Understanding Complementary DNA: An In-Depth Guide
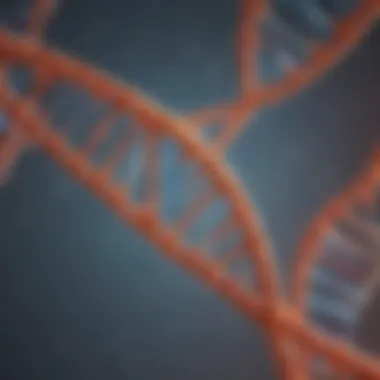
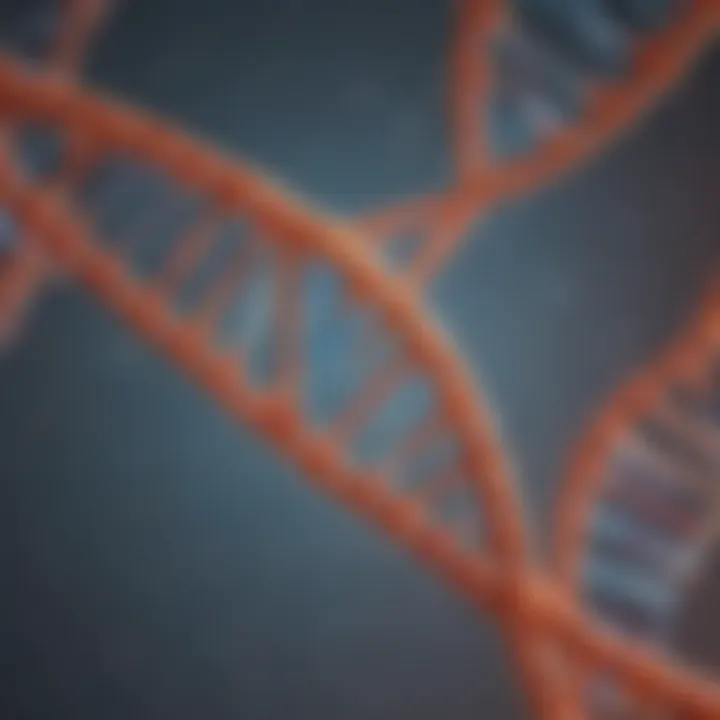
Intro
Complementary DNA, or cDNA, is increasingly vital in molecular biology. It serves as a critical tool for understanding gene expression and function. This narrative explores the synthesis, significance, and applications of cDNA in various research contexts. Understanding cDNA provides insight not only into fundamental biological processes but also into innovative technologies shaping the field.
Research Highlights
Key Findings
cDNA is synthesized through a reverse transcription process. This involves converting messenger RNA (mRNA) back into DNA with the help of the enzyme reverse transcriptase. The resulting cDNA is a complementary copy of the mRNA, allowing for the study of gene expression patterns.
The use of cDNA has facilitated numerous advances in genetic research. It plays a crucial role in quantitative PCR, enabling the quantification of gene expression levels. Additionally, cDNA libraries allow researchers to isolate and analyze specific genes, contributing to the understanding of various diseases.
Implications and Applications
Understanding cDNA has profound implications for genetic research, therapeutic developments, and biotechnology. It enables the identification of gene functions and interactions, facilitating advancements in personalized medicine. The technology can be used in vaccine development, cancer research, and stem cell studies, highlighting its versatility. Furthermore, emerging techniques like CRISPR gene editing and RNA sequencing are increasingly intertwined with cDNA processes.
Methodology Overview
Research Design
A robust methodology is necessary for effective cDNA analysis. Proper research design considers control measures, sample selection, and the specific techniques employed for synthesis and analysis.
Experimental Procedures
- Preparation of mRNA: First, mRNA is isolated from the cells or tissues of interest. This step ensures the integrity and purity of the RNA used during synthesis.
- Reverse Transcription: The isolated mRNA is subjected to reverse transcription. This involves mixing it with reverse transcriptase and other necessary reagents to generate cDNA.
