Understanding Alanine Scanning in Protein Engineering
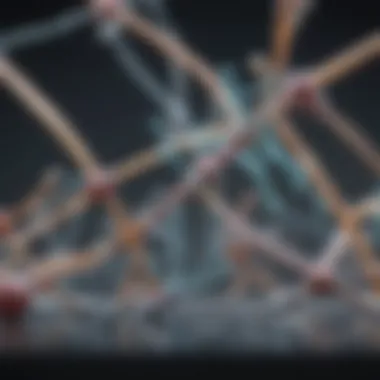
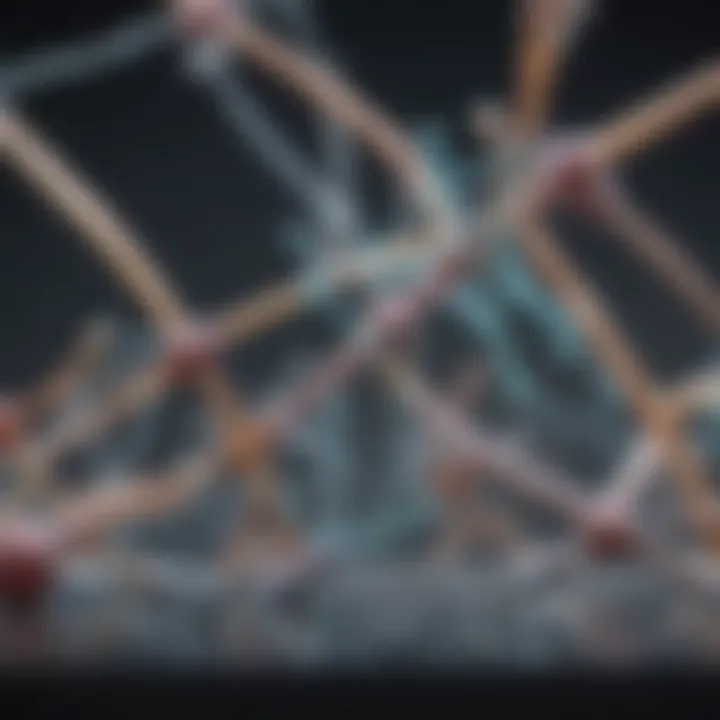
Intro
Alanine scanning has emerged as a significant tool in molecular biology, particularly in the field of protein engineering. Understanding how specific amino acids contribute to the structure and function of proteins can lead to groundbreaking advances in biotechnology. Through systematic substitution of amino acids with alanine, researchers are able to assess their individual roles. This approach simplifies complex protein interactions into manageable investigations.
In this article, we will explore the methodologies involved in alanine scanning, examine its various applications, and highlight the insights gained from this technique. By bridging theoretical knowledge with practical experimentation, we aim to provide a comprehensive understanding of alanine scanning and its utility in protein design.
Research Highlights
Key Findings
Alanine scanning has provided crucial information about protein stability, folding, and interaction sites. Some notable findings include:
- Identification of Critical Residues: Research has shown that nearly 30% of the amino acids in certain proteins are critical for maintaining structural integrity.
- Functional Insights: The technique has helped identify residues that play key roles in enzymatic activity and ligand binding.
- Protein Engineering Applications: By pinpointing essential residues, alanine scanning aids in the rational design of proteins with enhanced functionalities.
Implications and Applications
The implications of these findings are significant. Alanine scanning can:
- Facilitate drug design by targeting specific residues within proteins.
- Lead to the development of more effective vaccines by understanding protein interactions with immune receptors.
- Inform studies of protein-protein interactions, enhancing our grasp of cellular mechanisms.
Through these applications, alanine scanning proves to be an invaluable resource in molecular biology, with potential benefits spanning various disciplines.
Methodology Overview
Research Design
The research design for alanine scanning typically involves two main components: selection of target residues and systematic substitution.
- Target Residue Selection: Researchers identify amino acids within a protein that are hypothesized to play essential roles.
- Systematic Substitution: Each selected residue is replaced with alanine to evaluate its contribution to protein function.
Experimental Procedures
The experimental procedures involve several steps:
- Site-Directed Mutagenesis: This technique is used to create mutations in the DNA encoding the protein, leading to the production of alanine-substituted variants.
- Protein Expression and Purification: The mutated proteins are expressed in host systems like Escherichia coli and subsequently purified.
