Types of RNA Sequencing: A Comprehensive Guide
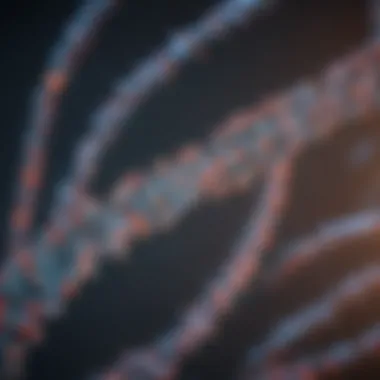
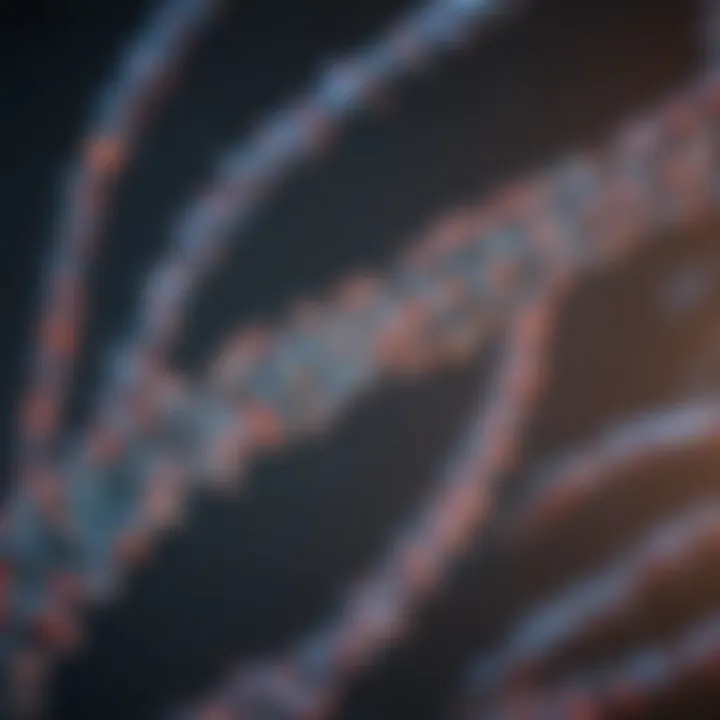
Intro
RNA sequencing has revolutionized the field of molecular biology, providing an unprecedented lens through which to view the complex landscape of gene expression. The ability to uncover the intricacies of the transcriptome allows researchers not only to understand basic biological processes better but also to explore pathological states, like cancers and genetic disorders. In this environment of rapid technological advancement, various RNA sequencing methods have emerged, each boasting unique methodologies and applications that cater to diverse research questions.
Understanding these techniques is paramount for anyone involved in biological research—be it students, educators, or professionals. As we delve into the different types of RNA sequencing, we aim to highlight their principles, strengths, weaknesses, and specific use cases. By grasping the nuances of each method, readers can better appreciate their impact on research outcomes and medical advancements.
Research Highlights
Key Findings
The most notable outcomes of RNA sequencing can be summarized as follows:
- Comprehensive Transcript Profiling: RNA sequencing enables a holistic view of RNA populations, going beyond mere gene expression levels to include alternative splicing, isoform variations, and non-coding RNAs.
- Enhanced Sensitivity: Compared to traditional methods like microarrays, RNA-Seq offers greater sensitivity in detecting low-abundance transcripts, which can be crucial for understanding intricate biological mechanisms.
- Single-Cell Resolution: Recent innovations in single-cell RNA sequencing have made it possible to dissect heterogeneous populations of cells, revealing cellular states and functions that often go unnoticed in bulk analyses.
Implications and Applications
The applications of various RNA sequencing techniques are as varied as the approaches themselves:
- Disease Research: Techniques like RNA-Seq can assist in identifying biomarkers for diseases, leading to better diagnostics and therapeutic strategies.
- Developmental Biology: By employing single-cell RNA sequencing, researchers can track gene expression changes during cellular differentiation and developmental stages.
