Understanding cDNA Reverse Transcription: Methods and Applications
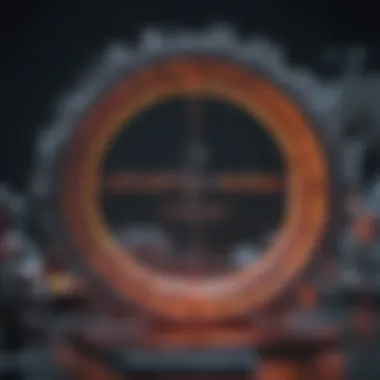
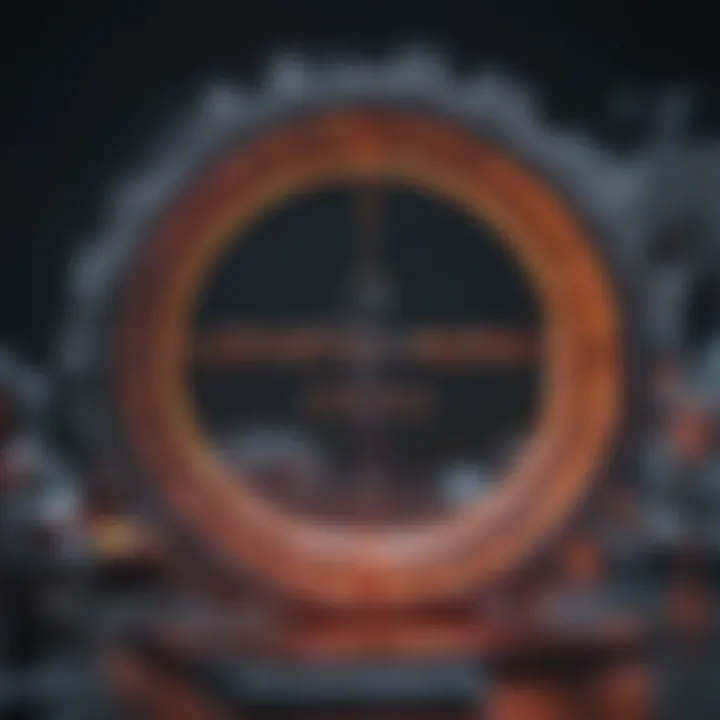
Intro
cDNA reverse transcription plays a crucial role in the field of molecular biology. Understanding this process is essential for various scientific endeavors. At its core, reverse transcription is the conversion of RNA into complementary DNA (cDNA). This action serves as a critical link between gene expression and molecular analysis.
The rapid development of new technologies has propelled cDNA synthesis into prominent applications. Its use extends from basic research to practical applications in diagnostics and therapeutic development. Researchers and professionals must grasp this complex but fascinating subject.
In this article, we aim to overview the comprehensive aspects surrounding cDNA reverse transcription, detailing the methods used, the importance in different fields, and directions for future research.
Research Highlights
Key Findings
- cDNA synthesis is foundational for studying gene expression. It allows scientists to analyze mRNA levels across various conditions.
- Different enzymes, such as reverse transcriptases, play a critical role in cDNA synthesis accuracy and yield.
- Advanced techniques have improved the efficiency of reverse transcription, significantly impacting research outcomes.
Implications and Applications
The applications of cDNA reverse transcription are vast and interdisciplinary. These include:
- Gene expression analysis: Essential in studying how genes respond to stimuli.
- Diagnostics: Used in detecting viral infections where RNA is the primary genetic material, e.g., SARS-CoV-2.
- Therapeutic development: Facilitates drug discovery by enabling understanding of gene impacts.
"cDNA reverse transcription is not just a technique; it is a bridge between genetic data and its biological implications."
Methodology Overview
Research Design
The design and approach in studying cDNA reverse transcription typically involve systematic protocols to ensure reproducibility. Factors like temperature and enzyme choice significantly affect the outcome, influencing the quality of the synthesized cDNA.
Experimental Procedures
- Selection of RNA: Quality of RNA is paramount. Isolated RNA should be free from contaminants.
- Enzyme Selection: Choosing appropriate reverse transcriptases is critical for successful reaction.
- Reaction Conditions: Predefined conditions regarding temperature and buffer composition must be adhered to for optimal results.
