Understanding Gene Expression: Mechanisms and Impacts
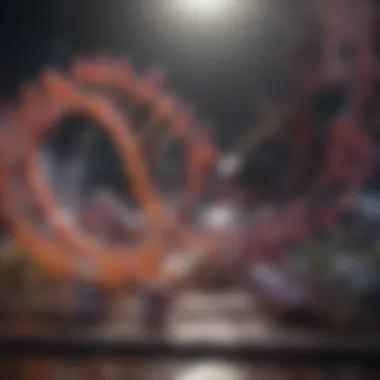
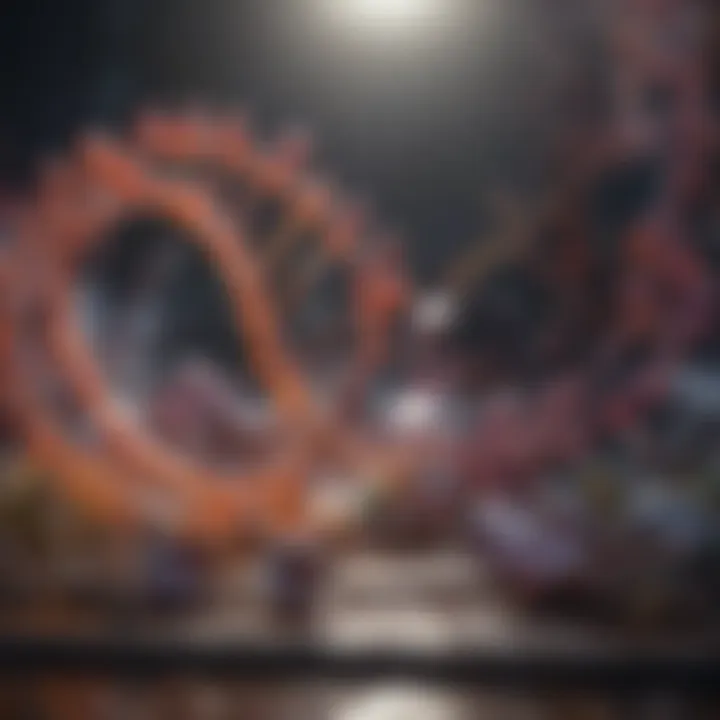
Intro
In the intricate web of biology, gene expression stands as a fundamental process, a bridge between the genetic blueprint within our DNA and the myriad functions that sustain life. It's not merely about deciphering sequences; it’s about understanding how these sequences dictate the very essence of who we are and how we respond to our environment. This article aims to shine a light on the complexities of gene expression, unraveling the layers from transcription to translation, while also touching upon the implications this knowledge has in areas such as health and biotechnology.
This exploration begins with the basics, providing an essential knowledge base for students, educators, researchers, and professionals alike. The nuances of how genes are turned on or off, the factors that influence this delicate balance, and the consequences of deviations from the norm are critical for anyone engaged in the life sciences. So, whether you're a seasoned researcher or just dipping your toes into the fascinating world of genetics, let’s begin a comprehensive journey into understanding this vital aspect of biology.
Research Highlights
In the field of gene expression, several key findings and their implications have emerged that merit closer examination. This section delves into the essence of these findings and how they shape current understanding and application.
Key Findings
- Transcription Factors: These proteins play a pivotal role in regulating gene expression by facilitating or hindering the transcription of DNA to mRNA, acting as gatekeepers in the genetic flow.
- Alternative Splicing: This process allows a single gene to produce multiple protein variants, enhancing the diversity of proteins produced in a cell without increasing the number of genes.
- Epigenetics: Modifications such as methylation and histone modification can influence gene expression without altering the DNA sequence itself, showcasing an additional layer of regulation.
- Small RNAs: Molecules like microRNAs have emerged as significant players in post-transcriptional regulation, impacting gene expression patterns in response to cellular signals.
Gene expression doesn’t merely reflect the genetic code; it is a dynamic process influenced by an interplay of cellular components and external stimuli.
Implications and Applications
Understanding gene expression has profound implications across various domains:
- Medical Research: Insights into gene regulatory mechanisms can lead to novel therapeutic approaches for diseases including cancer and genetic disorders.
- Biotechnology: In agricultural fields, manipulation of gene expression can result in crops with desirable traits, such as increased yield or resistance to pests.
- Pharmacogenomics: Tailoring medication based on individual genetic makeup is becoming feasible as knowledge of gene expression grows, paving the way for personalized medicine.
Methodology Overview
Understanding the methodologies employed to study gene expression can illuminate the approaches researchers take to unravel these complex processes.
Research Design
Studies often employ a combination of experimental and computational approaches to explore how genes are expressed under various conditions. This can include everything from lab-based experiments to bioinformatics analyses that predict gene behavior.
Experimental Procedures
Common techniques in this realm include:
- Quantitative PCR: Used to quantify mRNA levels, providing insights into how much of a gene is being expressed.
- RNA-Seq: This next-generation sequencing tool provides a comprehensive view of the gene expression landscape across the entire genome.