- Subpopulation Analysis: RNA sequencing allows scientists to study specific cell subsets within a larger population, providing insights into immune responses, tumor evolution, and more.
"The advances in RNA sequencing technologies have opened up a new frontier in our understanding of biology, offering a more nuanced perspective on gene regulation and expression."
Methodology Overview
Research Design
A sound research design is critical for effective RNA sequencing studies. Typically, a study will begin with well-defined research questions, guiding the choice of methodology:
- Bulk RNA Sequencing: Utilized for understanding overall tissue gene expression and provides an average profile of the RNA content.
- Single-Cell RNA Sequencing: Lends itself to more targeted questions, examining the gene expression profiles of individual cells.
Experimental Procedures
The experimental procedures can differ significantly based on the type of RNA sequencing employed:
- Sample Preparation: This step varies; bulk sequencing may require homogenization and RNA extraction from tissues, while single-cell approaches require careful isolation of individual cells.
- Library Construction: A critical step that involves converting RNA into complementary DNA (cDNA) and preparing it for sequencing.
- Sequencing and Analysis: Following sequencing, bioinformatics tools and pipelines are necessary to analyze the data, translating raw reads into meaningful biological insights.
By understanding these methodologies and their implications, we set the stage to further explore the specific types of RNA sequencing techniques available, providing valuable context for future discussions.
Preface to RNA Sequencing
In the field of molecular biology, RNA sequencing has become a crucial technique, much like having a reliable compass in an uncharted forest. This method allows scientists to probe deep into the world of genes, uncovering the vast array of transcripts produced by cells. The significance of RNA sequencing cannot be overstated; it gives researchers detailed insights into gene expression and regulation at both broad and granulated levels. As we embark on this exploration, we’ll highlight several core elements of RNA sequencing that highlight its value in contemporary research.
First, let’s consider what RNA sequencing does. By providing a snapshot of the transcriptome, it enables the assessment of which genes are actively being expressed in particular conditions or developmental stages. With RNA sequencing, it’s easier to observe how various external factors, such as environmental stressors or treatments, influence gene expression. This dynamic capability is vital in fields like cancer research, where understanding gene regulation and expression can lead to breakthroughs in treatment and diagnosis.
Furthermore, RNA sequencing techniques are adaptable. There are several methodologies, each suited to different research needs. Whether it’s whole transcriptome sequencing for a broad analysis or targeted RNA sequencing focusing on specific genes, these methods arm scientists with a versatile toolkit to tailor their investigations effectively.
Considerations abound when it comes to choosing the right RNA sequencing technique. Factors such as the specific research question, the type of sample, and budget constraints can dictate the approach. Despite the challenges, the potential benefits make RNA sequencing an indispensable element in the biological sciences.
"RNA sequencing offers a profound window into gene expression, one that can lead to significant scientific advancements."
Fundamental Techniques in RNA Sequencing
RNA sequencing has rapidly changed the landscape of molecular biology. As the world moves towards personalized medicine and understanding the intricacies of gene expression, the fundamental techniques in RNA sequencing have become indispensable. These techniques serve as the backbone for various methodologies used in this field, enabling researchers to extract insights from the transcriptome efficiently and accurately.
Overview of Standard RNA-Seq
Standard RNA sequencing, often simply referred to as RNA-Seq, has established itself as a gold standard in transcriptome analysis. At its core, the technique involves converting RNA into complementary DNA (cDNA) through reverse transcription. This cDNA is then amplified and sequenced, yielding a wealth of data about gene expression levels within a sample. One of the standout benefits of RNA-Seq lies in its ability to detect not only known transcripts but also novel ones, which can be a game changer in research.