- Amplification and Analysis: Once synthesized, cDNA can be amplified using polymerase chain reaction (PCR) techniques. This allows for further analysis and quantification of the gene expression levels.
cDNA synthesis is a foundational process, essential for various applications in molecular biology.
In summary, cDNA serves as a gateway to understanding the complexities of gene expression. The synthesis and study of cDNA enable researchers to explore the molecular bases of health and disease. As technologies evolve, the potential applications of cDNA are likely to expand, offering new avenues for scientific exploration.
Preface to Complementary DNA
In the realm of molecular biology, complementary DNA, commonly referred to as cDNA, holds a profound significance. Its understanding is crucial not only for basic research but also for practical applications in biotechnology and medicine. This section lays the groundwork for grasping the complexities of cDNA, exploring its definition, historical evolution, and its pivotal role in various biological processes. As we delve deeper into the intricacies of cDNA synthesis and its applications, it becomes evident that a solid understanding of its foundational aspects is imperative for students, researchers, and professionals.
Definition of Complementary DNA
Complementary DNA is synthesized from a messenger RNA (mRNA) template through a process known as reverse transcription. This synthetic form of DNA is the complement of the mRNA sequence. In simple terms, each nucleotide in the mRNA strand is paired with its corresponding nucleotide to create a cDNA strand. The process essentially transforms a single-stranded RNA into a double-stranded DNA, which can be subsequently used for various analytical purposes. cDNA does not include introns or non-coding regions, thus providing a distilled, functional representation of gene expression at a specific point in time.
Historical Context
The journey of cDNA began in the 1970s with the pioneering work of researchers like Howard Temin and David Baltimore, who independently discovered the enzyme reverse transcriptase. This enzyme is key to converting RNA back into DNA, laying the foundation for the molecular techniques we use today. Initially, cDNA was pivotal in studying retroviruses, but its applications have expanded vastly. Over the years, improvements in synthesis techniques and amplification methods have transformed cDNA into a cornerstone of genetic research, enabling the analysis of gene expression across various biological systems. Understanding this historical context provides insight into how cDNA evolved into a versatile tool in contemporary biology.
Importance in Molecular Biology
The importance of cDNA in molecular biology cannot be overstated. It serves as a critical intermediary in the expression of genes, allowing scientists to convert information from RNA into DNA, which is more stable for long-term studies. cDNA is instrumental in several key areas:
- Gene Expression Profiling: Researchers can quantify RNA transcript levels by creating cDNA libraries, which helps in identifying the active genes in specific tissues.
- Functional Gene Studies: cDNA enables the investigation of gene functions by facilitating cloning and characterization of expressed genes
- Disease Research: cDNA plays a crucial role in understanding genetic disorders by allowing scientists to analyze gene expression patterns under various pathological conditions.
"cDNA is more than just a copy; it’s a roadmap of gene expression that scientists can explore to unlock the mysteries of the genome."
In summary, cDNA is a fundamental component of molecular biology that bridges the gap between genotype and phenotype, providing invaluable insights into gene function and regulation.
The Mechanism of cDNA Synthesis
The mechanism of complementary DNA (cDNA) synthesis is integral to understanding its role in molecular biology. This process is vital for converting RNA sequences into stable DNA molecules. Such conversions allow researchers to study gene expression and function more effectively. By examining the mechanics of synthesis, one gains insight into both its advantages and potential limitations.
Reverse Transcription Process
The reverse transcription process is the first critical step in cDNA synthesis. This mechanism begins when an RNA template is used to create a complementary DNA strand. The enzyme reverse transcriptase catalyzes this reaction. The RNA strand binds to a short primer, which serves as a starting point for DNA synthesis. As the reverse transcriptase moves along the RNA, it synthesizes cDNA by adding complementary bases.
