biological phenomenon.
Prologue to Protein Synthesis
Understanding protein synthesis is crucial for grasping the foundation of biological processes. This intricate procedure is vital for the growth, maintenance, and regulation of cells within any organism. Detoxifying enzymes, regulatory proteins, hormones, and structural components of cells all emerge from this captivating synthesis. Every organism is essentially a product of its proteins, making the study of these processes imperative.
Definition and Importance
Protein synthesis refers to the biological process in which cells build proteins. This process involves several steps, primarily transcription and translation, which convert genetic information from DNA into functional proteins. The importance of protein synthesis cannot be overstated. Proteins serve as the building blocks of life, participating in virtually every cellular activity. For instance, enzymes, antibodies, and transport proteins all perform specific cellular functions that are critical for survival. By studying protein synthesis, researchers can address various biological questions ranging from disease mechanisms to cellular metabolism. Furthermore, advancements in this field have significant implications in medicine, biotechnology, and genetic engineering.
Overview of Central Dogma
The central dogma of molecular biology outlines the flow of genetic information within a biological system. It encompasses three main processes: replication, transcription, and translation. First, DNA replicates itself during cell division to ensure genetic continuity. Next, transcription involves the synthesis of RNA from a DNA template. Finally, the RNA is translated into a protein, completing the process initiated by the instructions held within the DNA.
Here are key features of the Central Dogma:
- Replication: DNA makes copies of itself.
- Transcription: DNA is transcribed into messenger RNA (mRNA).
- Translation: mRNA is translated to form proteins.
Understanding these processes provides insight into how genetic information is expressed and regulated within cells. It forms a framework for exploring complex biological interactions and understanding diseases caused by mutations or faults in these processes.
Transcription is a critical process in protein synthesis. It serves as the bridge between the genetic information stored in DNA and the functional proteins that perform various roles within cells. Understanding transcription is essential for grasping how genes are expressed, how proteins are synthesized, and eventually how cellular functions are regulated.
In this section, we will explore both the significance of transcription and the nuanced differences between transcription and translation.
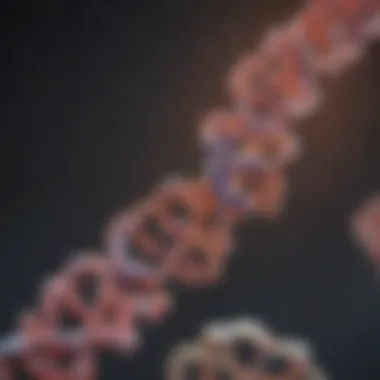
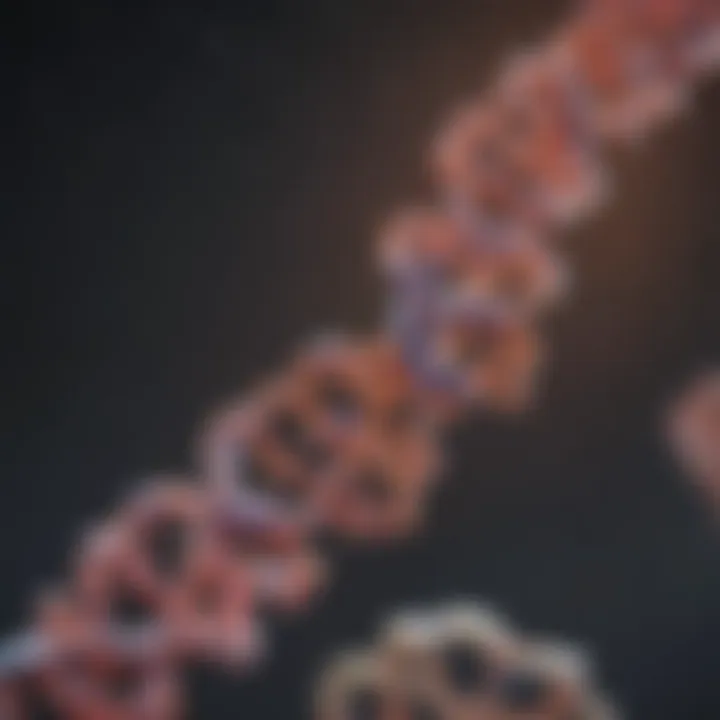
Transcription as a Preliminary Step
Transcription is the first step in the process of gene expression. It involves the synthesis of RNA from a DNA template. Without transcription, the information encoded in the DNA would remain inaccessible for protein synthesis. This step is indispensable for converting genetic information into functional products.
