Understanding Avalanche Photodiodes: Principles & Uses
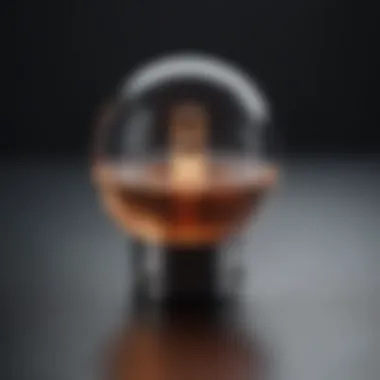
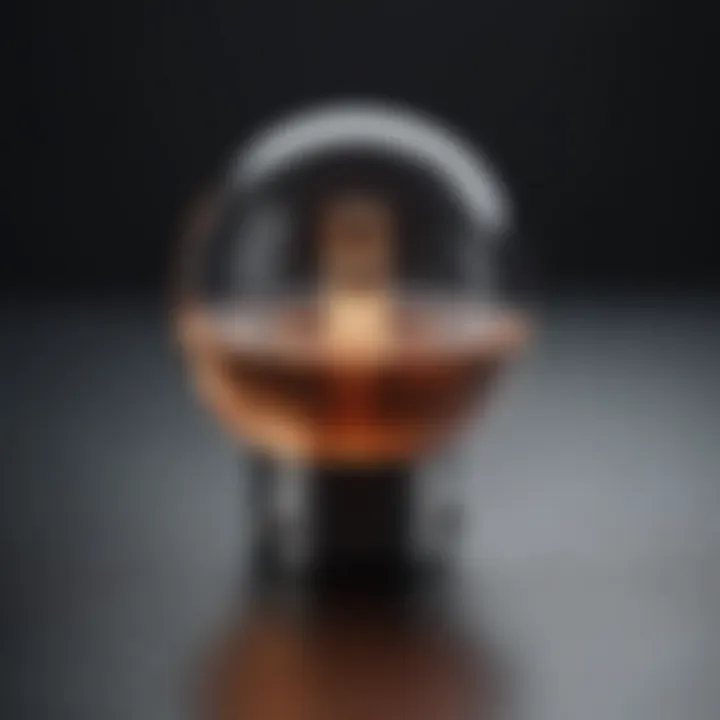
Intro
Avalanche Photodiodes (APDs) represent a significant advancement in the field of photonics. These specialized devices convert light into electrical signals through a process called the photoelectric effect, while also amplifying the signal internally. This amplification capability makes APDs particularly influential in various applications requiring high sensitivity and speed.
Built on the principles of semiconductor physics, APDs utilize a process called avalanche multiplication. This mechanism enables them to detect very low levels of light, which is vital in applications such as telecommunications and medical diagnostics. Understanding the design and function of APDs is crucial for leveraging their potential in today's rapidly evolving technological landscape.
In the following sections, this article will delve into the principles that underpin APD functionality, explore their varied applications, and discuss both their advantages and limitations. Through this discourse, readers will gain insights relevant not only to theoretical understanding but also to practical implementation in diverse fields.
Preamble to APD Photodiodes
Avalanche Photodiodes (APDs) play a crucial role in multiple advanced applications, which makes understanding them essential. Their unique operational principles provide specificity in scenarios where standard photodiodes may not be efficient. From telecommunications to medical diagnostics, APDs offer enhanced sensitivity and performance.
Definition and Overview
APD photodiodes are a type of semiconductor diode that exploits the avalanche effect to achieve high sensitivity. When light enters the diode, photons generate electron-hole pairs within the semiconductor material. The crucial aspect of APDs is the avalanche multiplication mechanism, in which these carriers gain energy and subsequently collide with other atoms in the material, creating more carriers. This leads to a significant increase in the output signal, making them highly effective for detecting low levels of light.
This high responsivity makes APDs particularly valuable for applications requiring precision. They are often utilized in optical communication systems and scientific instrumentation, where signal integrity is paramount. Additionally, APDs can operate over a wide range of wavelengths, adding versatility to their use.