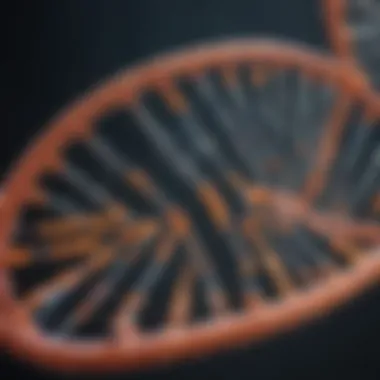
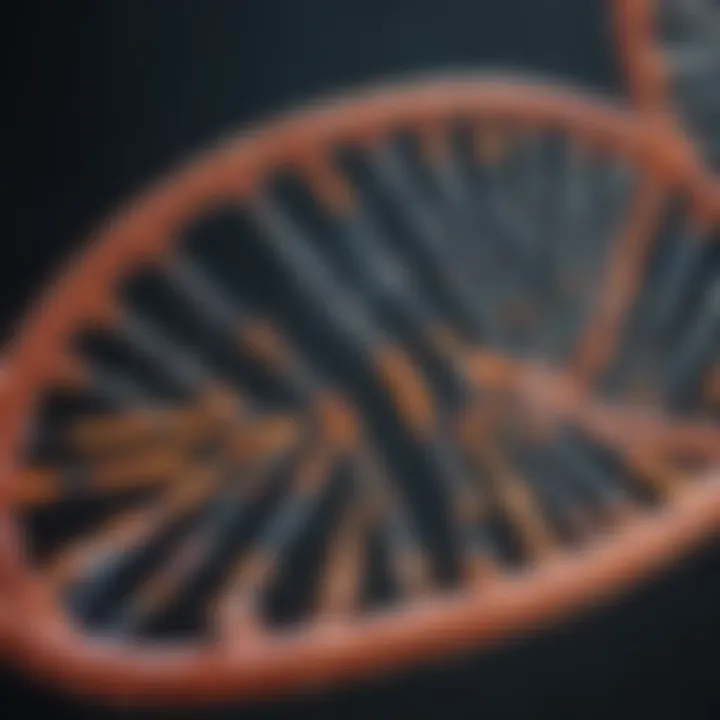
"The reliability of reverse transcription is vital for accurate cDNA generation."
This step is essential since the quality of the cDNA formed relies heavily on the fidelity of the reverse transcriptase. This process is not always 100% efficient, and factors such as the template length and RNA purity can affect results.
Enzymatic Role of Reverse Transcriptase
Reverse transcriptase plays a pivotal role in the cDNA synthesis mechanism. This enzyme has unique properties distinguishing it from other DNA polymerases. Its ability to utilize RNA as a template is crucial, as typical DNA polymerases cannot perform this function. Different reverse transcriptases exist, offering variations in thermal stability and processivity.
When synthesizing cDNA, the choice of reverse transcriptase can significantly impact the outcome. Commercial options include M-MLV and AMV reverse transcriptases, each with different characteristics.
Research applications often require high-quality cDNA, making the selection of the appropriate enzyme paramount. In addition, understanding the behavior of reverse transcriptase under varying conditions can lead to improved synthesis outcomes.
Polymerization of cDNA
After the initial synthesis through reverse transcription, the next phase is the polymerization of the cDNA strand. This step involves the elongation of the newly formed cDNA molecule. Following the completion of the first strand, a second strand can be synthesized, creating double-stranded cDNA. This can be done using DNA polymerase, which elongates the cDNA template to produce a complete molecule.
The advantage of double-stranded cDNA is its stability when stored, making it an ideal format for further analysis. Techniques like amplification and cloning become viable options following successful polymerization.
In summary, the mechanism of cDNA synthesis encompasses several critical steps, each contributing to the overall effectiveness of the process. An understanding of these elements can significantly enhance research capabilities and gene expression studies in diverse applications.
Applications of cDNA in Research
The applications of complementary DNA (cDNA) in research illustrate its pivotal role in advancing molecular biology and biotechnology. Understanding how cDNA is utilized can help in interpreting gene activity and function in various biological contexts. This section explores the key applications of cDNA, focusing on gene expression analysis, cDNA libraries, and functional gene studies.