During transcription, RNA polymerase binds to the promoter region of a gene. This marks the start of the transcription process. The RNA polymerase unwinds the DNA strands, allowing the synthesis of a complementary RNA strand. This single strand of RNA carries the genetic information needed for protein production.
The importance of transcription lies in its role as a preliminary step. It essentially dictates which genes will be expressed at any given time, influencing cellular functions and phenotypes. Without effective transcription, the dynamic response of a cell to its environment would be drastically impaired.
Transcription vs. Translation
Understanding the difference between transcription and translation is crucial for comprehending the overall protein synthesis process. While transcription involves creating an RNA strand from DNA, translation is the subsequent step where the RNA sequence is used to synthesize proteins.
- Transcription:
- Translation:
- Location: Nucleus (in eukaryotes)
- Process: Synthesis of RNA from DNA
- Key enzyme: RNA polymerase
- Location: Cytoplasm
- Process: Synthesis of proteins from mRNA
- Key components: Ribosomes, tRNA
Transcription ensures that all necessary information is accurately transcribed into RNA, which then serves as a template for translation. The efficiency and accuracy of transcription have a direct impact on the final protein product.
"Transcription and translation are two fundamental processes in molecular biology that work seamlessly to ensure the continuity of life at the cellular level."
Molecular Players in Transcription
Transcription is a complex process that relies on several key molecular players, each with defined roles. Understanding these components is crucial for comprehending how genetic information is converted into functional proteins. This section delves into the primary players involved in transcription: DNA, RNA polymerase, promoters, and enhancers. Each of these elements holds particular significance in the transcription process, affecting both its efficiency and accuracy.
DNA: The Genetic Blueprint
DNA serves as the template for transcription and contains the information necessary for protein synthesis. It is structured in a double helix, comprising nucleotide sequences arranged in a specific order. Within the DNA, genes are segments that code for proteins. The sequence of these nucleotides directly determines the order of amino acids in a protein, ultimately influencing its structure and function.
DNA must first unwind to expose the genes for transcription to occur. The specificity of this unwinding process is guided by the regions surrounding the genes, which include promoter regions. Proper interactions between various proteins and DNA are vital to ensure that the correct gene is transcribed at the right time. Understanding the sequence and structure of DNA is therefore fundamental for predicting the transcription efficiency and overall gene expression.
RNA Polymerase: The Catalyst
RNA polymerase is the enzyme responsible for synthesizing RNA from the DNA template. It binds to the promoter region, marking the beginning of transcription. This enzyme unwinds the DNA strands and adds RNA nucleotides complementary to the DNA template. The accuracy of RNA polymerase is crucial; errors can lead to malfunctioning proteins and potentially harmful consequences for the organism.
There are different types of RNA polymerases, such as RNA polymerase I, II, and III in eukaryotes. Each has distinct roles in synthesizing various types of RNA. RNA polymerase II, for instance, is primarily involved in transcribing mRNA, which eventually translates into proteins. This enzyme's function and fidelity play a significant role in gene expression regulation.
Promoters and Enhancers
Promoters and enhancers are critical regulatory elements that influence the transcription activity of genes. A promoter is a specific DNA sequence located at the start of a gene, providing a binding site for RNA polymerase and transcription factors. Conversely, enhancers are located away from the gene they regulate and can enhance transcription by facilitating the binding of RNA polymerase to the promoter.
The interaction between these elements is complex. While a promoter ensures the correct initiation of transcription, enhancers can significantly increase the likelihood that a gene will be expressed. The presence and strength of these regulatory sequences determine how effectively a gene is transcribed, affecting the levels of mRNA produced. This layered interaction between promoters and enhancers highlights the intricate control over gene expression within cellular environments.
Understanding the molecular players in transcription provides insight into the regulatory networks that govern gene expression.
In summary, the molecular players in transcription are essential for the accurate expression of genes. With DNA as the template, RNA polymerase acting as the catalyst, and promoters and enhancers modulating the process, a complex yet precise transcription mechanism is established.
Stages of Transcription
Transcription is a critical phase in protein synthesis, representing the initial step of gene expression. Understanding the stages of transcription is essential since each stage plays a significant role. It ensures that the genetic information stored in DNA is translated into a form that can be utilized by the cell to produce proteins. This process involves various components, each contributing to the accuracy and efficiency of transcription.