To break it down:
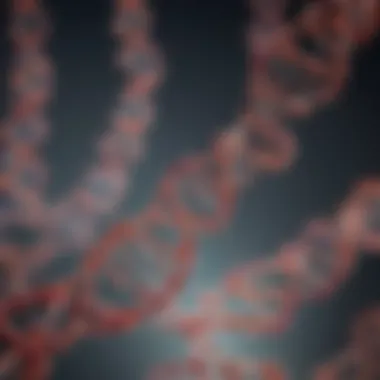
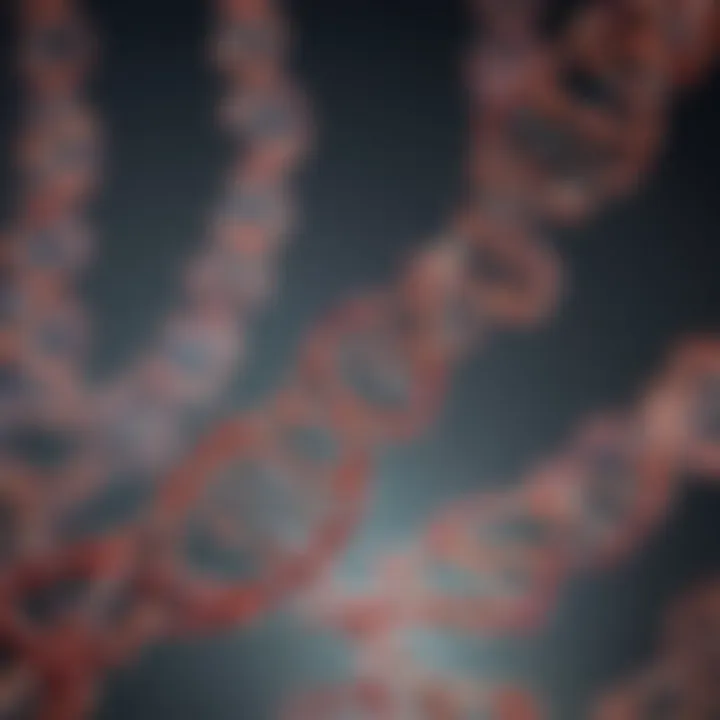
- The initial step usually involves isolating RNA from the cells or tissues of interest.
- Next, through reverse transcription, this RNA is converted to cDNA.
- Following this, a series of amplification reactions prepare thousands to millions of DNA fragments, which are then sequenced.
This whole process allows researchers to determine the abundance of transcripts and discover variants, thereby paving the way for deeper biological insights. What's important to note is that standard RNA-Seq is not without its challenges, including the need for high-quality RNA, suitable library construction, and the complexities of data interpretation.
Quantitative RNA Sequencing
Quantitative RNA sequencing takes the insights obtained from standard RNA-Seq a step further by focusing on measuring the levels of gene expression quantitatively. This technique is particularly beneficial for studies aiming to quantify transcript abundance more accurately. The methodology often employs techniques such as unique molecular identifiers (UMIs) or digital gene expression counting to improve the precision of data.
In a nutshell, here’s how it works:
- Sample Collection: RNA is extracted from the cells or tissues.
- Library Preparation: Each transcript is tagged with a unique identifier to monitor its abundance during sequencing.
- Sequencing: The library is sequenced to provide a detailed view of gene expression.
Notably, this approach helps in reducing biases rooted in read depth, leading to more reliable data. Quantitative RNA sequencing is especially crucial in comparative studies where the specific level of expression must be quantified across different conditions or groups, like tumor versus normal tissue.
"In RNA sequencing, clarity often lies in the details—accurate quantification allows for a clearer understanding of transient biological phenomena."
In essence, both standard RNA-Seq and quantitative RNA sequencing are fundamental techniques that set the stage for future discoveries in various biological contexts. They serve as critical tools in the scientist's toolkit, aiding in the exploration of life's complex symphony hidden within the transcriptome.
Types of RNA Sequencing Techniques
RNA sequencing has opened the floodgates to a better understanding of cellular functions and gene expression. Each RNA sequencing technique offers unique advantages, serving specialized research objectives. By diving into diverse types of RNA sequencing, researchers can tackle specific questions in genetics, development, and even disease pathology. The choice of technique often depends on factors such as the sample type, complexity of the transcriptome, and the desired resolution.