Gene Expression Analysis
Gene expression analysis using cDNA is one of the most significant applications in the field of molecular biology. This method allows researchers to measure the expression levels of specific genes under various conditions. By converting mRNA into cDNA, scientists can create stable copies that enable easier quantification.
This process aids in distinguishing between actively expressed genes and those that are not, which is crucial when studying disease mechanisms, environmental responses, or developmental biology. Using techniques such as reverse transcription quantitative PCR (RT-qPCR), researchers can quantify cDNA and use it to infer gene expression levels.
cDNA Libraries
cDNA libraries represent another critical application of cDNA in research. By constructing a cDNA library, researchers can establish a collection of cDNA clones that correspond to mRNA present in a specific cell type or condition.
The cDNA library serves multiple purposes:
- Analysis of gene function
- Identification of novel genes
- Discovery of alternative splice variants
Researchers can screen these libraries for genes of interest, facilitating functional studies and drug discovery. The construction of a cDNA library typically involves the isolation of mRNA followed by synthesis of cDNA, which is then cloned into suitable vectors. This unique approach has paved the way for significant discoveries in genomics.
Functional Studies of Genes
Functional studies of genes are vital for validating the role of specific genes in biological processes. cDNA plays a central role here by enabling the expression of cloned genes in various systems. This can help in investigating the impact of individual genes on cellular function, signalling pathways, and phenotypic outcomes.
Key aspects of these functional studies include:
- Gene knockdown or overexpression: By manipulating the expression of cDNA, scientists can assess the resultant changes in cellular behaviour.
- Protein interaction studies: cDNA can be used to express proteins for interaction studies, clarifying the networks through which genes exert their effects.
As research continues to evolve, so does the application of cDNA in functional studies. Each investigation enhances the academic community's understanding of gene functions and their broader implications in health and disease.
"The proper application of cDNA can unlock new insights into biological complexity and functionality, greatly aiding our understanding of genetics and molecular interactions."
cDNA vs. Genomic DNA
The comparison between complementary DNA (cDNA) and genomic DNA is fundamental in molecular biology. Both types of DNA play distinct roles in genetic research and biotechnology. Understanding their differences helps clarify why scientists choose one over the other for various applications.
Differences in Structure and Function
cDNA is synthesized from an mRNA template through the reverse transcription process. Its primary role is to represent the expressed genes of the cell at a specific time. This is crucial for understanding gene expression patterns because cDNA reflects only the genes that are being actively transcribed. In contrast, genomic DNA contains all the genetic material of an organism, including non-coding regions and introns. It serves as a complete blueprint of an organism's genetic information.
The structure of cDNA is typically single-stranded and it may become double-stranded after synthesis. Genomic DNA, on the other hand, is double-stranded and organized into a complex structure of chromosomes. This difference is critical because it influences the methods applied during experimentation.