Initiation of Transcription
The initiation of transcription marks the beginning of the process where RNA polymerase binds to DNA at a specific region known as the promoter. This step is crucial, as it determines where transcription will start. The promoter region contains specific sequences that are recognized by transcription factors, signaling RNA polymerase where to attach.
Once the promoter is recognized, the DNA strands unwind, exposing the template strand for RNA synthesis. It is important to note that only one strand of DNA serves as the template. This phase sets the foundation for the entire transcription process and is tightly regulated to prevent errors. Any mistake in this step can lead to faulty RNA production, thus impacting protein synthesis.
Elongation Phase
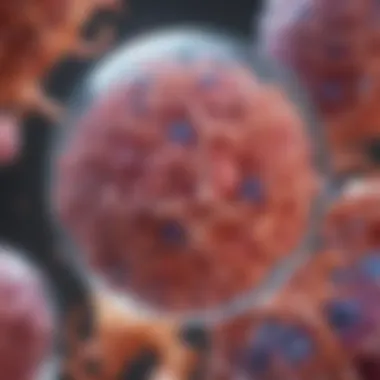
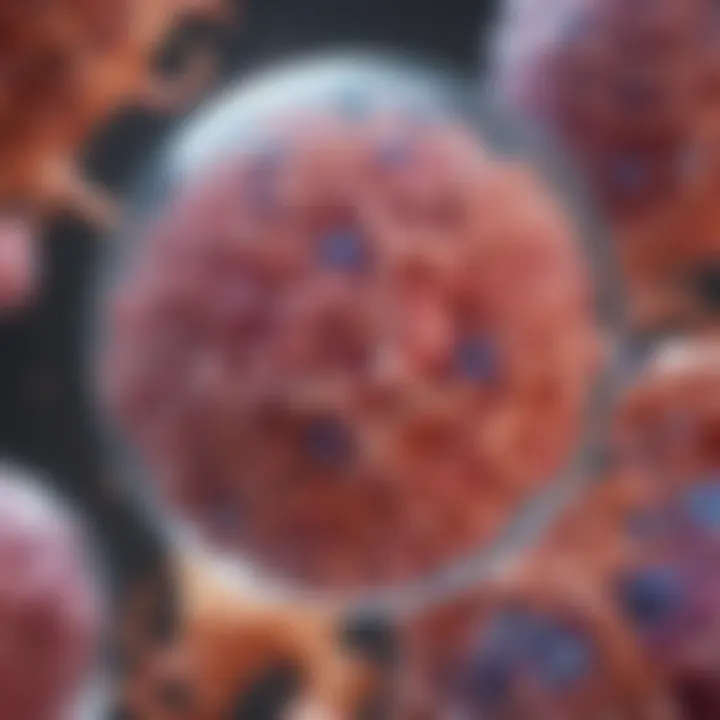
Following initiation, the elongation phase begins. Here, RNA polymerase moves along the DNA template strand, synthesizing RNA by adding ribonucleotides complementary to the DNA sequence. Nucleotide triphosphates (ATP, GTP, CTP, UTP) provide energy and serve as building blocks for RNA.
During this phase, the RNA strand elongates, and the newly formed RNA molecule detaches from the DNA to allow the DNA helix to reform. This process is essential to ensure accurate transcription. Protective mechanisms are in place to minimize mistakes, enhancing the fidelity of RNA synthesis. Each nucleotide added is a specific response to the complementary DNA bases, which ensures that the genetic code is transcribed correctly.
Termination of Transcription
Termination of transcription occurs when RNA polymerase reaches a termination sequence in the DNA. At this point, several factors lead to the release of the newly synthesized RNA strand. The end of transcription is essential for several reasons: it ensures proper completion of RNA synthesis, prepares the RNA for subsequent processing, and allows the transcription machinery to be recycled for future transcription events.
The RNA transcript may also undergo several modifications post-termination, which helps in its stabilization and functionality. Overall, the termination phase concludes the transcription process, allowing the newly formed RNA to fulfill its role in protein synthesis.
"Transcription is the gateway to gene expression, where information from DNA is first converted into RNA. Understanding this process illuminates the complexity of cellular function."