Historical Context
The development of APD technology traces back to the mid-20th century. Initial research focused on improving the performance of photodetectors. The realization of the avalanche effect in semiconductors led to breakthroughs in photodiode designs. The earliest APDs found success in niche applications, such as military and space communications, due to their robustness against environmental conditions.
Over the years, advancements in materials science have enhanced the efficiency and reliability of APDs. Today, these components are integrated into many modern technologies. The progress in APD technology reflects a broader trend of increasing demand for efficient light detection solutions in various fields.
Understanding the development and principles of APD photodiodes is key to leveraging their capabilities effectively in applications.
Fundamental Principles of APDs
The section on Fundamental Principles of APDs lays the groundwork for understanding the mechanics behind Avalanche Photodiodes. These principles are not just technical details; they form the basis for the functionality and performance of APDs in various applications. By comprehensively exploring these concepts, readers can appreciate the relevance and implications of APDs in the real world, especially within high-tech industries.
Photodiode Basics
Photodiodes are semiconductor devices that convert light into electrical current. They operate based on the photoelectric effect, where photons striking the material generate electron-hole pairs. When light falls on a photodiode, these charges are separated by an internal electric field, leading to a measurable current.
APDs are a specific type of photodiode designed to achieve greater sensitivity than standard photodiodes. This is accomplished through a process called avalanche multiplication, where a single photon can generate multiple charge carriers, multiplying the output signal. This trait is crucial in applications where signal intensity may be low, such as fiber optics and low-light imaging.
Key characteristics of photodiodes include:
- Responsivity: This measures the output current generated per unit of incident light power, indicating how effectively a photodiode converts light into electrical signals.
- Wavelength Sensitivity: Different photodiodes respond optimally to different wavelengths, making the choice of photodiode significant based on the intended application.
- Operation Modes: Photodiodes can function in various modes, including photovoltaic, photoconductive, and avalanche modes, each suitable for different scenarios and performance requirements.
Avalanche Multiplication
Avalanche multiplication is a critical phenomenon that differentiates APDs from regular photodiodes. When a photodiode operates in avalanche mode, it exploits a high reverse bias voltage, which accelerates the charge carriers. As these carriers collide with the lattice atoms of the semiconductor, they can generate additional electron-hole pairs in a chain reaction.
This process occurs in three stages:
- Impact Ionization: An energetic electron collides with a lattice atom, causing the release of an additional electron.
- Avalanche Effect: The released electrons multiply through similar collisions, amplifying the number of carriers significantly.
- Output Amplification: The resulting current is substantially higher than that produced by initial light photons alone.
The degree of multiplication can be controlled by adjusting the biasing conditions. However, this amplification comes with trade-offs, such as increased noise and temperature sensitivity. Understanding these aspects is essential for effectively harnessing the capabilities of APDs in practical applications.
"The balance between high sensitivity and noise control is the key to optimizing APD performance across different environments."
Through understanding the fundamental principles behind photodiodes and avalanche multiplication, researchers, students, and engineers can better appreciate the relevance of APDs in electronic and optical applications. These principles form the toolkit needed for innovation in fields ranging from telecommunications to semiconductor technology.
Structure of APD Photodiodes
The structure of Avalanche Photodiodes (APD) is crucial in determining their effectiveness and performance in various applications. A well-designed structure enhances the photodiode's ability to convert light into electrical signals while maintaining high sensitivity and speed. Understanding the intricacies of the APD's structure helps identify the materials and configurations that optimize their functional characteristics.