- Functional Assays: Various assays are conducted to test the functional properties of the mutated proteins compared to the wild-type.
This meticulous methodology allows for thorough evaluations of each amino acid's role, significantly enriching our understanding of protein dynamics.
Foreword to Alanine Scanning
Alanine scanning is a crucial technique in the field of molecular biology. It allows researchers to dissect the contributions of individual amino acids in the context of protein structure and function. Understanding how specific residues affect protein interactions and stability can provide insights into protein design, aiding both basic and applied research. By replacing amino acids with alanine, researchers can investigate whether these residues are essential for maintaining protein activity or stability.
This methodology plays a significant role in protein engineering. The ability to evaluate the impact of alterations on protein performance opens up avenues for designing proteins with improved functionalities. Through alanine scanning, scientists can refine therapeutic targets in drug design and enhance the efficacy of enzymes used in industrial applications.
Alanine scanning not only elucidates fundamental concepts in structural biology but also serves practical applications in biotechnology. Understanding the dynamic roles of amino acids in proteins can lead to the development of innovative biotechnological solutions. As a result, this technique is becoming more integral to modern molecular biology practices.
Definition and Purpose
Alanine scanning involves systematically substituting one or several amino acids in a protein sequence with alanine. The primary purpose of this approach is to pinpoint residues that are critical to the protein's structure and function. By analyzing the changes in activity, stability, and binding interactions due to these substitutions, scientists can discern the roles of different amino acids in the larger protein context. This technique can uncover crucial information about enzyme active sites, receptor ligand interactions, and overall protein folding.
Furthermore, alanine scanning provides qualitative and quantitative data that contributes to our understanding of protein dynamics. It helps create a more nuanced view of how proteins work, ultimately aiding in the development of targeted interventions in health and disease.
Historical Context
The concept of alanine scanning has its roots in the development of site-directed mutagenesis in the late 20th century. Researchers sought methods to understand protein functions at a molecular level, leading to methodologies that allowed for the precise alteration of amino acids in proteins. Over the years, studies utilizing alanine scanning have significantly expanded, showcasing its utility in various areas of research.
Initially, studies using this technique focused on enzyme catalysis. Researchers discovered that specific amino acids played pivotal roles in enzymatic reactions. This sparked further investigation across diverse areas, such as receptor biology and structural biology, where researchers continue to apply alanine scanning to explore interactions and specific functions within proteins.
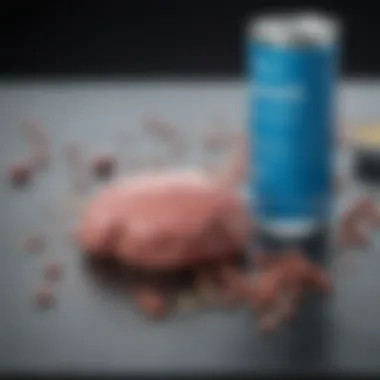
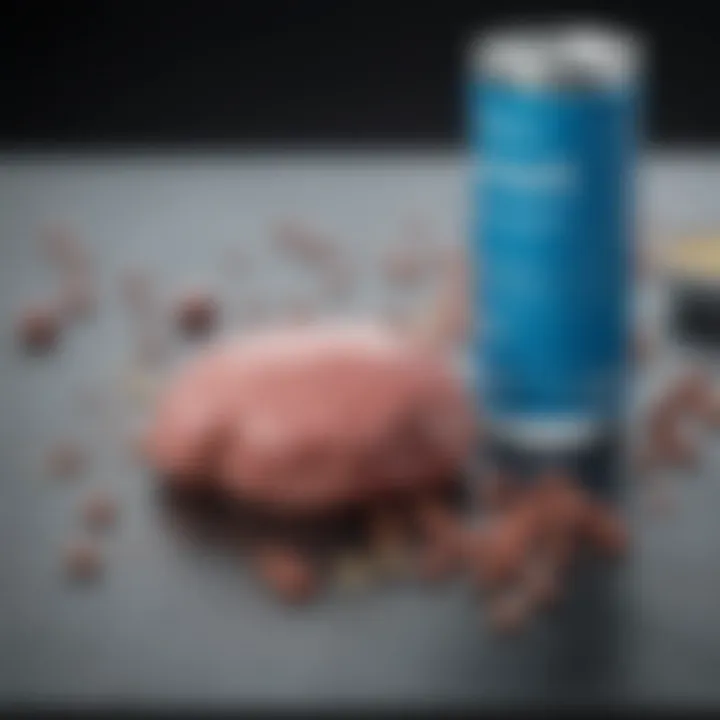
As molecular techniques became more refined, the applications of alanine scanning have grown, solidifying its place as a standard analytical tool in protein engineering. This evolution underscores the importance of alanine scanning in advancing our understanding of protein function and design.