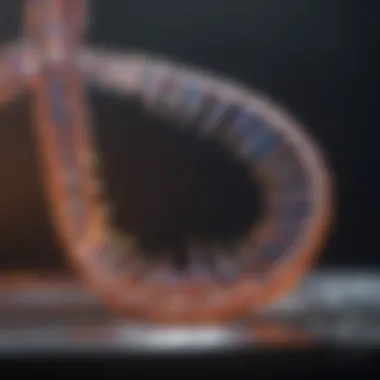
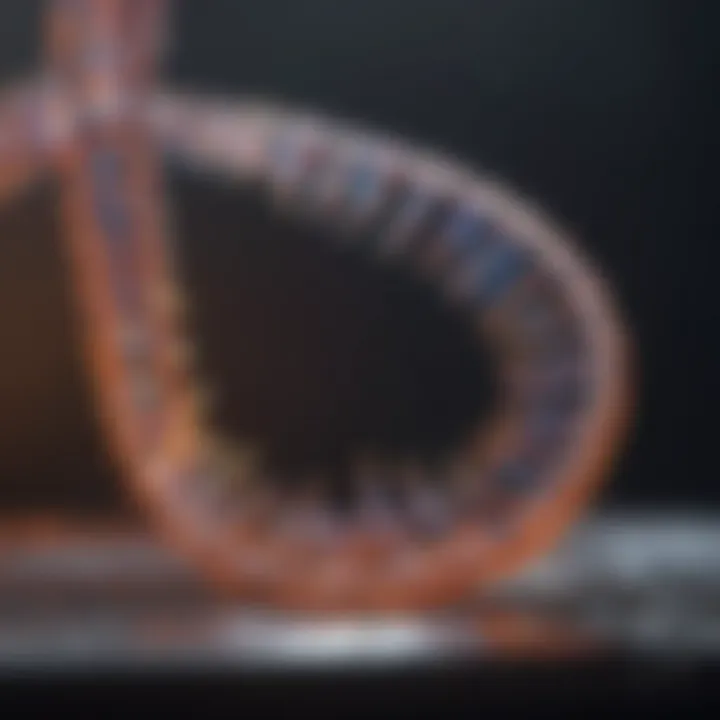
Quantification Techniques
When comparing cDNA and genomic DNA, quantification techniques vary significantly. For cDNA, methods like reverse transcription quantitative polymerase chain reaction (RT-qPCR) are commonly used. This technique enables precise measurement of specific mRNA levels, allowing researchers to gauge gene expression across various conditions. In contrast, genomic DNA quantification often employs standard quantitative PCR (qPCR) as well, but it focuses more on overall genomic content rather than specific gene expression.
In summary, the different characteristics of cDNA and genomic DNA shape their applications in research.
"Understanding these differences is essential for researchers, as it can influence the outcomes of genetic analyses and manipulations."
Using robust quantification techniques allows scientists to achieve accurate assessments, paving the way for advancements in genetic research and therapeutics.
Techniques Used in cDNA Research
Understanding the techniques used in cDNA research is vital for researchers and students alike in the field of molecular biology. These techniques not only facilitate the synthesis and analysis of cDNA but also unveil insights into gene expression and function. The advancements in these methodologies continue to expand the boundaries of what can be studied and understood. Here, we will explore three key techniques: Polymerase Chain Reaction (PCR), Quantitative PCR, and Microarray Analysis.
Polymerase Chain Reaction (PCR)
Polymerase Chain Reaction, commonly known as PCR, is a foundational technique in molecular biology. It allows scientists to amplify specific DNA sequences, making millions of copies from just a few initial template molecules. This amplification is important for various applications, particularly when the amount of starting material is limited.
The process involves repeated cycles of denaturation, annealing, and extension. During denaturation, the double-stranded DNA is heated to separate it into single strands. In the annealing step, short sequences called primers bind to targeted regions of the single-stranded DNA. Finally, in the extension phase, a DNA polymerase enzyme synthesizes new DNA strands.
This technique has several benefits:
- Sensitivity: PCR can detect low abundance sequences, enabling studies like gene expression analysis.
- Specificity: The use of primers ensures that only the desired targets are amplified.
- Speed: The entire process can be completed in a few hours, which is beneficial for time-sensitive experiments.
Quantitative PCR
Quantitative PCR (qPCR) extends the capabilities of traditional PCR by not only amplifying DNA but also allowing for the quantification of the target DNA in the sample. This is particularly useful for applications such as measuring gene expression levels.
In qPCR, fluorescent dyes or probes incorporated during the PCR process allow researchers to monitor the amplification in real-time. This provides data on the starting quantity of cDNA, which is essential for comparative analyses and standardizations.
Key advantages of qPCR include:
- Real-Time Data: Researchers obtain data during the amplification process, allowing for immediate analysis.
- High Throughput: Multiple samples can be analyzed at once, facilitating efficiency in experiments.
- Accuracy: The quantitative nature of this method enhances the reliability of data on gene expression levels.
Microarray Analysis
Microarray analysis represents another powerful technique in cDNA research, particularly in the exploration of gene expression profiling. This method involves hybridizing cDNA to a microarray chip, which contains thousands of probes corresponding to different genes.