Whole Transcriptome Sequencing
Methodology and Protocols
Whole transcriptome sequencing (WTS) captures the entire RNA profile of a cell, enabling researchers to gain a comprehensive view of gene expression. The methodology typically involves extracting RNA, converting it to complementary DNA (cDNA), and applying next-generation sequencing (NGS). The key characteristic of WTS is its ability to quantify all transcripts, including non-coding RNA, which is often overlooked in standard approaches.
A unique feature of WTS is its flexibility; it can analyze RNA samples from various sources, making it highly beneficial for comparing gene expression across different conditions. However, the breadth of data generated can also be overwhelming, requiring robust bioinformatics tools for data analysis.
Applications in Research
WTS plays a pivotal role in various research fields. Its capability to analyze and compare transcriptomes has made it a powerful tool in cancer research, allowing for the identification of novel biomarkers and therapeutic targets. The comprehensive data from WTS aids in understanding the cellular responses during diseases and development. Yet, the extensive data needed for interpretation can pose challenges; researchers must be prepared to manage and analyze large datasets effectively.
Targeted RNA Sequencing
Focus on Specific Gene Regions
Targeted RNA sequencing narrows its focus to specific gene regions of interest, providing a more manageable dataset that emphasizes depth of coverage. This technique allows researchers to explore the expression levels of particular genes associated with diseases, making it a favored choice among those seeking precise data. A significant advantage lies in its efficiency; since it doesn't cover the entire transcriptome, it requires less sequencing time and resources.
However, a drawback is the potential for missing out on broader interactions within the transcriptome, which could be critical for mutual gene involvement in complex traits or diseases.
Use Cases and Advantages
This approach is particularly useful in clinical research where quantifying specific biomarkers can influence diagnostic or treatment decisions. Targeted RNA sequencing is often utilized in oncology, enabling oncologists to monitor specific cancer-related gene alterations over time. Its focused nature leads to cost-effectiveness and heightened sensitivity when studying low-abundance transcripts. Nevertheless, researchers must balance the advantages of depth against the possible oversights of excluding relevant gene expression data.
Single-Cell RNA Sequencing
Technological Advances
Single-cell RNA sequencing (scRNA-seq) represents a significant leap forward, allowing researchers to explore gene expression at the individual cell level. The advancement in droplet-based technologies and microfluidics has made scRNA-seq a groundbreaking technique. Its ability to dissect complex tissues into unique cell profiles has tremendous implications for understanding cell differentiation and function.
A key characteristic of scRNA-seq is its capacity to uncover cellular heterogeneity, offering insights into how different cells in a seemingly homogeneous population may contribute to disease. While scRNA-seq can elucidate intricate cellular processes, the analysis often requires sophisticated computational frameworks, thus posing a hurdle for some labs.
Insights into Cellular Heterogeneity
This method has profoundly influenced developmental biology, providing insights into cellular diversity during processes such as embryogenesis or tumor progression. scRNA-seq highlights the distinct roles of individual cells within tissues, sparking new hypotheses about cellular behavior and interactions. However, while it reveals the ‘who’s who’ at a cellular level, it can sometimes be limited by low RNA yield from single cells, which complicates the analysis of gene expression.