Post-Transcriptional Modifications
Post-transcriptional modifications play a crucial role in the way genetic information is expressed in cells. After transcription from DNA to RNA, the initial RNA transcript, often referred to as pre-mRNA, requires several modifications before it can function effectively in protein synthesis. These modifications serve multiple purposes, including enhancing the stability of RNA, facilitating its transport from the nucleus to the cytoplasm, and regulating the translation process.
Understanding post-transcriptional modifications is essential, as these processes can significantly impact gene expression and cellular function. Misregulation or errors in these processes can lead to various diseases, including cancer and genetic disorders.
Capping and Polyadenylation
Capping and polyadenylation are two significant steps in the post-transcriptional modification of eukaryotic pre-mRNA.
Capping occurs at the 5' end of the mRNA molecule. This process involves the addition of a 7-methylguanylate cap, which protects the RNA from degradation by exonucleases. This cap also plays a role in the initiation of translation by helping ribosomes recognize the mRNA.
Polyadenylation refers to the addition of a poly(A) tail at the 3' end of the RNA. This tail consists of a long chain of adenine nucleotides. Polyadenylation enhances the stability of the mRNA and is important for the export of the mRNA from the nucleus. Moreover, it influences the efficiency of translation and the lifespan of the mRNA molecule in the cytoplasm.
Both processes ensure that the mRNA is properly processed and ready for translation, which is vital for protein synthesis.
Splicing Mechanisms
Splicing is another critical post-transcriptional modification. The initial pre-mRNA contains non-coding regions known as introns and coding regions called exons. During splicing, introns are removed, and exons are joined together to form the mature mRNA. This process is predominantly carried out by a complex known as the spliceosome, which consists of small nuclear RNAs and proteins.
The splicing mechanism is essential for producing a functional mRNA molecule. Mis-splicing can result in the inclusion of introns in the final mRNA or the exclusion of exons, leading to potentially nonfunctional or harmful proteins. Additionally, alternative splicing can occur, allowing a single gene to code for multiple proteins. This process increases the diversity of the proteome, which enhances the complexity of cellular functions.
In summary, post-transcriptional modifications such as capping, polyadenylation, and splicing are vital for the proper expression of genes. They ensure the iintegrity, stability, and functionality of the mRNA, directly influencing protein synthesis.
"Properly processed RNA is essential for translating genetic information into functional proteins, impacting cellular health and organismal development."
Understanding these modifications offers insights into the regulation of gene expression and the potential implications for medical research and biotechnology.
Regulatory Mechanisms in Transcription
Transcription is not just about copying information from DNA to RNA; it is also a highly regulated process. The regulatory mechanisms in transcription play a critical role in ensuring that proteins are produced in the right amounts and at the right times. This regulation is essential in maintaining cellular functions and responding to environmental changes.
These mechanisms include various elements such as transcription factors and epigenetic modifications. The benefits of a well-regulated transcription process are significant. It allows organisms to adapt to different conditions, control cell differentiation, and manage developmental processes. Therefore, understanding these regulatory components is vital for comprehending gene expression and its implications in health and disease.
Transcription Factors
Transcription factors are proteins that bind to specific DNA sequences to regulate the transcription of genes. They can act as activators, promoting the initiation of RNA synthesis, or as repressors, hindering it. Transcription factors recognize specific sequences, often found in promoter regions. Here are key points about transcription factors:
- Specificity: Each transcription factor can interact with specific genes, controlling their expression uniquely.
- Cooperativity: They can work together, enhancing or repressing the transcription process in a coordinated manner.
- Signal Response: Some transcription factors respond to external signals, facilitating adjustments in gene expression based on environmental conditions.
A well-known example is the tumor suppressor p53. This protein binds to specific DNA regions to regulate genes involved in the cell cycle and apoptosis, showcasing how transcription factors can control essential biological processes.
Epigenetic Modifications
Epigenetic modifications refer to changes in gene expression that do not alter the DNA sequence. These changes significantly affect transcriptional activity and can be stable across cell divisions. Key types of epigenetic modifications include DNA methylation and histone modification.
Here is how these modifications impact transcription:
- DNA Methylation: The addition of a methyl group to DNA can repress gene expression by preventing transcription factors from accessing the DNA.
- Histone Modification: Histones are proteins around which DNA wraps. Their chemical modification can influence how tightly or loosely DNA is packaged, impacting accessibility for transcription.