- Chromatin Immunoprecipitation (ChIP): This approach helps to identify the proteins that are bound to particular regions of DNA, shedding light on the mechanisms of gene regulation.
By delving into the methodologies used, we can better appreciate the rigor involved in gene expression research and the significance of the findings uncovered through these varied processes.
As this article continues, we will unravel the detailed mechanisms of transcription and translation, allowing for a deeper appreciation of how genes achieve their roles within the cellular architecture.
Defining Gene Expression
Gene expression stands at the crux of molecular biology, serving as a gateway to understanding the functional roles that genes play in living organisms. Grasping the nuances of this concept is essential, as it touches on various facets of cellular biology, genetics, and even medical science.
Overview of Genes
Genes are segments of DNA that act as the blueprints for building proteins and regulating various cellular processes. Picture them as instructions for assembling a complex piece of machinery. Each gene holds a specific code that guides the synthesis of proteins, which are pivotal in executing diverse functions related to growth, repair, and regulation within the body.
In essence, genes are vital to life; they carry the information that governs biological functions. The human genome, for instance, is made up of approximately 20,000-25,000 genes, each playing a unique role in determining everything from physical traits to susceptibility to diseases. More than just static sequences, genes interact with a variety of environmental and cellular signals. This dynamic relationship is where gene expression becomes vital – it's not just about having genes; it's about how they are activated or silenced in response to internal and external stimuli.
What is Gene Expression?
So, what exactly is gene expression? At its core, gene expression refers to the process by which the information encoded in a gene is used to synthesize a functional gene product, typically a protein. This involves two main phases: transcription and translation.
During transcription, the gene's DNA sequence is transcribed into messenger RNA (mRNA), which serves as a temporary copy of the genetic information. This mRNA strand then undergoes translation, where it is read by ribosomes to produce a corresponding protein. It's almost as if the gene has written a recipe, and now the cellular machinery is cooking up the end product.
Consider this analogy: if a gene is akin to a book containing a favorite recipe, gene expression is the act of using that book to create a delicious dish. Without the expression of that gene, no dish would ever be prepared. Consequently, understanding gene expression is fundamental for comprehending how organisms function, adapt, and respond to their environment.
"The expression of genes is the foundation of biological activity in cells and organisms."
In summary, defining gene expression encapsulates not just the technical processes involved but also its significance in the broader context of biology. It sheds light on how genetic information is utilized, how proteins are produced, and, ultimately, how life as we know it is sustained.
Through this exploration, we can appreciate the delicate orchestration of genes, proteins, and environmental factors that underpins life, laying a robust framework for the discussions to follow.
The Process of Gene Expression
The process of gene expression serves as a cornerstone in understanding how genetic information translates into action within living organisms. It's crucial to grasp this topic because it encapsulates how the subtle interplay of chemistry and biology may orchestrate a symphony of cellular functions. Without gene expression, our cells would operate like a poorly written script, devoid of meaning and functionality.
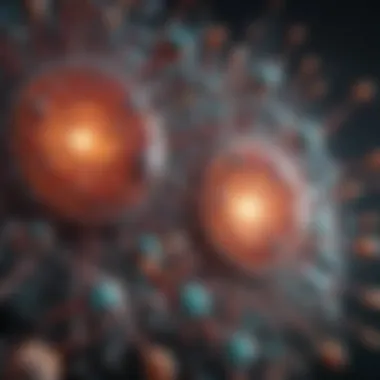
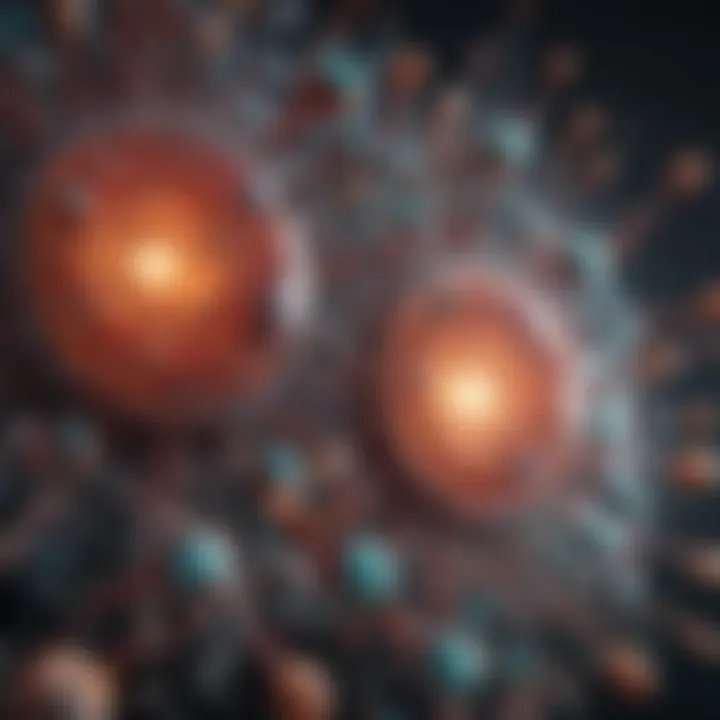
In this article, we’ll delve into the nitty-gritty details of how genes transform from mere sequences of nucleotides into proteins that perform critical tasks in our bodies. As we set the stage for exploration, it's going to become evident how essential the process is for numerous applications ranging from medicine to environmental science.