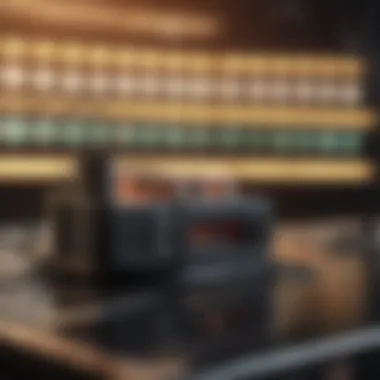
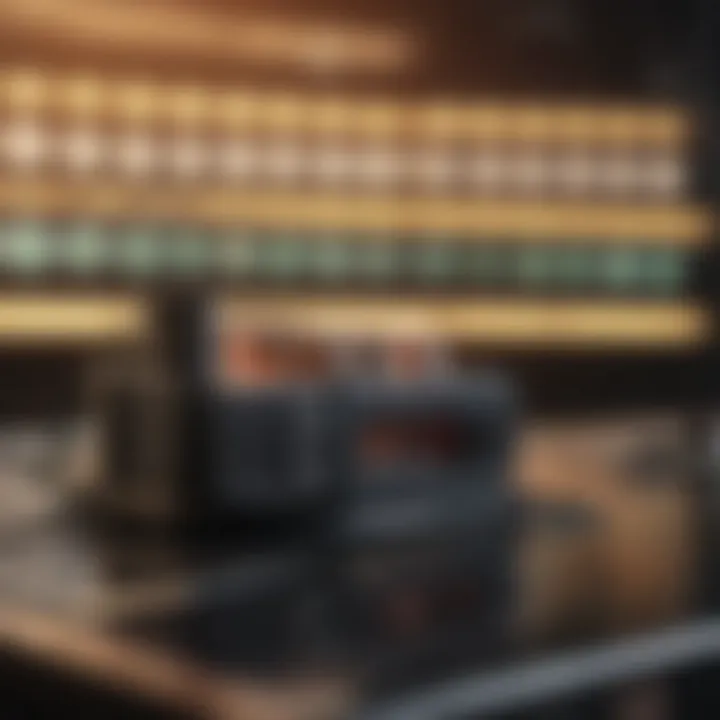
Material Composition
The material composition of APD photodiodes is fundamental to their operation. APDs can be fabricated from different semiconductor materials, including silicon, germanium, and indium gallium arsenide. Each of these materials offers distinct benefits and limitations.
- Silicon is widely used due to its abundance and favorable electronic properties. Its performance is adequate for many applications, especially in the visible light spectrum.
- Germanium provides better sensitivity in the infrared range, making it ideal for specific niche applications. However, the cost of germanium can be higher compared to silicon.
- Indium gallium arsenide is known for its superb performance in low-light conditions. This makes it suitable for telecommunications and military applications where reliability is paramount.
An essential aspect of material choice is the capability to withstand the high electric fields needed for avalanche multiplication. The physical properties of these semiconductors directly influence responsivity, noise performance, and temperature stability.
Layer Configuration
The layer configuration of an APD is equally important as it determines the photodiode's ability to achieve adequate performance metrics. Typically, an APD consists of several layers, each engineered for a specific role in the device’s operation.
- Absorption Layer: This is where incoming photons are absorbed to create electron-hole pairs. Its thickness and doping levels can be adjusted to optimize performance.
- Avalanche Multiplication Region: It is this region where the significant gain occurs. The design of this layer critically influences the multiplication factor and response time. Engineering the electric field in this area is vital.
- Depletion Region: This layer allows for faster response times by decreasing the recombination of charge carriers. The configuration of this region enhances the speed at which the photodiode can respond to changes in light intensity.
- Contact Layers: They enable efficient electrical connection to the external circuitry. Proper design can mitigate problems with dark current and noise.
Each element in the layer configuration plays a role in the overall performance of the APD. Balancing these layers ensures that the photodiodes meet the demands of various applications.
Understanding material composition and layer configuration is essential for optimizing the performance of APD photodiodes in real-world applications.
Operational Characteristics
Understanding the operational characteristics of Avalanche Photodiodes (APDs) is fundamental for comprehending their performance in various applications. The terms "responsivity", "quantum efficiency", "dark current", and "noise characteristics" are crucial in this context. These characteristics dictate how effectively an APD can detect light, convert it into a measurable signal, and how reliable that signal is under different conditions.