- cDNA Amplification: Subsequent amplification of cDNA allows for quantitative analysis and further investigation.
Understanding the intricacies of these methodologies enlightens the significant workflows involved. In addition, we will explore advancements within this field and examine challenges faced by current practices.
Prolusion to cDNA Reverse Transcription
cDNA reverse transcription is a vital process in biotechnology and molecular biology. It enables researchers to create complementary DNA from RNA, serving as a foundation for various experimental techniques. Understanding this process is essential for investigating gene expression, developing diagnostics, and implementing therapeutic solutions. The significance of cDNA reverse transcription extends far beyond academic inquiries; it fundamentally shapes the landscape of modern biological research and applications. The methods used for cDNA synthesis can greatly affect the quality and outcome of subsequent analyses, making it crucial for practitioners to grasp both its methodologies and implications.
Definition and Importance
cDNA, or complementary DNA, is synthesized through a process known as reverse transcription. This involves the enzyme reverse transcriptase, which transcribes RNA into DNA. The ability to convert RNA into cDNA allows for the more stable and manageable analysis of genetic material. cDNA is pivotal in various experiments, including quantitative PCR, cloning, and sequencing, where high-quality DNA is required. The importance of this process is evident in its applications across multiple fields, including medicine and genetics. The accurate quantification of RNA levels gives insight into gene expression, making it indispensable for studies related to development, disease, and treatment responses.
Historical Context
The concept of reverse transcription was introduced in the early 1970s. Howard Temin and David Baltimore independently discovered the enzyme reverse transcriptase. Their work earned them the Nobel Prize in Physiology or Medicine in 1975. Initially observed in retroviruses, such as HIV, reverse transcription highlighted a unique mechanism through which viruses could integrate their genetic material into host cells.
Following these groundbreaking discoveries, the capabilities of cDNA synthesis began to expand in the following decades. Researchers started applying cDNA in various molecular techniques, significantly enhancing genetic research's depth and breadth. Over time, innovations in the methodologies used for cDNA synthesis have led to more efficient and reliable protocols, aiding in everything from basic research to practical medical applications. Understanding this progression helps underline the ongoing relevance and potential of cDNA reverse transcription in future studies and developments.
Fundamentals of Reverse Transcription
Reverse transcription stands as a cornerstone of molecular biology, pivotal for various applications that span research, diagnostics, and therapeutic developments. Understanding the fundamentals of this process is critical, not only because it enables the conversion of RNA into complementary DNA (cDNA) but also because it opens the door to numerous techniques employed in genetic analysis. The foundational knowledge of reverse transcription allows researchers to harness this mechanism effectively when interpreting gene expression or exploring genetic disorders.
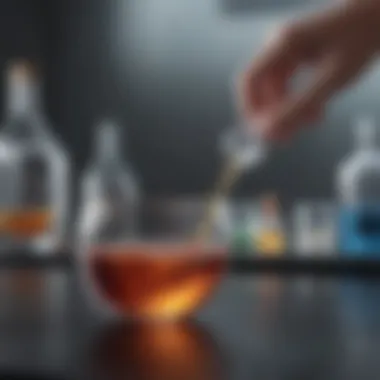
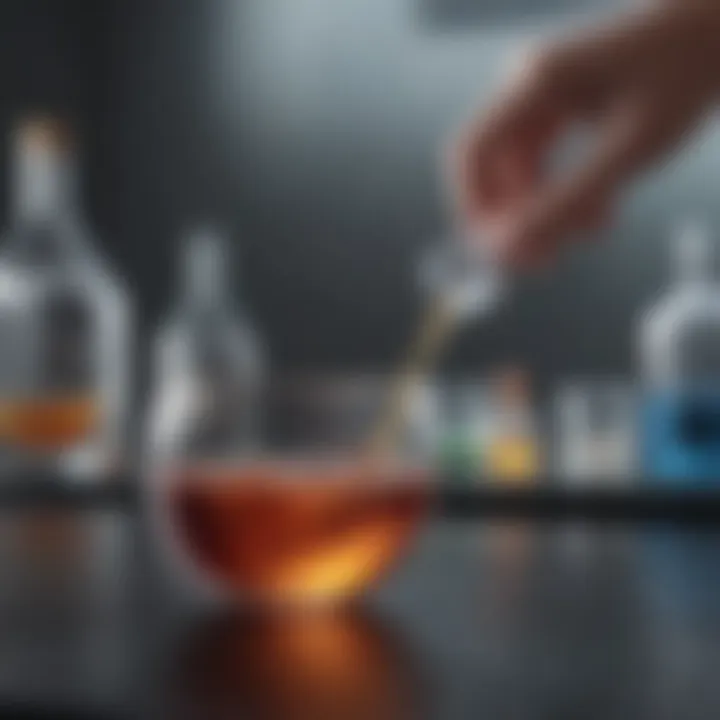
Mechanism of Reverse Transcription
At its core, reverse transcription involves several key steps that transform single-stranded RNA into double-stranded cDNA. The initial step involves the binding of the enzyme reverse transcriptase to the RNA template. This enzyme is paramount, as its catalytic activity synthesizes the complementary DNA strand.