Spatial Transcriptomics
Mapping Gene Expression
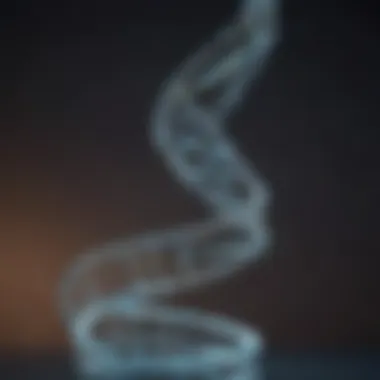
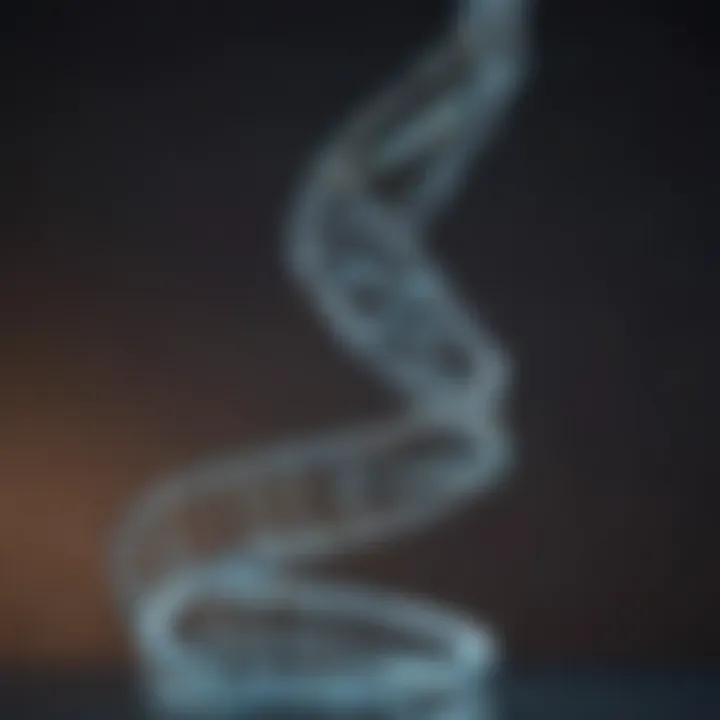
Spatial transcriptomics delivers a way to examine the spatial organization of gene expression in tissue samples, combining histology with transcriptomic analysis. This method involves preparing tissue sections and then mapping gene expression at a cellular resolution, revealing how gene activity correlates with tissue architecture. The capacity for high granularity complemented by spatial context makes this technique especially informative for developmental biology and pathology.
One unique feature of spatial transcriptomics is its ability to illuminate the local cellular microenvironment, which can be crucial for understanding diseases like cancer. However, the high cost and technical complexity may limit its widespread application, especially in settings with limited resources.
Implications for Tissue Studies
The implications of spatial transcriptomics are vast, providing new avenues for exploring how genes regulate local cellular interactions in their native environments. This approach sheds light on tumor microenvironments and immune responses, significantly enhancing our grasp of multicellular interactions. Still, the interpretative side of the data can be quite elaborate, requiring careful consideration when deciphering results.
Double-Strike RNA Sequencing
Double-strike RNA sequencing, while less commonly discussed, showcases an innovative approach to dissecting RNA biology. This method integrates two rounds of sequencing to fine-tune the sensitivity and specificity of gene expression analysis. By employing two strikes on the same sample, researchers can achieve a layered understanding of complex transcriptome dynamics. It's particularly advantageous for studying transient transcripts or those with low expression levels.
As with any emerging technique, discussions about its applications, benefits, and drawbacks continue to evolve among scientists. The balance between the insight gained and the complexity of additional layers of data remains a topic of interest as this field matures.
In summary, exploring the various techniques of RNA sequencing highlights the evolving landscape of molecular biology, emphasizing the intricate nature of transcriptomic research. Each technique from whole transcriptome to single-cell approaches reveals new layers of complexity, allowing for enhanced insight into the fabric of life at a molecular level.
Applications of RNA Sequencing
The realm of RNA sequencing is expansive, making its applications pivotal in various domains of biological research. Understanding the applications of RNA sequencing illuminates not only its functionality but also the transformative impact it has on scientific discovery. By leveraging the specific techniques in RNA sequencing, researchers can delve deep into the molecular intricacies that define organismal biology. This section will explore how RNA sequencing advances cancer research, informs developmental biology, and facilitates biomarker discovery, ultimately providing nuanced insights into living systems.
Cancer Research and Diagnostics
Cancer research stands as one of the most significant areas enhanced by RNA sequencing technologies. The ability to analyze gene expression profiles in tumors contributes to a deeper understanding of cancer biology. For instance, RNA sequencing comprises a powerful method to identify mutations and aberrant gene expression, which can differentiate between tumor types and stages. By doing so, it helps in identifying personalized treatment strategies based on the specific genetic makeup of an individual’s cancer.