When the cDNA binds to its corresponding probe on the chip, it produces a signal that indicates the presence of gene expression. This method allows for simultaneous analysis of the expression of thousands of genes, providing a comprehensive overview of cellular activity.
Some key aspects of microarray analysis are:
- High Throughput: Ability to analyze the expression levels of many genes at once.
- Comparative Studies: Researchers can compare gene expression profiles between different conditions or treatment groups.
- Data Richness: The wealth of data generated can lead to new discoveries in various biological processes.
"Each of these techniques shapes our understanding of cDNA and its applications, impacting research directions significantly."
In summary, effective techniques used in cDNA research, including Polymerase Chain Reaction, Quantitative PCR, and Microarray Analysis, enable a deeper understanding of genetic expression and molecular biology. By leveraging these methodologies, scientists are able to dissect complex biological systems and apply their findings to real-world challenges.
Challenges and Limitations of cDNA
Complementary DNA (cDNA) plays a crucial role in molecular biology, especially in gene expression studies. However, despite its significant advantages, there are considerable challenges and limitations associated with its use. Understanding these difficulties is important for researchers as it can influence experimental design and data interpretation. Awareness of these issues enables researchers to apply cDNA techniques more effectively and develop strategies to mitigate potential problems.
Technical Challenges
The synthesis and manipulation of cDNA involve various technical challenges that can affect the quality and reliability of results. One major issue is the quality of RNA used as a template. RNA is inherently unstable and can be degraded by RNases. This degradation can lead to incomplete or erroneous cDNA synthesis, impacting subsequent analyses. It is essential to use high-purity RNA and stringent handling protocols to preserve the integrity of the sample.
Another challenge lies in the efficiency of reverse transcription. The enzyme responsible for this process, reverse transcriptase, can be affected by various factors including temperature, buffer conditions, and enzyme quality. Variability in these conditions can lead to differences in cDNA yield and quality. Additionally, the possibility of secondary structures forming during the reverse transcription can hinder the enzyme's access to the RNA template, resulting in incomplete cDNA production.
Bias in Synthesis
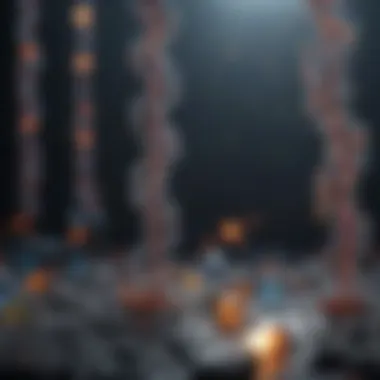
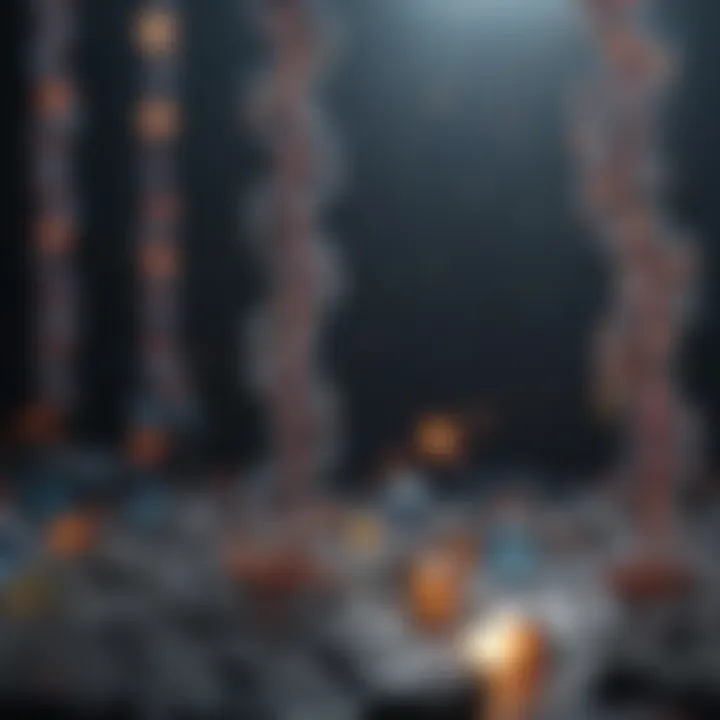
Bias during cDNA synthesis is another significant concern. This bias can arise from several factors, such as the oligo(dT) primers frequently used to initiate reverse transcription. These primers may not bind uniformly to all messenger RNAs (mRNAs), leading to unequal representation in the resulting cDNA library. Consequently, certain genes may be overrepresented while others are underrepresented, distorting the perception of gene expression levels.