The mechanism can be outlined as follows:
- Template Hybridization: The RNA molecule hybridizes with a primer, which is a short segment of DNA or RNA that provides a free 3' hydroxyl group. This is crucial for initiating synthesis.
- Synthesis of cDNA: The reverse transcriptase enzyme facilitates the elongation of the DNA strand by adding nucleotides complementary to the RNA template. This process is guided by the base-pairing rules, allowing for accurate transcription.
- Formation of Double-Stranded cDNA: After synthesizing the first strand of cDNA, the RNA strand is removed, often via hydrolysis or specific enzymes. The second strand is then synthesized, yielding a double-stranded cDNA product.
This entire process is highly regulated and can be influenced by various factors such as temperature, pH, and the presence of inhibitors or co-factors. Recognizing these nuances is essential for optimizing results during experiments.
Key Enzymes Involved
The reverse transcription process relies on several enzymes, with reverse transcriptase leading the pack. Different types of reverse transcriptases exist, each with unique properties and advantages:
- M-MLV Reverse Transcriptase: This enzyme is derived from the Moloney Murine Leukemia Virus and is known for its high fidelity in synthesizing cDNA.
- AMV Reverse Transcriptase: Found in the Avian Myeloblastosis Virus, AMV reverse transcriptase functions effectively at higher temperatures, making it suitable for specific applications.
- Taq Polymerase: Often utilized in PCR, Taq can also act as a reverse transcriptase under certain conditions, highlighting its versatility in molecular applications.
In addition to reverse transcriptases, other enzymes such as RNase H play a role in degrading the RNA strand post-cDNA synthesis. Quality and reliability of the final cDNA product depend significantly on the choice of enzymes and their conditions of operation.
"Understanding the mechanics and enzymes involved in reverse transcription provides valuable insights for optimizing cDNA synthesis and ensuring accuracy in genetic analyses."
The fundamentals of reverse transcription are not just academic; they are the foundation upon which many modern biotechnological innovations are built. As science continues to evolve, so too will the methods and applications of reverse transcription, reinforcing its essential role in the realm of molecular biology.
Methodologies for cDNA Synthesis
Understanding the methodologies for cDNA synthesis is crucial for researchers. This process is central to many applications in molecular biology, including gene expression analysis and diagnostics. Each methodological element significantly impacts the efficiency, yield, and quality of cDNA produced. Selecting the right procedures and techniques can enhance experimental outcomes and lead to more accurate and reliable results.
Polymerase Selection
Choosing the correct polymerase for cDNA synthesis is vital. Different types of reverse transcriptases offer unique properties, making them suitable for various applications. For example, M-MLV (Moloney Murine Leukemia Virus) reverse transcriptase is known for its high processivity and ability to synthesize long cDNA strands. On the other hand, AMV (Avian Myeloblastosis Virus) offers a broader temperature range, which can be beneficial in specific experimental conditions.
When selecting a polymerase, consider factors like:
- Sensitivity: Some polymerases work better with low amounts of starting RNA.
- Specificity: Ensure that the chosen enzyme can effectively convert RNA to cDNA without significant errors.
- Yield: Evaluate the enzyme’s performance in producing high amounts of cDNA.
Overall, the choice of polymerase should align with the goals of your research, considering both the nature of the RNA sample and the downstream applications.
Optimizing Reaction Conditions
Optimizing reaction conditions is another key component of cDNA synthesis. This not only includes temperature but also factors such as pH and ionic strength. Successful optimization can enhance the reaction’s efficiency and the integrity of the synthesized cDNA.