A promising example of this application is in the analysis of non-small cell lung cancer. By comparing transcriptomes of patients with different prognoses, researchers can pinpoint specific pathways that might be activated or suppressed in aggressive tumors. This knowledge not only aids in diagnosis but also plays a crucial role in devising targeted therapies, significantly improving patient outcomes.
Moreover, RNA sequencing can also detect minimal residual disease, which is a key factor in preventing relapse. This technique provides insights into the efficacy of treatments, allowing clinicians to adjust therapeutic strategies in real-time.
Understanding Developmental Biology
RNA sequencing also serves as a cornerstone in the study of developmental biology, shedding light on the complex processes that govern organismal development. By analyzing gene expression at different stages of development, researchers can map out regulatory networks that dictate how cells differentiate into various cell types.
For example, scientists may track the expression profiles of embryonic stem cells as they differentiate into defined lineages, highlighting critical genes that govern fate decisions. This is particularly relevant in regenerative medicine, where understanding the cellular reprogramming can lead to advancements in tissue engineering and stem cell therapy.
Analyzing these dynamic transcriptional landscapes allows researchers not just to observe changes but to predict outcomes. When researchers can pinpoint when and how specific genes are expressed throughout development, they open doors to resolving questions about developmental disorders that arise from improper gene regulation.
Biomarker Discovery
Biomarker discovery is another promising application of RNA sequencing, transforming the landscape of diagnostics and personalized medicine. RNA sequencing facilitates the identification of biomarkers that can indicate disease presence, progression, or response to treatment.
Biomarkers derived from RNA sequencing are particularly useful because they reflect the active biological processes occurring within tissues. For instance, in diseases like Alzheimer's, specific RNA signatures can be isolated that correlate with the disease state much earlier than traditional diagnostic methods.
Additionally, biomarkers can be categorized into various classes, such as those indicative of drug response or those that highlight disease stages. Researchers might utilize RNA sequencing data to generate a list of differentially expressed genes in cancers, which can then be cross-referenced with databases to identify potential biomarkers for use in clinical settings.
"The integration of RNA sequencing technologies in biomarker discovery not only champions precise diagnostics but also enhances therapeutic decisions based on an individual’s unique genetic landscape."
Technical Considerations in RNA Sequencing
Understanding the technical aspects of RNA sequencing is crucial for researchers who aim to glean meaningful insights from the data generated. When one considers RNA sequencing, it's not just about getting a pile of data but ensuring that the methods employed yield accurate and reliable results.
Key elements such as sample preparation, quality control, and data processing play a pivotal role in the effectiveness of the sequencing process. For instance, if the RNA sample is degraded or of poor quality, it can lead to unreliable results that can misguide subsequent research. Thus, sound technical practices must be observed at every step.
Importantly, mastering these technicalities not only aids in generating quality results but also enhances the reproducibility of experiments, a cornerstone of scientific integrity.
Sample Preparation and Quality Control
Sample preparation is where it all begins. Obtaining high-quality RNA is the bedrock for successful sequencing experiments. It’s like laying a solid foundation before building a house. Researchers typically utilize specific kits and protocols designed to isolate and preserve RNA integrity. Any mishaps during this preparing stage could end up as costly errors down the road.
Quality control measures often include assessing RNA integrity using tools like the Agilent Bioanalyzer. A well-prepared sample should have a high RNA integrity number (RIN). Samples with lower RIN scores may skew data interpretations, impacting the outcomes of studies on gene expression, alternative splicing, and more.