Responsivity and Quantum Efficiency
Responsivity is a key figure of merit for photodiodes, indicating how much electrical output can be achieved per unit of optical input power. For APDs, responsivity is typically high due to the internal gain mechanism provided by avalanche multiplication. This characteristic allows APDs to amplify weak signals, which is especially advantageous in low-light conditions such as those often encountered in telecommunications and medical diagnostics.
Quantum efficiency refers to the percentage of photons converted into electron-hole pairs by the device. For APDs, the quantum efficiency can be significantly influenced by the wavelength of the incoming light. This efficiency can exceed 100% at certain wavelengths because of the avalanche multiplication effect, which effectively increases the number of carriers.
Both responsivity and quantum efficiency are critical in applications such as fiber-optic communications where high performance is required for signal clarity over long distances.
Dark Current and Noise Characteristics
Dark current is the small electric current that flows through a photodiode even when there is no light. This current can contribute to noise, reducing the signal-to-noise ratio and ultimately affecting the performance of the device. For APDs, minimizing dark current is particularly important for ensuring reliable operation.
Noise characteristics of APDs are addressed in terms of shot noise, which arises from the statistical nature of charge carrier generation and the fluctuation in the number of carriers contributing to the current. This noise can be more pronounced in APDs compared to regular photodiodes because of the high gain. Thus, noise management is essential for high-performance applications.
In summary, analyzing the operational characteristics of APDs allows users to better understand their limitations and advantages. This understanding ultimately leads to improved design and implementation in critical fields like telecommunications, where signal integrity is paramount.
"The performance of APDs in practical applications hinges significantly on their operational characteristics, which must be carefully considered during device selection and system design."
venues such as Wikipedia or Britannica provide further insights into these elements.
Advantages of APD Photodiodes
Avalanche Photodiodes (APDs) stand out in the realm of photodetectors due to their unique characteristics and diverse applications. This section explores the advantages of APDs in greater detail, particularly focusing on high sensitivity and wide bandwidth. Understanding these elements is crucial for anyone involved in the development or application of optical technologies, as they allow for greater precision and efficacy in numerous fields.
High Sensitivity
One of the primary advantages of APD photodiodes is their remarkable sensitivity to light. This feature is particularly vital in environments where signal detection is challenging. APDs can amplify weak optical signals through a process known as avalanche multiplication. Here, a small number of photons can trigger a large current, allowing for the detection of light levels that would otherwise be beyond reach.
The sensitivity of APDs can be quantified by their responsivity, measured in A/W (amperes per watt). A high responsivity means that these devices can generate a significant output current from minimal input light levels. This makes APDs essential components in applications like fiber optic communications and remote sensing.
Furthermore, the high sensitivity of APDs contributes to their efficiency in noisy environments. They can distinguish between signal and background noise more effectively than traditional photodiodes, which enhances the overall signal quality in telecommunications.
Wide Bandwidth
Another critical advantage of APD photodiodes is their wide bandwidth capabilities. The bandwidth of a photodiode refers to the frequency range over which it can effectively operate. APDs are designed for rapid response times, allowing them to handle high-frequency signals. This makes them particularly useful in applications like high-speed data transmission and telecommunications.