Key considerations involve:
- Temperature: Reverse transcription typically occurs at around 37°C to 55°C. Adjusting this can affect enzyme activity and yield.
- Buffer Composition: A suitable buffer improves enzyme stability and function. Using commercial kits can simplify this aspect.
- Reaction Time: Ensuring adequate time for reverse transcription is crucial. Under/over-reacting can lead to suboptimal results.
These optimizations require experimentation and careful monitoring to determine the best conditions for specific RNA samples.
Priming Strategies
Priming strategies are essential for initiating reverse transcription. The choice of primers impacts the specificity and yield of the cDNA. Generally, there are three common approaches: oligo(dT) primers, random hexamers, and gene-specific primers.
- Oligo(dT) Primers: These target the poly(A) tail of mRNA, making them suitable for full-length cDNA synthesis of mRNA transcripts.
- Random Hexamers: Ideal for capturing a broader range of RNA types, including mRNA and non-coding RNAs.
- Gene-Specific Primers: Used for synthesizing cDNA from specific transcripts, allowing focused studies on particular genes.
Each priming strategy has implications regarding which RNA species are amplified and the completeness of the cDNA produced. The selection should be aligned with the biological questions being investigated.
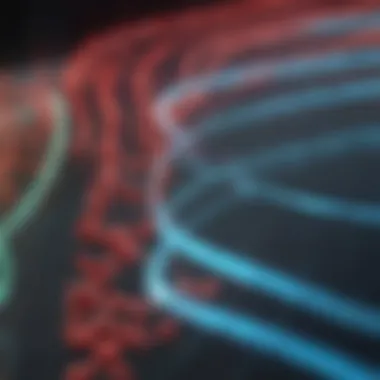
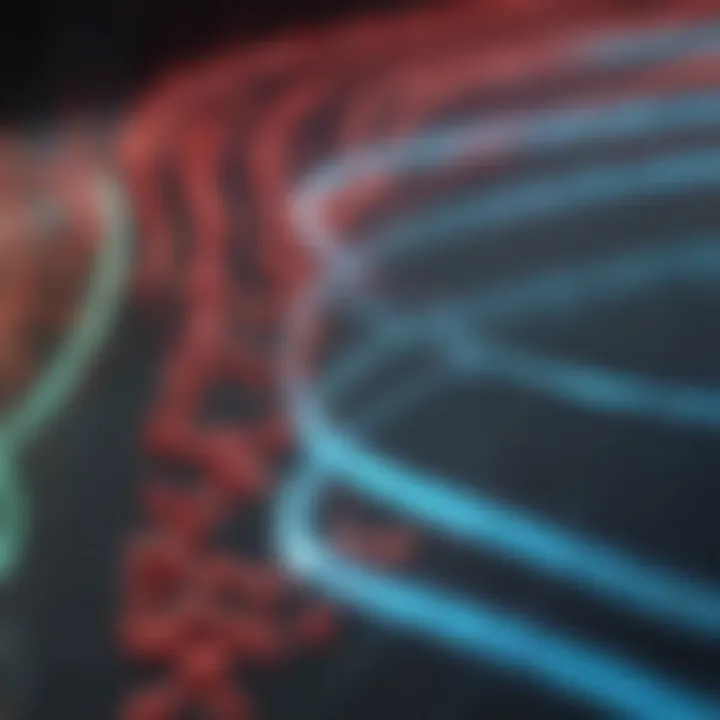
Applications of cDNA Reverse Transcription
cDNA reverse transcription serves as a cornerstone in modern molecular biology and various related scientific disciplines. Understanding its applications is vital because it offers insights into how researchers utilize this process to explore DNA, RNA, and their interactions. The significance of cDNA is not limited to mere synthesis; it extends into diverse fields like gene expression studies, diagnostics, and therapeutic development.
Gene Expression Analysis
One of the most prominent applications of cDNA reverse transcription is gene expression analysis. By assisting in the conversion of mRNA into cDNA, this technique allows scientists to examine gene transcription levels quantitatively. The ability to measure gene expression is essential for understanding cellular processes, disease mechanisms, and the impact of treatments.