Data Analysis and Interpretation
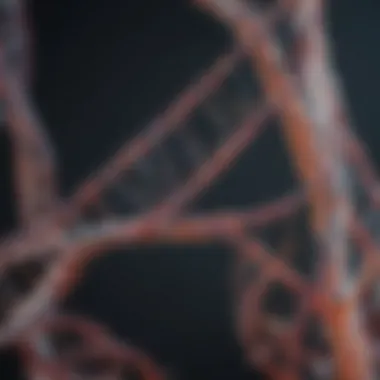
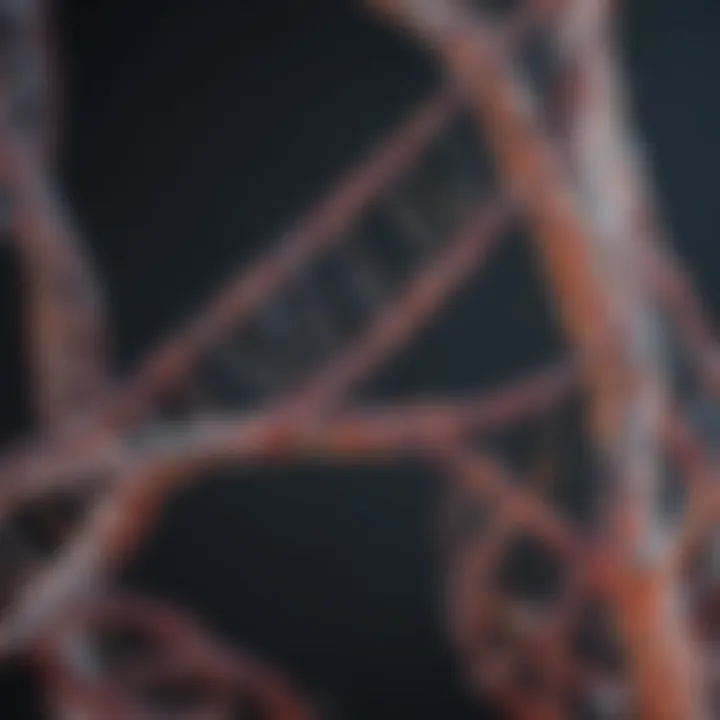
Data analysis is might be among the most demanding and critical phases in RNA sequencing. Once the sequencing is completed, the sheer volume of data produced can be overwhelming. Hence, the quality of bioinformatics tools becomes paramount.
Bioinformatics Tools
Bioinformatics tools serve as the compass in navigating the complex landscape of RNA sequencing data. They range from established software like STAR and HISAT for alignment to edge tools like DESeq2 for differential expression analysis. Each tool boasts unique features tailored to tackle specific challenges in data interpretation.
A notable characteristic of these tools is their ability to process large datasets efficiently. By employing these tools, researchers can quickly analyze vast amounts of background noise generated during sequencing. This efficiency is vital for studies where time-sensitive decisions are paramount. The high adaptability of bioinformatics tools makes them a popular choice in the RNA-seq community.
"The right bioinformatics tool can transform noisy sequences into coherent narratives about genetic expression."
Challenges in Data Processing
Data processing brings its own set of challenges. One must navigate issues related to batch effects, normalization, and potential biases that can arise from the sequencing process itself. For instance, batch effects can distort results, leading to incorrect conclusions if not adequately addressed.
The inherent complexity of the data means that researchers must sometimes juggle multiple questions simultaneously. The focus should not only be on the outcome but also on understanding the underlying discrepancies that might arise due to varying sequencing depths or sample origins. Thus, attention to processing details can make or break a study.
In the grand tapestry of RNA sequencing, the threads of technical considerations weave everything together. By paying heed to proper sample preparation, diligent quality control, and robust data analysis, researchers can ensure their findings contribute meaningfully to the broader scientific dialogue.
Future Directions in RNA Sequencing Research
As the field of molecular biology continues to evolve, the significance of RNA sequencing (RNA-Seq) is becoming clearer than ever. Understanding the future directions in RNA sequencing research is crucial for anyone involved in genomic studies, from students to seasoned professionals. With each passing year, advancements in technology and methodology continuously reshape how we analyze and interpret RNA data.
The focus areas in the upcoming climate of RNA-Seq include enhancing the resolution, accuracy, and speed of sequencing methods. These developments are not merely incremental; they promise to revolutionize how we approach various biological questions.
One of the key benefits is the potential for broader applications. Researchers are keen to explore RNA-Seq in clinical diagnostics and personalized medicine, giving rise to an era where treatments could be specifically tailored based on a patient's transcriptome.