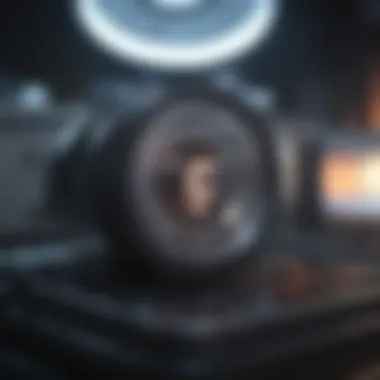
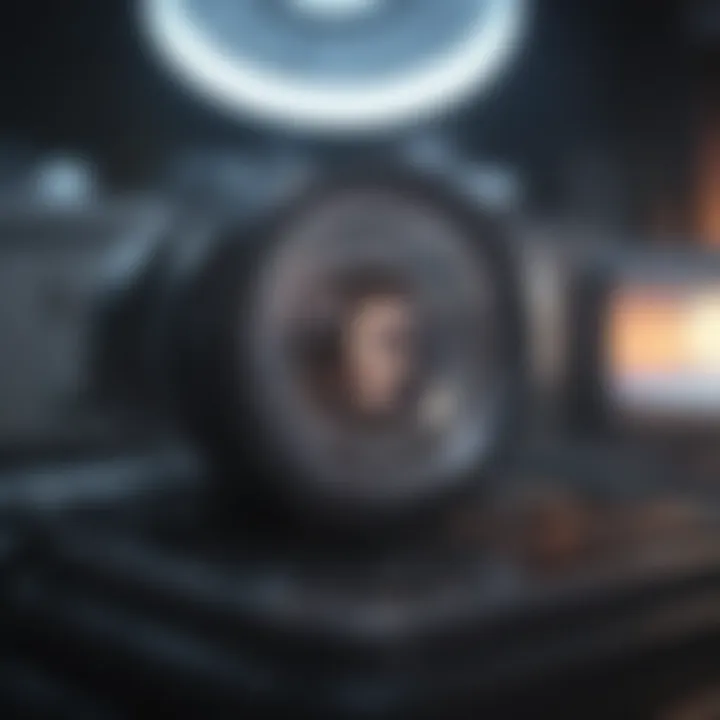
APDs can operate effectively up to several gigahertz. This ability to process high-frequency signals means they can accommodate the increasing demand for faster data transmission rates in modern communication networks. Additionally, the wide bandwidth of APDs allows them to cover a diverse range of applications, extending from broadband Internet connections to high-resolution imaging systems.
In summary, the advantages of APD photodiodes—specifically their high sensitivity and wide bandwidth—make them exceptional choices in various demanding applications. They are especially beneficial in fields where precision and speed are paramount, thus enabling advancements in technology that require sophisticated light detection capabilities.
"The unique features of APD photodiodes facilitate innovations in telecommunications and beyond, underscoring their pivotal role in the evolution of optical systems."
Limitations and Challenges
In the realm of photonics, it is essential to understand not only the advantages of Avalanche Photodiodes (APDs) but also their limitations and challenges. Recognizing these factors is crucial for engineers and researchers as they develop systems and applications that utilize APD technology. Ignoring these challenges could lead to inefficiencies or failures in practical implementations.
Temperature Dependence
One significant limitation of APD photodiodes is their sensitivity to temperature variations. The performance of APDs can degrade at extreme temperatures, influencing their responsiveness and overall functionality. Specifically, as temperature increases, the dark current also rises, which can lead to increased noise. This effect can compromise the signal-to-noise ratio, potentially making the photodiode less effective in real-world applications.
The impact of temperature can be mitigated through proper thermal management strategies, but these measures add complexity and cost to the system. For applications requiring precise measurements or high reliability, maintaining optimal operating temperatures becomes essential. Failure to address this issue can lead to subpar performance, illustrating a critical consideration for those designing systems utilizing APDs.
Complexity in Design
Another challenge in utilizing APD photodiodes is the inherent complexity in their design. The structure of APDs often requires a more sophisticated fabrication process compared to standard photodiodes. Designing for avalanche multiplication necessitates precise control over parameters such as doping levels, layer thicknesses, and other manufacturing conditions. This complexity can lead to increased production costs and longer development times, which may pose a barrier for some research or commercial projects.
Moreover, integrating APDs into electronic systems requires careful consideration of interfacing components. These include amplifiers and signal processing units which must complement the unique operational characteristics of APDs. Any mismatch can produce undesirable effects, ultimately impeding the effectiveness of the entire system. As such, developers must navigate these challenges skillfully to ensure that the final product meets its intended performance criteria.