In practical settings, real-time quantitative PCR (qPCR) often employs cDNA for the detection of specific genes. This method provides precise quantification, making it possible to compare expression levels across different conditions or treatments. Researchers can identify upregulated or downregulated genes in response to external factors, leading to significant insights into biological pathways and networks.
Diagnostic Applications
cDNA reverse transcription is also crucial in diagnostics. Particularly in molecular diagnostics, this method plays a critical role in detecting infectious agents and genetic disorders. Infectious diseases, such as viral infections, can be identified by converting viral RNA into cDNA, followed by amplification. This increases the sensitivity of detection and allows for timely diagnosis.
Furthermore, cDNA aids in the diagnosis of genetic disorders through techniques like RT-PCR. By analyzing specific gene expression patterns, healthcare professionals can identify mutations or alterations linked to diseases. This capability facilitates more accurate diagnoses and informs personalized treatment strategies.
Therapeutic Development
The therapeutic potential of cDNA reverse transcription cannot be overlooked. It has substantial implications in the development of gene therapies and mRNA-based vaccines. For instance, understanding how specific genes express can lead to developing novel therapies targeting aberrant gene products in various conditions, including cancer.
Additionally, the use of cDNA in vaccine development has gained prominence, particularly with the rise of mRNA vaccines. These vaccines rely on the principles of cDNA reverse transcription to generate mRNA, which then encodes antigen proteins. The immune response generated from such vaccines highlights the practical benefits stemming from the foundational knowledge within cDNA reverse transcription.
"The implications of cDNA reverse transcription reach far beyond the laboratory, influencing real-world applications in healthcare and research."
In summary, the applications of cDNA reverse transcription span across multiple domains, from gene expression analysis to diagnostics and therapeutic advancements. Understanding these applications not only illuminates the significance of cDNA but also represents a leap towards future innovations in biosciences.
Challenges in cDNA Reverse Transcription
cDNA reverse transcription is an essential part of many molecular biology workflows. However, it is not without its challenges. Understanding these challenges is crucial for researchers and professionals working in the field. Quality control issues and technical limitations are two significant factors that can affect the efficiency and accuracy of reverse transcription processes. By addressing these challenges, researchers can enhance the reliability of their experiments.
Quality Control Issues
Quality control is a paramount concern in cDNA reverse transcription. Impurities in RNA samples can lead to poor-quality cDNA, which can significantly impact subsequent analyses. If the starting RNA is degraded or contaminated, the resulting cDNA might not accurately represent the original transcriptomic landscape. This can lead to false conclusions in studies focusing on gene expression.
Inadequate measures during the cDNA synthesis can result in variations in cDNA yield and integrity. Therefore, it is crucial to implement robust quality control measures. This includes assessing RNA purity using spectrophotometry or gel electrophoresis before starting the reverse transcription. Ensuring that the RNA is intact and free of contaminants helps to improve the quality of the synthesized cDNA. Additionally, it is beneficial to use controls during the reverse transcription process to verify the efficiency and specificity of the enzymes used.
Using quality control steps at early stages saves time and resources later.
Technical Limitations
Technical limitations often arise during cDNA synthesis. One such limitation is the selection of the reverse transcriptase enzyme. Different enzymes exhibit varying efficiencies, substrate specificities, and operational temperature ranges. Some enzymes may be more suitable for specific RNA types. For instance, RNA with secondary structures may require enzymes with higher processivity.
Another technical aspect is the optimization of reaction conditions. Elements such as temperature, buffer composition, and ion concentration can significantly influence the success of reverse transcription. Suboptimal conditions might lead to incomplete reverse transcription or non-specific amplification. Thus, precise optimization is necessary for reliable results.
Moreover, the scaling of cDNA synthesis poses challenges, especially in high-throughput settings. When increasing the reaction volume, it becomes critical to maintain consistent enzyme activity and accuracy across larger sample sizes.
In summary, recognizing and addressing quality control issues and technical limitations are vital. These challenges necessitate careful planning and execution in the cDNA reverse transcription workflow to ensure the reliability and validity of the generated data.
Advancements in Reverse Transcription Techniques
The field of reverse transcription has seen significant advancements in recent years. These improvements enhance the efficiency, accuracy, and applicability of cDNA synthesis techniques. Understanding these advancements is crucial for researchers and professionals who wish to leverage these methods in various applications, including gene expression studies and therapeutic developments.