Principles of Alanine Scanning
Understanding the principles behind alanine scanning is crucial for grasping its utility in protein engineering. This technique allows researchers to uncover the intricate relationships between amino acid residues and the overall structure and function of proteins. By systematically replacing amino acids with alanine, scientists can assess the contribution of individual residues to protein stability, enzymatic activity, and ligand binding. This focus on discrete elements helps in designing better proteins and gaining key insights into biological processes.
Mechanism of Action
The mechanism of action in alanine scanning is relatively straightforward yet profound. It involves mutating selected amino acids within a protein to alanine, which is a small, neutral residue. This replacement often leads to a diminished or altered function of the protein, allowing for the inference of the original amino acid's role. The rationale behind substituting with alanine lies in its simple structure, which minimizes steric hindrance and electrostatic interactions. By observing changes in protein behavior or activity, researchers can identify critical residues that contribute to various biochemical pathways.
In a typical approach, a specific protein is chosen, and potential target residues are identified based on previous structural and functional information. Each identified residue is then systematically substituted with alanine. After mutagenesis, the resulting variants are expressed, purified, and analyzed using functional assays. This process helps determine the effect of each mutation. The results lead to a quantitative understanding of the role of specific amino acids in maintaining protein integrity.
Role of Alanine in Protein Structure
Alanine plays a unique role in protein structure due to its simple side chain. Its presence is often associated with localized stability in protein structures. When substituted into complex areas of a protein, alanine can help reveal the importance of hydrophobic interactions and steric effects. In proteins where precise interactions are crucial, alanine serves as a useful tool to evaluate how replacements can impact overall stability or functionality.
Alanine residues often can be found in structural motifs like alpha helices and beta sheets. This positioning further emphasizes their functional significance. The impact of alanine scanning is profound, as the mutation of a critical alanine can have cascading effects on overall protein behavior. Understanding where alanine fits thus informs researchers about the spatial and functional dynamics of larger protein interactions.
"Alanine scanning is a cornerstone in the toolkit of molecular biology, offering insights that pave the way for novel protein designs."
Methodology of Alanine Scanning
The methodology of alanine scanning is integral to understanding the functional roles of amino acids in protein structures. This area of research involves strategic planning and techniques that enable scientists to isolate specific residues for mutation analysis. By swapping various amino acids in proteins to alanine, researchers can discern the importance of these residues in biochemical processes and structural stability. This systematic approach sheds light on intricate protein interactions and helps refine our knowledge about molecular architecture.
Designing Alanine Scanning Mutants
Designing alanine scanning mutants requires careful consideration of the target protein's structure and function. Researchers typically start with structures derived from X-ray crystallography or NMR spectroscopy. Software tools may be employed to predict the impact of mutations on protein function. Key factors in designing these mutants include the conservation of the residue, its role in binding interactions, and overall structural integrity. The success of alanine scanning depends heavily on how effectively these factors are analyzed.
The role of alanine is central here. Because alanine is a small, hydrophobic amino acid, it often does not disrupt the protein's overall structure when substituted for larger or charged residues. As a result, this process enables clearer insights into the role of the native amino acids. The goal is to generate a library of mutant proteins that can be characterized further in functional assays.
Experimental Techniques
Site-Directed Mutagenesis
Site-directed mutagenesis allows for the precise alteration of specific amino acids within a protein. This technique is vital in alanine scanning as it provides a direct method for introducing alanine substitutions. The key characteristic of this method is its high specificity, enabling targeted changes without affecting adjacent residues. Researchers typically use oligonucleotide primers designed to carry the desired mutation during the PCR amplification process. This precision makes site-directed mutagenesis a popular choice in alanine scanning studies.
One unique feature of site-directed mutagenesis is that it can be combined with various other techniques to validate the mutations. However, it can be labor-intensive and may require extensive testing to ensure the desired mutations are achieved.
Expression and Purification
Once the alanine mutants are created, they must be expressed and purified for study. The expression systems such as Escherichia coli or Yeast are often used, depending on the protein's complexity and post-translational modification needs. The primary goal of this process is to generate sufficient quantities of the mutant protein for functional analysis.