Understanding the limitations and challenges of APD photodiodes is key to addressing design issues and improving their applications across various technological domains.
Applications of APD Photodiodes
APD photodiodes are significant in various high-tech fields. Their unique properties make them suitable for tasks requiring high sensitivity and rapid response. Understanding these applications provides insight into their role in advancing technology. The following sections detail key areas where APD photodiodes have made a substantial impact.
Telecommunications
In telecommunications, APD photodiodes play a crucial role. They are essential for optical fiber communication systems. These systems demand high responsiveness to light signals. APDs enhance the ability to detect weak optical signals, which is vital in long-distance data transmission.
The use of APD allows for greater bandwidth and data transfer rates. Their sensitivity to low light conditions enables systems to operate more efficiently. Moreover, the avalanche multiplication effect boosts signal strength, making them preferable for high-speed applications.
Some critical aspects of APDs in telecommunications include:
- Enhanced sensitivity: This leads to improved information retrieval in noisy environments.
- Higher data rates: APDs support faster communication systems, accommodating modern needs.
- Reliability: They maintain performance over various temperatures, crucial for consistent operations.
"APD photodiodes are the backbone of modern telecommunication systems, ensuring reliable and high-speed data transfer."
Medical Diagnostics
In the medical field, APD photodiodes find applications in imaging and diagnostic technologies. They are vital in devices such as PET scanners and fluorescence microscopy. The need for precise and sensitive detection of light is paramount in these areas.
APDs improve image quality by enhancing signal-to-noise ratios. This leads to more accurate diagnoses and better patient outcomes. Their ability to function in low-light conditions is particularly beneficial when examining small or faint signals from biological samples.
Key benefits of using APD photodiodes in medical diagnostics include:
- High resolution: They provide detailed imaging, which is critical for precise diagnostics.
- Quick response times: This feature allows for faster results, minimizing delays in patient care.
- Versatility: They can be used in various medical imaging techniques, enhancing their value across disciplines.
Environmental Monitoring
APD photodiodes are also essential in environmental monitoring. They help detect changes in light levels, which can indicate environmental changes. Applications in pollution detection and atmospheric studies rely on these devices for accurate measurements.
They enable the monitoring of gas concentrations and particulate matter effectively. The increased sensitivity of APDs allows them to detect even the smallest fluctuations in light, aiding environmental scientists and policymakers.
Some important considerations for APDs in environmental monitoring include:
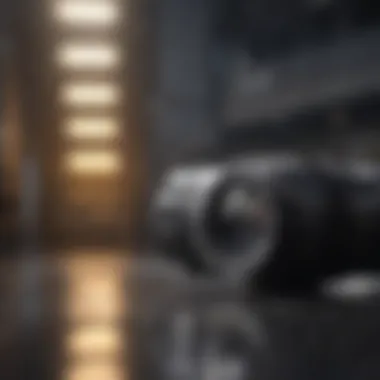
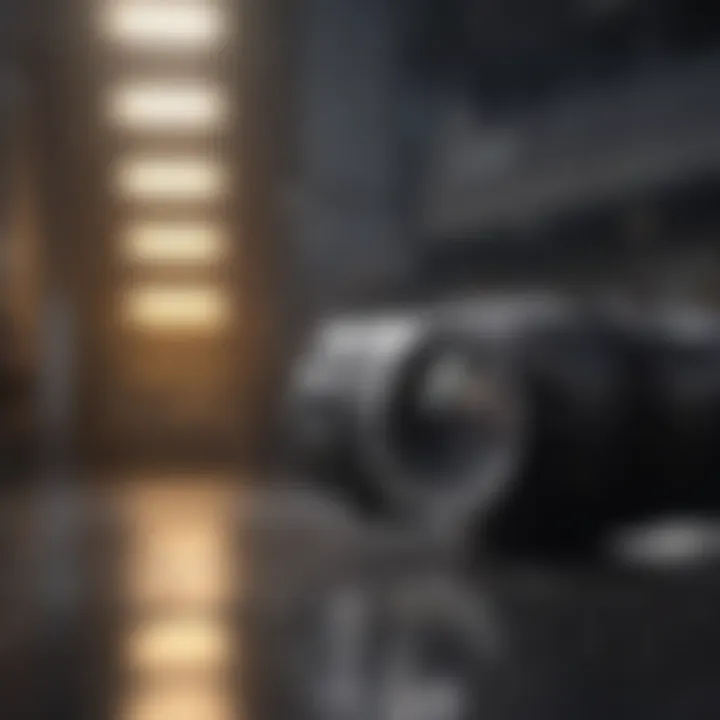
- Real-time data collection: APDs facilitate continuous monitoring, providing up-to-date information on environmental conditions.