"The future of RNA sequencing hinges on integrating technological advances with biological insights, paving the way for groundbreaking discoveries in medicine and biology."
Innovative Technologies on the Horizon
A wealth of innovative technologies is emerging, poised to transform RNA sequencing landscape. One notable player is the development of nanopore sequencing, which allows for continuous read lengths and real-time analysis. Unlike traditional methods that rely on PCR amplification, nanopore technology can sequence native RNA molecules with high fidelity, minimizing biases.
Another promising approach is the combination of RNA-Seq with CRISPR technology. This integration can pinpoint the roles of specific genes within complex pathways.
Here are a few technologies to keep an eye on:
- Single Molecule, Real-Time (SMRT) Sequencing: Offers the ability to read longer RNA sequences and reduce the risk of data dropout.
- Spatial Transcriptomics: Maps RNA distribution in tissues, bringing about a better understanding of cellular environments.
- Long-Read Sequencing: Enhances the detection of full-length transcripts, which is critical for understanding alternative splicing.
Integrative Approaches in Genomics
The integration of RNA sequencing data with other omics technologies, like proteomics and metabolomics, opens a new world of possibilities.
As scientists embrace a systems biology approach, the aim is to connect different layers of biological information. For instance, linking transcriptomic data with protein expression patterns can provide insights into regulatory mechanisms that govern cellular functions. This holistic view is necessary for comprehending complex diseases, such as cancer, where multiple pathways interact.
Utilizing machine learning algorithms with RNA sequencing data holds great promise. The ability to analyze vast datasets opens up potential for predictive modeling and identifying biomarkers for diagnostics.
In summary, the future directions in RNA sequencing research are rife with exciting developments. By embracing new technologies and integrative methodologies, the scientific community stands to gain not just improved tools, but also a much deeper understanding of the intricate dance of genes, RNA, proteins, and their role in health and diseases.
Closure
As we wrap up this exploration of the diverse types of RNA sequencing, it's clear that these techniques play a pivotal role in advancing our understanding of molecular biology. The evolution of RNA sequencing has brought forth a suite of methods tailored to different research needs, each boasting unique strengths and limitations.
Summation of Key Insights
In reviewing the various RNA sequencing approaches, several key insights stand out:
- Different Techniques for Different Needs: From whole transcriptome sequencing to targeted RNA sequencing, each method serves a distinct purpose. Researchers can choose methodologies that align best with their specific objectives, whether they’re interested in a broad overview of gene expression or a focused study on selected genes.
- Single-Cell Resolution: The advancement of single-cell RNA sequencing has unlocked new avenues for understanding cellular diversity. By examining gene expression at the individual cell level, researchers can glean insights into heterogeneity that were previously obscured in bulk analyses.
- Spatial Considerations: Spatial transcriptomics introduces the ability to map gene expression in tissue contexts, providing a more physiologically relevant view of gene activity. This has profound implications for fields like developmental biology and cancer research.
"One of the significant benefits of RNA sequencing lies in its ability to adapt to evolving research needs, ensuring that scientists remain at the cutting edge of biological inquiry."
- Applications Beyond Research: RNA sequencing is not just for academic research. Its applications in clinical settings, such as cancer diagnostics and biomarker discovery, illustrate its real-world impact.
The Evolving Landscape of RNA Sequencing
The landscape of RNA sequencing continues to evolve at a rapid pace. Each advancement, whether technological or methodological, pushes the boundaries of what is scientifically possible.
- Emerging Technologies: New sequencing technologies promise to further enhance resolution, reduce costs, and increase throughput. Innovations like nanopore sequencing and improving bioinformatic algorithms allow researchers to analyze more complex datasets with ease and accuracy.
- Integration with Other Omics: There is a growing trend toward integrating RNA sequencing data with genomic, proteomic, and metabolomic data, a holistic approach that offers deeper insights into biological processes.
- Broader Accessibility and Collaboration: As these sequencing technologies become more accessible, there is a remarkable increase in collaborative research efforts worldwide. This accelerates the translation of scientific findings from bench to bedside, driving real change in healthcare.