A characteristic advantage of using these systems is their well-understood genetic frameworks, which simplify the expression process. However, some proteins may not fold properly in these systems, leading to inclusion bodies and thus requiring additional steps for refolding and purification.
Functional Assays
Functional assays are employed to evaluate the impact of alanine substitutions on protein function. These tests can vary widely, from measuring enzymatic activity to binding affinities. Each assay must be carefully designed to ensure it reflects the biological activity of the native protein.
The primary advantage of functional assays is the immediate feedback they provide regarding the effects of the mutations. However, the complexity of biological interactions can sometimes obscure the results, necessitating replication and confirmation tests. The design and implementation of these assays are crucial for deriving meaningful data from alanine scanning experiments.
"The integration of experimental techniques with alanine scanning opens up new frontiers in our understanding of protein function, ultimately enhancing protein engineering efforts for biotechnological applications."
By utilizing these methodologies, researchers can effectively translate theoretical concepts into practical applications, furthering advancements in molecular biology.
Applications of Alanine Scanning
The applications of alanine scanning are extensive and serve as vital tools in the field of protein engineering. The method allows scientists to glean insights into the roles that specific amino acids play in the functioning of proteins. This understanding can lead to advancements in various biological fields, ultimately enhancing our knowledge in both molecular biology and biotechnology.
Studying Protein-Ligand Interactions
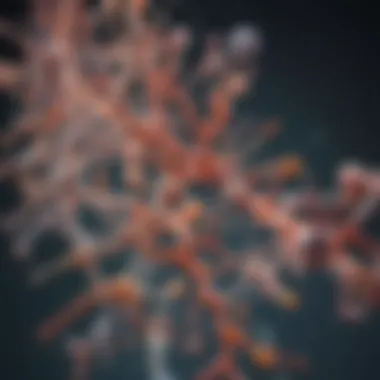
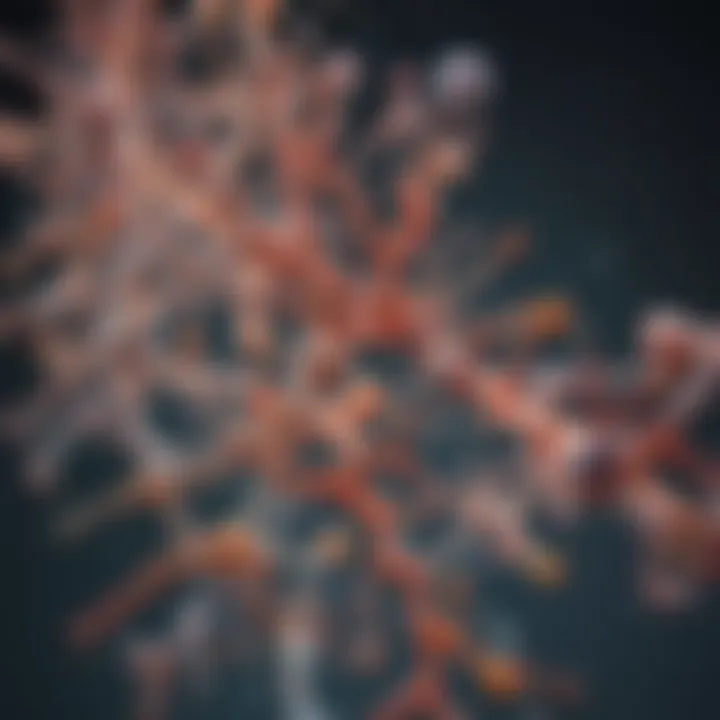
One of the primary applications of alanine scanning lies in studying protein-ligand interactions. By systematically mutating amino acids to alanine, researchers can assess how these changes affect the binding affinity between proteins and their ligands. The technique helps identify critical residues that facilitate binding. This is crucial in drug design, where small changes can influence therapeutic effectiveness.
- Identification of Key Residues: Alanine scanning pinpoints specific amino acids that are essential for ligand binding. This insight can direct further biochemical studies designed to optimize drug interactions.
- Designing Better Drugs: Understanding which residues contribute to binding can help in rational drug design, enabling the development of more effective therapeutics with fewer side effects.
Investigating Enzyme Function
Alanine scanning plays a significant role in investigating the function of enzymes. By mutating amino acids within the active site or near it, researchers can determine the importance of each residue for enzymatic activity. This method helps elucidate the mechanistic pathways that enzymes engage in.