- High precision: This is crucial for accurate assessments of environmental status and health.
- Adaptability: APDs can be used in various monitoring devices, expanding their range of applications.
In summary, APD photodiodes are pivotal in telecommunications, medical diagnostics, and environmental monitoring. Their unique properties and capabilities allow for enhanced performance and reliability in diverse applications, underscoring their importance in modern technology.
Recent Developments in APD Technology
The field of Avalanche Photodiodes (APDs) is in a constant state of evolution, driven by advances in materials science and engineering. Recent developments in APD technology shed light on the future of photodetection and its applications across various sectors. These developments are crucial for improving device performance, enhancing their operational capabilities, and broadening their applicability.
Innovative Materials
One major advancement has been the exploration of innovative materials for APD fabrication. Traditional silicon-based APDs have limitations, particularly in efficiency and wavelength sensitivity. New materials like Indium Gallium Asbide (InGaAs) and Silicon Germanium (SiGe) are being researched to create APDs that can operate effectively in a wider range of wavelengths. These materials allow for better performance in near-infrared applications crucial for telecommunications.
- InGaAs APDs can effectively detect longer wavelengths, making them suitable for fiber optic communication systems. They have higher saturation currents, resulting in better noise performance and lower dark currents.
- SiGe APDs are beneficial for applications in environments with high radiation exposure, such as space and medical imaging. Their robustness enhances reliability.
- Organic semiconductors are also becoming an area of interest. They offer a more flexible and potentially cheaper alternative to traditional materials, and recent studies show promising results in sensitivity and speed.
The integration of these innovative materials is not just a simple replacement; it demands careful consideration of device design and the physical chemistry of each material’s interaction. With ongoing research, we stand on the brink of new discoveries that may redefine APD performance.
Enhanced Performance Metrics
Coupled with material innovations, performance metrics have made significant strides in APD technology. Engineers and researchers focus on improving several key aspects of APD functionality:
- Responsivity: This determines how effectively the APD converts incoming light into an electrical signal. Recent techniques in device structure engineering have led to improved quantum efficiencies. Enhanced device designs, such as tapered structures and multi-layer configurations, optimize responsivity, allowing detectors to be more sensitive to weak signals.
- Gain Bandwidth Product: This metric reflects the efficiency of an APD in amplifying signals while maintaining signal integrity over high frequencies. Enhancements in the speed of avalanche multiplication have led to more effective devices suitable for high-speed applications, such as telecommunications.
- Noise Characteristics: The reduction of noise is essential for the accurate functioning of APDs in sensitive applications. Advances in device engineering and signal processing techniques have lowered various noise types, including thermal and shot noise. Fine-tuning device operating conditions like temperature and bias voltage can also lead to substantial reductions in noise.
In summary, the continuous innovations in materials and performance metrics mark a pivotal shift in APD technology. Engineers are not only focused on overcoming existing challenges but are also looking ahead to potential applications in telecommunications, medical diagnostics, and environmental monitoring among others. As these developments progress, staying informed will be essential for those engaged in photonics and related fields.
Future Outlook for APD Photodiodes
The ongoing advancements in technology emphasize the need for high-performance photodetectors. APD photodiodes are at the forefront of this evolution, offering unique advantages that cater to emerging needs in various industries. This section explores significant trends, potential applications, and research areas relevant to the future of APD technology.