Moreover, variations in the reverse transcription efficiency among different transcripts can exacerbate this bias. For instance, highly expressed genes are more likely to be converted into cDNA than lowly expressed genes, skewing data interpretation. Recognizing these biases is essential for researchers to select appropriate methods and perform accurate data normalization.
"Understanding the challenges associated with cDNA synthesis is vital to ensure the reliability of downstream applications."
Researchers should consider these limitations when designing experiments and interpreting results. Efforts to develop unbiased methods and improve reverse transcription efficiency may help overcome these obstacles. Innovations in cDNA research can lead to more accurate conclusions and a deeper understanding of gene expression dynamics in various biological contexts.
Emerging Technologies Involving cDNA
The field of complementary DNA (cDNA) is rapidly evolving, driven by technological advancements that enhance our capabilities in genetic research and biotechnology. These emerging technologies are crucial to understanding biological systems and developing innovative solutions for various challenges in healthcare and research. In this section, we will explore two key areas: RNA sequencing advances and single-cell cDNA applications.
RNA Sequencing Advances
RNA sequencing has revolutionized the way scientists analyze gene expression. This technology allows researchers to obtain precise readouts of the transcriptome, the complete set of RNA in a cell at a given time. One major advantage of RNA sequencing is its ability to detect low-abundance transcripts that traditional methods might miss. As a result, researchers can gain deeper insights into cellular functions and disease mechanisms.
Moreover, advancements in RNA sequencing technologies continually improve data accuracy and reduce costs. Several protocols have emerged that utilize cDNA synthesis prior to sequencing. This enables the conversion of RNA into cDNA libraries, which can then be sequenced. The increased efficiency of these processes accelerates the pace of research in areas such as cancer genomics, neuroscience, and developmental biology.
Integrating RNA sequencing with machine learning algorithms can also greatly enhance data interpretation. This marriage of techniques has the potential to identify gene interactions and regulatory networks that are essential for understanding complex biological phenomena.
"RNA sequencing stands at the forefront of genomic technology and cDNA plays a fundamental role in its effectiveness and scope."
Single-Cell cDNA Applications
Single-cell analysis has opened new doors in cellular biology by examining individual cells rather than bulk populations. This approach is particularly important when studying heterogeneous tissues, where differences among cells can be masked in aggregate data. The use of cDNA in single-cell applications is critical, as it allows for the detailed investigation of gene expression profiles at the single-cell level.
Recent advancements enable researchers to isolate cDNA from single cells, thus providing a clearer picture of the individual cellular state. Techniques such as single-cell RNA sequencing are now standard practices in biological research, allowing for the profiling of thousands of cells simultaneously.
The implications of these applications are profound. Understanding cellular diversity within tissues can unveil new targets for therapies, particularly in fields such as immunology and oncology. For instance, identifying unique expression profiles in tumor cells can lead to the development of personalized medicine approaches.
In summary, emerging technologies surrounding cDNA, like RNA sequencing and single-cell applications, are driving forward our comprehension of life's complexities. Together, they illustrate the interplay of innovation and knowledge in molecular biology and highlight the future potential of cDNA in research and development.