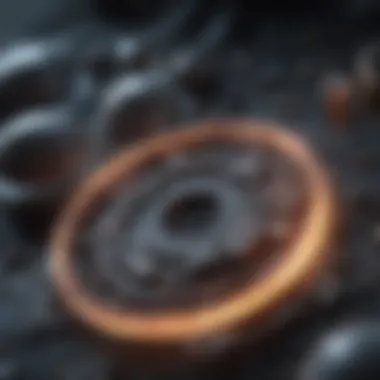
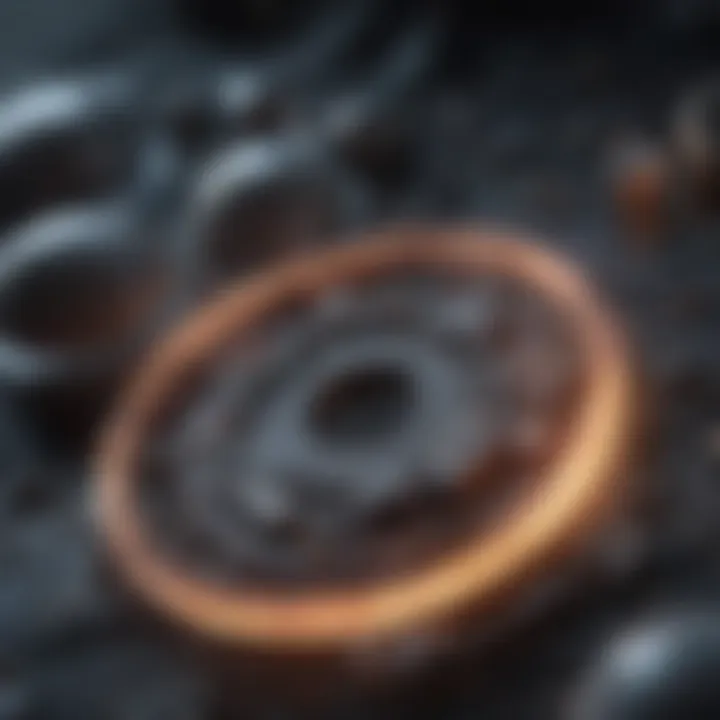
Novel Enzymatic Approaches
One of the most notable changes in reverse transcription techniques has been the development of novel enzymatic approaches. The traditional enzyme used for reverse transcription is Moloney murine leukemia virus reverse transcriptase (M-MLV RT). However, researchers have investigated alternative enzymes that display different properties. For instance, Thermus thermophilus reverse transcriptase (Tth RT) shows higher thermal stability and creates cDNA more rapidly under elevated temperatures. This can be beneficial in high-throughput experiments, where speed and efficiency are essential.
Additionally, engineered reverse transcriptases have emerged, showing increased accuracy in synthesizing cDNA. These engineered enzymes reduce errors during the reverse transcription process, leading to better-quality cDNA. This is particularly relevant when working with complex samples that may have low amounts of target RNA or when precision directly impacts the downstream applications such as real-time PCR.
Automation and High-Throughput Technologies
The integration of automation and high-throughput technologies represents another critical advancement in reverse transcription techniques. Automated systems reduce the hands-on time required for cDNA synthesis, allowing researchers to focus on analysis rather than manual processes. This is especially important in laboratories handling large sample sizes, where maintaining consistent conditions becomes challenging.
High-throughput techniques enable the simultaneous processing of multiple samples. Using microfluidics or plate-based systems, researchers can perform thousands of reactions in a short period, which accelerates research. As a result, projects can progress faster, and more data can be collected to draw meaningful conclusions.
In summary, advancements in enzymatic approaches and automation provide vital tools for modern biosciences, significantly improving the reliability and efficiency of cDNA synthesis.
Thus, embracing these advances can enhance the scope of research and diagnostics, making reverse transcription techniques more accessible and efficient for a wide array of applications.
Future Perspectives on cDNA Reverse Transcription
The field of cDNA reverse transcription is evolving rapidly, with significant implications for molecular biology and related disciplines. As researchers deepen their understanding of RNA dynamics and its relationship with gene expression, the need for refined reverse transcription methods becomes critical. This section addresses the significance of future perspectives by highlighting emerging trends and the potential integration of cDNA reverse transcription with other genomic technologies.