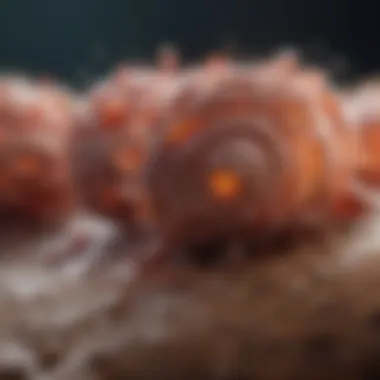
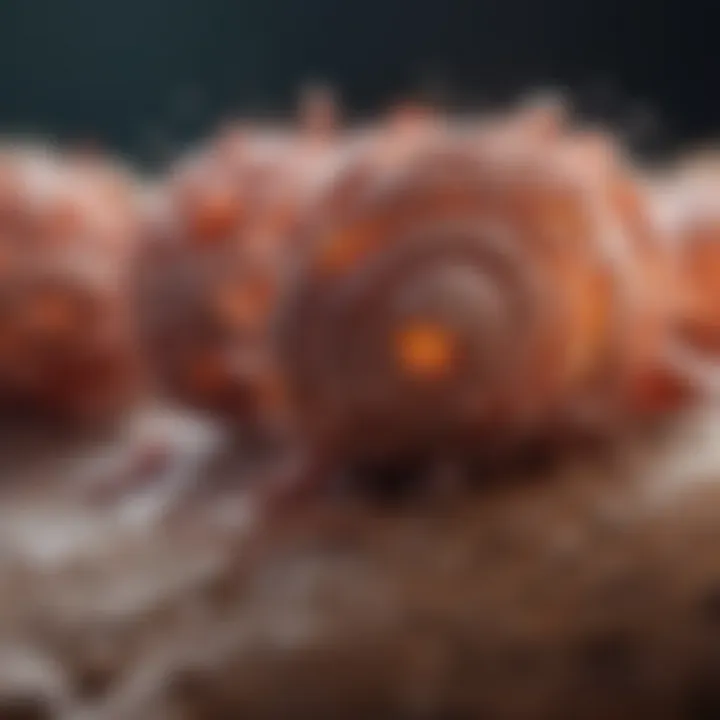
The importance of epigenetic modifications lies in their ability to provide a layer of control over gene expression. They also allow for adaptation to long-term environmental changes and play a role in the inheritance of traits without altering the underlying genetic code.
Epigenetic changes can be influenced by environmental factors such as diet, stress, and toxins, highlighting the interaction between external conditions and gene regulation.
Transcription in Eukaryotes vs. Prokaryotes
Transcription varies significantly between eukaryotic and prokaryotic organisms, shaping the overall efficiency, control, and complexity of protein synthesis. This difference not only reflects the fundamental distinctions in their cellular architecture but also reveals the intricate mechanisms that sustain their life processes. Understanding these variations provides insights into evolutionary adaptations and the functional significance of transcription in different biological systems.
Differences in Mechanisms
Eukaryotes and prokaryotes employ distinct mechanisms during transcription. In prokaryotes, the process typically occurs in the cytoplasm. RNA polymerase binds directly to the promoter regions of DNA, facilitating simultaneous transcription and translation, as there is no nuclear membrane to impede these processes.
Eukaryotic cells, on the other hand, compartmentalize transcription within the nucleus. The process involves multiple steps adapted for complexity:
- Initiation – RNA polymerase II, the main enzyme for mRNA synthesis, requires several transcription factors to identify and bind to the promoter region. This complex assembling is crucial for accurate transcription.
- Capping and splicing – After RNA synthesis begins, the pre-mRNA undergoes capping and splicing, which is not observed in prokaryotic systems.
- Nuclear Export – Mature mRNA is then exported from the nucleus to the cytoplasm, where translation occurs. This multistep transcriptional process contributes to the cell's ability to regulate gene expression finely.
These mechanistic differences highlight how eukaryotes achieve greater control over gene expression. The layered regulatory aspects allow eukaryotic cells to respond to various signals and environmental changes more adeptly than prokaryotes.
Complexity of Regulation
Eukaryotic transcription is characterized by a higher degree of regulatory complexity compared to prokaryotic transcription. Eukaryotes employ diverse mechanisms for the regulation of gene expression, including:
- Transcription Factors: These proteins bind to specific DNA sequences near genes, influencing the transcription process by promoting or inhibiting RNA polymerase activity.