Future Directions in cDNA Research
The field of complementary DNA (cDNA) research is ever-evolving, driven by advances in technology and new understandings in molecular biology. As scientists continue to explore the complexities of gene expression and regulation, it becomes increasingly evident that cDNA will play a pivotal role in shaping future studies. In this section, we will examine the integration of cDNA with genomic studies and potential clinical applications, highlighting specific elements and considerations through which the field may develop.
Integrating cDNA with Genomic Studies
Integrating cDNA into genomic studies holds significant promise for enhancing our comprehension of genetic functions. This synthesis allows researchers to connect the expression patterns observed through cDNA analysis with genomic information. As techniques like whole-genome sequencing become more prevalent, pairing them with cDNA methodologies can elucidate the relationships between genotype and phenotype.
- Enhanced Understanding of Gene Regulation: By integrating cDNA with genomic data, scientists can better understand how certain genes are regulated at the transcriptional level. This can lead to identifying regulatory elements that influence gene expression.
- Elucidating Complex Traits: Combining cDNA analysis with genomic insights can aid in dissecting the genetic basis of complex diseases. Understanding how variant alleles affect gene expression can unravel pathways pertinent to diseases such as cancer and heart disease.
This approach encourages interdisciplinary collaboration and could lead to more refined models of how genes interact within biological systems. However, careful consideration must be given to data integration methods. The technical challenges in harmonizing these different data types are considerable but not insurmountable.
Potential Clinical Applications
The potential clinical applications of cDNA research are vast, significantly impacting diagnostics and therapeutic strategies. Here are a few areas where cDNA could revolutionize clinical practice:
- Personalized Medicine: The ability to analyze patient-specific gene expression profiles using cDNA can pave the way for tailored treatment plans. Understanding how a patient's genes interact with specific medications allows for customized therapy that could enhance efficacy and minimize adverse effects.
- Early Disease Detection: cDNA technology can improve early disease detection. By measuring specific RNA levels associated with diseases in patients, clinicians can identify conditions like cancer at an earlier stage, which often leads to better treatment outcomes.
- Vaccination Strategies: With insights from cDNA synthesis, researchers can develop RNA-based vaccines. These vaccines would instruct cells to produce specific antigens to stimulate an immune response. The rapid adaptation of this approach has been demonstrated during the COVID-19 pandemic.
"The integration of cDNA technologies into clinical applications could redefine treatment protocols and improve patient outcomes across numerous fields of medicine."
Epilogue
The conclusion of this article synthesizes the core concepts and discussions surrounding complementary DNA (cDNA). Understanding the importance of cDNA in molecular biology is crucial for both academic and practical applications. As we have explored, cDNA plays a key role in gene expression analysis, serving as a vital tool for researchers and practitioners alike. Its ability to convert RNA templates into a stable form allows for extensive studies on gene function and regulatory mechanisms.
Summary of Key Points
In this article, we covered several significant aspects of cDNA:
- Definition: cDNA is synthesized from an mRNA template via reverse transcription, providing essential insights into gene expression.
- Applications: Key applications include gene expression analysis, creating cDNA libraries, and facilitating functional studies of various genes.
- Distinctions: The fundamental differences between cDNA and genomic DNA were highlighted, emphasizing their unique roles in genetic research.
- Challenges: Technical challenges and potential biases in cDNA synthesis may affect research outcomes, underscoring the need for careful methodologies.
- Emerging Technologies: Advancements in RNA sequencing and single-cell applications expand the capabilities and significance of cDNA in contemporary research.
Implications for Future Research
The future directions in cDNA research promise exciting possibilities. Integrating cDNA studies with genomic research can lead to deeper understanding of the genome and its intricacies. As researchers continue to explore the clinical applications of cDNA, the potential to inform therapeutic strategies becomes more apparent. Furthermore, innovations in sequencing technologies may provide enhanced capabilities for studying gene expression at unprecedented resolutions. In summary, the progression of cDNA research is pivotal in unraveling the complexities of genetics and unlocking new avenues in molecular biology.
"The understanding of cDNA goes beyond mere applications; it represents a foundational element in the evolving landscape of genetic research."