Emerging Research Trends
Current research trends emphasize improved sensitivity and specificity in detecting gene expression through cDNA techniques. Techniques like digital droplet PCR and single-cell RNA sequencing are becoming more prevalent. These approaches allow for a deeper insight into cellular behavior by capturing the transcriptome from individual cells. Emerging research is focused on enhacing reverse transcription efficiency while maintaining fidelity. Studies are exploring novel enzymes that could potentially replace traditional ones. Moreover, advancements in computational biology enhance the analysis of cDNA products, enabling more accurate interpretations of data.
"The integration of machine learning in genomic data analysis is paving the way for unprecedented precision in understanding gene expression patterns."
Researchers are also investigating the environmental impact on RNA stability, leading to optimized cDNA synthesis from various sources. This could open new opportunities for applications in environmental biology, such as assessing responses to pollutants. Additionally, the development of more robust priming strategies that can accommodate diverse RNA qualities will be crucial in future studies.
Integration with Other Genomic Technologies
The future of cDNA reverse transcription lies in its conjunction with other genomic technologies. Techniques like CRISPR-Cas9 for gene editing and RNA sequencing for transcriptome analysis show potential when combined with advanced reverse transcription methods. This integration can provide comprehensive insights into gene function and regulation. For instance, studies utilizing CRISPR-techniques alongside reverse transcription could lead to innovative ways to understand genetic disorders by reviewing how modifications in DNA might influence RNA expression.
Moreover, integrating cDNA techniques with high-throughput screening technologies could facilitate large-scale studies in drug discovery and personalized medicine. The capability to analyze extensive datasets would enable researchers to map the relationships between genetic variations and diseases more effectively.
Overall, the merging of cDNA reverse transcription with cutting-edge genomic techniques will likely revolutionize research methodologies and therapeutic strategies. Continuous exploration in these areas will enhance our capabilities to influence health outcomes and biological research.
End
The conclusion of this article underscores the significance of cDNA reverse transcription in modern molecular biology. It integrates the various aspects discussed throughout and highlights the critical role of cDNA in different applications. The importance of understanding cDNA reverse transcription cannot be overstated. It not only influences gene expression analysis but also opens pathways for diagnostic and therapeutic advancements.
As discussed, cDNA reverse transcription is foundational for converting RNA into a more stable DNA format. This transformation is essential for numerous applications in research and clinical practice. Understanding the nuances of this process allows researchers to optimize their methodologies, ensuring reliable results. The synthesis of cDNA enables the detailed study of gene expression, which is crucial for identifying biomarkers and developing targeted therapies.
Furthermore, the discussion has also touched upon emerging trends and advancements in reverse transcription techniques. These innovations not only enhance efficiency but also expand the potential applications of cDNA in biotechnology and clinical research. Addressing challenges associated with quality control and technical limitations remains vital for the future landscape of cDNA utilization.
"The progress in cDNA reverse transcription encapsulates a promising future for genomics and personalized medicine."
Thus, the implications of this topic reverberate across diverse domains in the biosciences. The need for continual exploration of cDNA reverse transcription techniques is paramount. This dedication will lead to enhancements in workflows, accuracy in experiments, and ultimately more significant biological insights.
Summary of Key Points
- cDNA reverse transcription is a crucial process in converting RNA to DNA.
- It serves as a gateway for gene expression studies and diagnostics.
- Implementing optimal methodologies is necessary for ensuring quality results.
- Ongoing advancements in techniques provide new opportunities for research.
- Challenges in the field require ongoing attention to maintain progress.
Call for Further Research
Future research is essential to deepen our understanding of cDNA reverse transcription. Here are some focal points for investigation:
- Developing more efficient reverse transcriptases to streamline cDNA synthesis.
- Exploring integration with next-generation sequencing technologies to enhance data acquisition.
- Investigating the impact of RNA quality on cDNA synthesis outcomes, potentially leading to better standardization.
- Assessing the implications of cDNA applications in the realm of personalized medicine, particularly in oncology and genetic disorders.
- Continuing examination of quality control measures to mitigate technical challenges during synthesis.
In summary, the journey of cDNA reverse transcription has just begun, and it invites ongoing exploration and innovation in future research initiatives.