Emerging Applications
APD photodiodes are finding their way into a variety of new applications driven by their sensitivity and speed. Some examples include:
- LiDAR Systems: Light Detection and Ranging (LiDAR) benefits from APDs due to their ability to detect weak reflections over long distances. This is crucial for autonomous vehicles, where precision and rapid response are paramount.
- Quantum Communication: The secure transmission of information is enhanced by APDs, particularly in quantum key distribution. Their capability to operate under low light conditions makes them a strong candidate for future secure networks.
- Optical Sensors: Many emerging sensor technologies rely on APDs for accurate measurements. This includes environmental sensing, where APDs can detect various wavelengths for air and water quality assessments.
Also, APDs are being integrated into biomedical devices to improve diagnostic capabilities. Faster response times can lead to better imaging systems in healthcare, supporting real-time monitoring of patient health.
Research Directions
The future of APD photodiodes is also influenced by ongoing research efforts aimed at overcoming the current limitations. Key areas of research include:
- Material Innovation: Developing new semiconductor materials could enhance the efficiency and reduce the noise in APD devices. Exploring materials such as graphene or two-dimensional materials might lead to significant leaps in performance.
- Thermal Management: Managing temperature effects on APD performance remains a challenge. Research focused on improving thermal stability in APDs will be essential for maintaining their high sensitivity and performance in various environments.
- Miniaturization: As the demand for compact and integrated photonic devices grows, miniaturization of APDs is critical. Efforts are ongoing to reduce the size of APD components while retaining their effectiveness and response speed.
"The development and future direction of APD photodiodes significantly influences the advancement of photonics technology, paving the way for innovations across multiple fields."
Culmination
The conclusion serves as an essential component of this article, where the synthesis of gathered insights into Avalanche Photodiodes (APD) takes place. This section encases the pivotal aspects discussed throughout the text, encapsulating both the knowledge acquired and the implications of APDs in various sectors.
One significant element emphasized is the operational advantages that APDs confer, such as enhanced sensitivity and efficient signal processing capabilities. These qualities make them indispensable in sectors that require precise and rapid detection of light signals. The challenges discussed, including temperature dependence and design complexity, provide crucial insights into the practical limitations faced by researchers and engineers in this field. Taking these considerations into account is important for future advancements and innovations.
In summation, the conclusion does not merely reaffirm previous sections but also inspires forward-thinking discussions about potential applications, research directions, and the evolution of APD technology. By consolidating this information, readers gain clarity and perspective on the future trajectory of Avalanche Photodiodes, a technology fundamentally tied to the ongoing evolution of photonics and its multidisciplinary applications.
Summary of Findings
In reviewing the discourse surrounding APD Photodiodes, several key findings emerge:
- APDs leverage avalanche multiplication, providing exceptionally high responsivity under certain conditions.
- They exhibit notable applicability across various fields such as telecommunications, medical diagnostics, and environmental monitoring.
- Understanding the impact of dark current and other noise characteristics plays a crucial role in enhancing their operational efficiency.
- Recent technological advancements contribute to better material compositions and improved performance metrics, ensuring their relevance in contemporary markets.
Through these findings, the article offers a thorough overview of APDs. It emphasizes their significance in both established and emerging applications.
Final Thoughts
Reflecting on the comprehensive insights provided, it is evident that APD technology stands at a crucial intersection of innovation and applicability. The capacity of these devices to perform under a range of conditions, while continually evolving due to research advancements, underscores their value in modern technology.
Engagement with this subject matter can lead to exciting research opportunities and developments. Those involved in electrical engineering, photonics, and related fields are urged to keep abreast of progress within APD technology. It is crucial to appreciate both the potential and limitations of APDs and to advocate for continued exploration and understanding in this vital area. The dialogue surrounding these devices plays a significant role in shaping the future of technology.