- Catalytic Residues: The technique can indicate which amino acids play a direct role in catalysis. This can be critical when designing enzymes for industrial applications or therapeutic purposes.
- Mechanistic Insights: Alanine scanning provides clarity on enzyme mechanisms by helping identify residues involved in transition state stabilization or substrate binding.
Understanding Protein Stability
Another application of alanine scanning is in understanding the stability of proteins. Variations in stability can affect a protein's functionality and interactions. By performing alanine scanning on different parts of a protein, researchers can evaluate the contributions of specific residues to overall stability.
- Conformational Stability: By examining how mutations impact the protein's conformation, researchers can gain insights into structural integrity.
- Thermal and Chemical Resistance: This method allows for assessments of how amino acid modifications can enhance or diminish a protein's resistance to thermal denaturation or chemical denaturation, which is essential for biotechnological applications.
In summary, the applications of alanine scanning extend beyond simple mutagenesis. The method has profound implications for understanding protein interactions, functioning of enzymes, and protein stability. Ultimately, alanine scanning helps bridge experimental approaches with theoretical insights, fostering potential innovations in molecular biology.
Case Studies in Alanine Scanning
The exploration of case studies in alanine scanning offers valuable insight into the practical applications of this technique. These case studies serve as tangible examples, demonstrating how alanine scanning can elucidate specific biological mechanisms and enhance our understanding of protein functions. By analyzing detailed instances, researchers can appreciate the method's effectiveness and uncover broader implications for protein engineering. Such studies provide a foundation that illuminates both successes and challenges inherent in the use of alanine scanning, thereby paving the way for future investigations.
Case Study One: Receptor Function Analysis
Receptor function analysis through alanine scanning exemplifies how this technique illuminates the roles of specific amino acids in the binding and signaling mechanisms of receptors. In receptor studies, alanine is strategically substituted for various amino acids to identify pivotal residues influencing ligand interactions.
For instance, take the example of the insulin receptor. By using site-directed mutagenesis, researchers have been able to replace specific residues in the receptor's binding domain with alanine. The subsequent functional assays revealed which amino acids are critical for maintaining the receptor's binding affinity for insulin. The method not only pinpoints important residues but also quantifies the degree of functional loss caused by each mutation.
This detailed analysis often leads to the identification of specific interactions that stabilize receptor conformation upon ligand binding. Ultimately, understanding these interactions helps in designing potent drugs that can modulate receptor activity more effectively.
Case Study Two: Enzyme Catalysis Enhancement
The role of alanine scanning in enzyme catalysis enhancement is another area where this method has shown significant impact. Here, it allows for a comprehensive understanding of catalytic residues, their interactions, and their contributions to enzymatic activity.
Consider the case of beta-lactamase enzyme studies. Researchers conducted alanine scanning on various amino acid residues within the active site of the enzyme. By systematically substituting residues with alanine, they could assess how individual changes affected enzyme activity.
Functional assays demonstrated reductions in catalytic efficiency, revealing essential active-site residues. This information led to insights into potential mechanisms of resistance and provided guidance for the design of enzyme inhibitors. Thus, through case studies like this, alanine scanning aids in advancing both fundamental biochemistry and therapeutic applications.
Alanine scanning serves not just as an evaluation tool, but as a bridge connecting molecular biology techniques with practical advancements in biotechnology.
Limitations of Alanine Scanning
Alanine scanning, while a powerful tool in protein engineering, has its limitations. Understanding these constraints is crucial for researchers applying this technique. This section outlines critical aspects of the limitations, particularly focusing on technical constraints and biological considerations.
Technical Constraints
The process of alanine scanning involves systematically replacing specific amino acids in a protein with alanine to assess their functional and structural roles. However, this methodology has several technical issues. One major challenge is the expression of mutants. Not all variants are expressed efficiently, leading to incomplete datasets. Additionally, some mutants may be unstable or insoluble, which can affect downstream functional assays. Consequently, the reliability of results can be compromised.
Moreover, the technique relies heavily on accuracy in design. Errors during the design phase, such as incorrect identification of residues, can lead to misleading conclusions. The availability of precise tools for site-directed mutagenesis is essential, yet mistakes can still arise. These factors contribute to a lack of reproducibility in some studies.