- Epigenetic Modifications: Chemical alterations to DNA and histone proteins influence chromatin structure, affecting accessibility to transcriptional machinery. Examples include DNA methylation and histone acetylation, which can upregulate or downregulate the transcription of specific genes.
- Enhancers and Silencers: Unlike prokaryotes, eukaryotic genes can have regulatory elements located far from the promoter. Enhancers boost transcription whereas silencers inhibit it, creating a network of regulatory controls that fine-tune gene expression in response to developmental cues and environmental stimuli.
In contrast, prokaryotic transcription regulation primarily hinges on the availability of RNA polymerase and the presence of transcription factors called sigma factors, which bind to specific promoters. Although prokaryotes can respond to environmental changes rapidly, their regulatory mechanisms remain more linear compared to the multifaceted strategies utilized by eukaryotes.
Eukaryotic transcription reflects the evolutionary pressure to regulate gene expression across diverse cellular contexts, allowing for increased adaptability and complexity.
Applications and Implications of Transcription Research
Transcription research delves deep into the initial phase of protein synthesis, offering insights into fundamental biological processes. This section highlights its applications in various fields, focusing on its significance in understanding diseases and advancing biotechnological innovations.
Key elements and benefits of transcription research:
- Enhances understanding of gene expression regulation.
- Aids in the development of targeted therapies.
- Supports advancements in synthetic biology and genetic engineering.
Research in transcription is not merely academic; it holds real-world implications that can shape medical interventions and biotechnological applications. The complexities of transcription provide a lens through which scientists can study diseases and devise novel solutions.
Biomedical Research and Disease
Biomedical research often relies on our comprehension of transcription mechanisms. Abnormal transcriptional activity is linked with various diseases, including cancer and genetic disorders. By studying how transcription factors and regulatory elements function, researchers can identify potential therapeutic targets.
In cancer, for example, mutations in genes that encode transcription factors can lead to uncontrolled cell growth. Understanding the transcription process allows for the development of drugs that can intervene at specific points, potentially correcting these issues. A notable focus in this area is personalized medicine, where therapies are tailored based on the transcription profile of individual tumors.
This approach underscores the profound impact of transcription research on leading-edge medical practices.
Biotechnology and Genetic Engineering
Transcription research also bears significant relevance in biotechnology and genetic engineering. By manipulating transcription regulators, scientists can enhance the expression of beneficial genes or suppress harmful ones. This holds particular promise in agricultural biotechnology, where crops can be engineered to express traits like drought resistance or pest resistance.
In synthetic biology, researchers are constructing new biological pathways and systems. An understanding of the transcription process enables the design of gene circuits. These circuits can be programmed to respond to environmental changes or to perform specific functions, such as producing pharmaceuticals on demand.
Epilogue
Summary of Key Points
- Transcription serves as the initial stage in protein synthesis, converting DNA sequences into RNA.
- This process involves key molecular players, notably DNA, RNA polymerase, and a range of regulatory elements.
- Stages of transcription including initiation, elongation, and termination ensure accurate RNA production.
- Post-transcriptional modifications enhance the functionality of RNA molecules, preparing them for translation into proteins.
- Regulatory mechanisms, such as transcription factors and epigenetic modifications, play a significant role in controlling gene expression.
- Differences in transcription processes between eukaryotes and prokaryotes highlight the complexity of gene regulation across life forms.
- Applications of transcription research have far-reaching implications in biomedical fields and biotechnology, contributing to our understanding of diseases and the development of novel therapies.
Future Directions in Transcription Research
The future of transcription research appears promising as advancements continue to unfold in genetics and molecular biology. Ongoing studies aim to uncover the intricate dynamics of transcriptional regulation, particularly the role of non-coding RNAs and their impact on gene expression. Additionally, the development of CRISPR-Cas9 and other gene-editing tools provides exciting opportunities to manipulate transcription in specific contexts, potentially leading to breakthroughs in therapeutic applications.
There is also a growing interest in understanding how environmental factors influence transcriptional regulation and gene expression patterns. The integration of transcriptomics with proteomics and metabolomics will further enrich our knowledge and offer a holistic view of cellular functions.
As research progresses, further exploration into the complex interplay between transcription and diseases, such as cancer and genetic disorders, will yield valuable insights. Overall, the continued investigation of transcription promises to enhance our understanding of biological systems and lead to innovative solutions in medicine and biotechnology.