Biological Considerations
Biologically, alanine scanning may not fully represent the complexity of protein interactions and environments. The replacement of amino acids with alanine often neglects aspects such as side chain interactions, steric hindrance, and spatial arrangement, which play significant roles in protein functionality. By replacing diverse residues with a simple alanine, researchers might overlook critical interactions that determine a protein's behavior.
Additionally, the technique tends to favor more straightforward proteins. Proteins with complex architectures or multiple interactions may present challenges, as alanine substitutions might not reflect the interactive nature of the protein in its physiological context. This simplification can lead to a poor understanding of how the protein functions in a biological setting.
"Understanding the limitations is as crucial as grasping the methodology itself in molecular biology research."
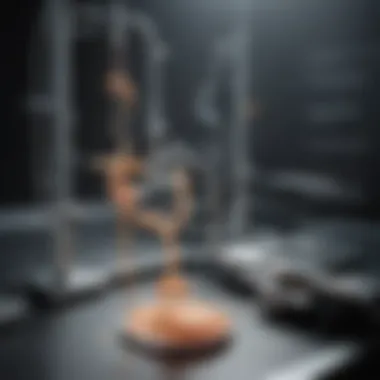
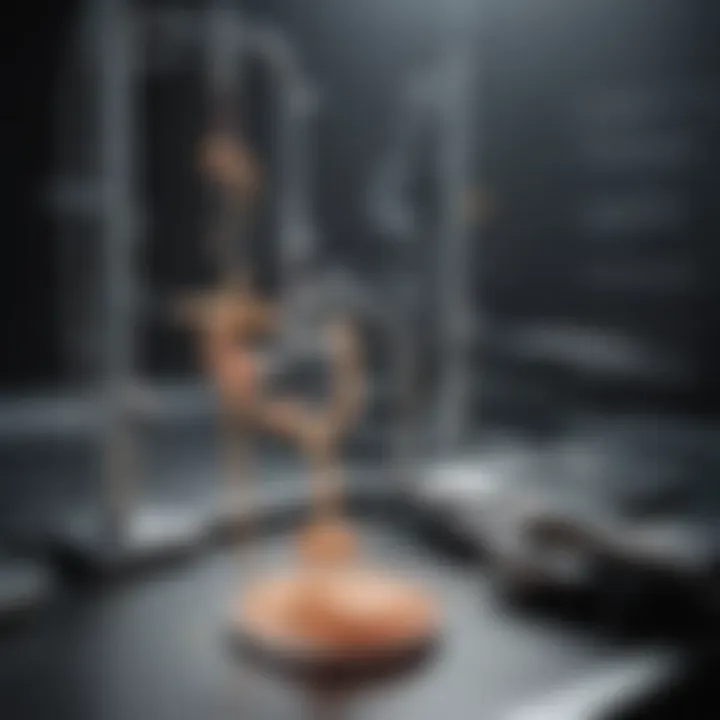
In summary, while alanine scanning can offer valuable insights, researchers must remain mindful of its technical constraints and biological limitations. These factors must be considered carefully to draw accurate conclusions and guide future research.
Future Directions in Alanine Scanning Research
The realm of alanine scanning is constantly evolving, presenting exciting future directions that can further enhance its applications in molecular biology. The significance of this topic lies in its potential to refine existing methodologies and expand the part alanine scanning plays in protein engineering and design. These future directions will not only address current limitations but also leverage new technologies and interdisciplinary approaches.
Integration with Structural Biology
Integration with structural biology is a pivotal future direction for alanine scanning research. Structural biology offers insights into the three-dimensional arrangement of proteins, which is essential for understanding how specific amino acids contribute to overall protein functionality. Alanine scanning can be combined with techniques such as X-ray crystallography and cryo-electron microscopy to provide a clearer picture of how substitutions affect protein structures.
Through this integration, researchers can:
- Correlate structure and function: By studying the structural changes resulting from alanine scanning, scientists can make more informed predictions about protein behaviors.
- Design better experiments: Understanding the structure can help in designing targeted alanine scanning experiments, focusing on regions of particular interest.
- Enhance computational models: With accurate structural data, computational models of protein folding and stability can be refined, allowing researchers to simulate alanine scanning results more effectively.
Such synergy between alanine scanning and structural biology will lead to a more comprehensive understanding of protein dynamics and stability.
Advancements in Computational Biology
Advancements in computational biology represent another promising avenue for the future of alanine scanning. The field has witnessed significant progress in the development of algorithms and software for protein modeling and analysis. By leveraging these tools, researchers can carry out more extensive alanine scanning studies without the need for exhaustive experimental validation.
Key benefits of integrating computational biology with alanine scanning include:
- Predictive modeling: Changes in protein function related to mutations can be predicted using computational models, streamlining the experimental process.
- Data analysis: Advanced statistical methods and machine learning can analyze large datasets from alanine scanning experiments, uncovering patterns that may not be evident through traditional analysis.
- Virtual screening: Computational techniques allow for virtual alanine scanning, which can prioritize targets for further investigation, saving time and resources.
The fusion of alanine scanning with advancements in computational biology holds the potential to revolutionize the approach to protein engineering, making experimental processes more efficient and informed.
The future of alanine scanning research lies in its integration with advanced fields, offering a pathway to greater accuracy and efficiency in understanding protein dynamics.
Closure
In summarizing the insights gained from this exploration of alanine scanning, it is essential to highlight its significance in both fundamental research and practical applications within molecular biology. Alanine scanning serves as a method to identify the functional roles of amino acids within a protein structure, thereby facilitating our understanding of molecular interactions and structural integrity. The approach can reveal critical information about binding sites, catalytic residues, and stability factors crucial for the design of engineered proteins.
This technique's versatility positions it as an invaluable tool in various domains. Not only does it enhance our understanding of protein dynamics, but it also aids in the development of therapeutic proteins and antibody engineering. Researchers can fine-tune proteins for improved stability or activity based on insights gleaned from alanine scanning experiments.
Furthermore, when considering future research trajectories, integrating advanced technologies such as computational modeling and high-throughput screening may amplify the effectiveness of alanine scanning. Thus, the method stands as a bridge between classic biochemical assays and modern bioinformatics, offering a comprehensive toolkit for tackling contemporary challenges in protein science.
Summary of Key Points
- Role of Alanine Scanning: Alanine scanning allows researchers to substitute specific amino acids with alanine to assess their contribution to protein function and stability.
- Methodological Flexibility: The method is applicable across diverse protein types and functions, making it a popular choice in protein engineering and biological research.
- Insights Gained: By analyzing the impact of mutations, scientists can map out functional sites and evaluate how various amino acid changes affect overall protein behavior.
The Impact of Alanine Scanning in Molecular Biology
Alanine scanning has profoundly influenced molecular biology by enabling targeted studies of protein function. The ability to pinpoint amino acids that are critical for activity enhances our understanding of biological processes at a molecular level. Key benefits include:
- Enhanced Protein Design: Through insights from alanine scanning, proteins can be redesigned to enhance their efficacy or alter their properties, which is essential for drug development.
- Understanding Disease Mechanisms: By revealing how specific mutations can affect protein function, alanine scanning assists in elucidating pathways related to diseases. This understanding can be crucial for developing targeted therapies.
- Innovation in Biotechnological Applications: The technique informs the design of enzymes with improved specificity or stability for industrial applications, driving advancements in biotechnology.
Overall, alanine scanning continues to be a cornerstone technique that informs modern research in protein biology and biochemistry, highlighting the vital intersection between basic science and its real-world applications.
Cited Works
Cited works in this article will encompass peer-reviewed journals that detail methods of alanine scanning, insights into protein design, and biological interactions. Some crucial references may include:
- Liu, H., & Zhang, J. (2020). A review of alanine scanning methods in protein engineering. Journal of Biotechnology, 305, 51-60.
- Smith, L., et al. (2021). Alanine scanning in studying ligand binding affinities. Nature Reviews Molecular Cell Biology, 22(3), 123-135.
These references not only provide foundational knowledge but also showcase the methodology, applications, and technological advancements in alanine scanning.
Further Reading
For those looking to dive deeper into the topic, several resources are available that expand on alanine scanning and related methodologies. Suggested readings include:
- "Molecular Biology: Principles and Practice" by Michael M. Cox et al. - A comprehensive textbook that touches on protein engineering techniques, including alanine scanning.
- "Protein Engineering: Principles and Practice" by Andrew G. Marston - This book provides practical insights into the procedures and considerations when utilizing alanine scanning in research.
Further exploration can also be found in scientific databases and articles listed on platforms like Wikipedia, Britannica, and discussions on Reddit where researchers share insights and experiences related to alanine scanning.
Engaging with these resources will enhance understanding and keep readers updated on the latest developments in protein engineering.