Transcription Explained
Transcription is the initial step in gene expression, where the information encoded in DNA is converted to messenger RNA (mRNA). This process can be likened to crafting a blueprint before constructing a building. Understanding transcription not only bolsters our grasp of biology but also sheds light on various implications in health and biotech.
Initiation
Initiation refers to that first critical moment when RNA polymerase binds to the DNA at a designated site known as the promoter. This stage is pivotal because it dictates which genes are activated at a particular time. A key characteristic of initiation is its precision; enzymes must recognize specific sequences to ensure that the right gene is expressed. For readers interested in molecular biology, this stage presents a profound benefit. It’s fascinating how tiny proteins can influence cellular fate merely by their ability to recognize a sequence!
A unique feature of initiation lies in its responsiveness to various signals. Factors like stress or development cues can control whether transcription takes place. However, this responsiveness brings with it a certain complexity; if the initiation fails or misregulates, it could lead to serious implications, even cancer.
Elongation
Once initiation has successfully occurred, elongation ensues. Here, RNA polymerase moves along the DNA, adding nucleotides to the growing mRNA strand. An essential aspect of elongation is that it signifies the actual synthesis of RNA, which allows for a more detailed analysis of transcription efficiency in diverse biological contexts.
Elongation is beneficial because it continues the process of gene expression, pushing the boundaries on types of RNA being synthesized, including mRNA, tRNA, and rRNA. What's especially unique about elongation is how it involves multiple elongation factors that assist RNA polymerase, ensuring the fidelity of transcription. Notably, errors during this phase can lead to nonfunctional proteins, demonstrating a potential downside.
Termination
Termination marks the end of the transcription process. It involves specific sequences that signal RNA polymerase to release the newly synthesized mRNA. This aspect is significant as it helps in ensuring that transcription does not continue indefinitely, which could lead to overproduction of genes.
A distinguishing trait of termination is its requirement for specific termination signals in the DNA sequence. For researchers, understanding this process is key, as improper termination can lead to truncated or malformed mRNA, severely affecting downstream protein synthesis. Additionally, studying termination can be beneficial for developing therapeutics that address transcription malfunctions.
Translation Mechanisms
After transcription, the next step is translation, where the mRNA synthesized during the previous process is decoded into a protein. This mechanism is fundamental in the world of biology because proteins are the workhorses of the cells, carrying out a multitude of roles essential for life.
Role of Ribosomes
Ribosomes play a pivotal role in the translation process. These cellular structures are the sites where the decoding of the mRNA into a protein takes place. A key characteristic of ribosomes is their ability to facilitate the interaction between mRNA and transfer RNA (tRNA). Their efficiency contributes to the speed and accuracy of protein synthesis, making them an invaluable part of cellular machinery.
Their unique feature lies in their dual composition of ribosomal RNA (rRNA) and proteins, showcasing a remarkable blend of genetics and chemistry. While they are undoubtedly efficient, ribosomes can also be influenced negatively by various inhibitors, which, if not properly managed, can lead to dysfunctional protein translation.
TRNA Functionality
Transfer RNA, or tRNA, has the unique job of bringing the correct amino acids to the ribosome during translation. This process creates polypeptides, the building blocks of proteins. A significant aspect of tRNA functionality is its specificity; each type of tRNA is charged with a particular amino acid determined by the codon on the mRNA.
One of the benefits of tRNA's intricate design is its ability to ensure that proteins are synthesized accurately according to the genetic code. However, a disadvantage might arise when tRNA is mischarged or fails to recognize the correct codon, leading to the potential obtention of flawed proteins. This goes to show that while tRNA is integral to proper protein synthesis, any missteps can have dire consequences.
Polypeptide Formation
Polypeptide formation is essentially the final act of translation, where sequences of amino acids are linked together to form a complete protein. Its contributing role in the overall topic is immense, as it embodies the end result of gene expression. One of its most striking characteristics is how these chains can fold into complex three-dimensional shapes that determine their function.
The unique feature here is the complexity of the folding process. It requires a collaborative effort from chaperone proteins to ensure the polypeptides fold correctly. However, pockets of errors can lead to misfolded proteins, potentially culminating in diseases such as Alzheimer's or cystic fibrosis.
"Gene expression processes are not just about passing on genetic instructions; they are about orchestrating cellular symphonies that maintain life's delicate balance."
Factors Influencing Gene Expression
Understanding gene expression goes beyond just its basic definition; it involves unraveling the various drivers that can influence how genes manifest themselves in living organisms. This section emphasizes the importance of recognizing these factors, as they underlie salient biological processes, with implications for health, disease, and biotechnology.
Genetic Regulation
Promoters and Enhancers
Promoters and enhancers are critical components in the realm of gene regulation. At the heart of this topic lies the promoter, a DNA sequence that initiates transcription of a particular gene. Promoters serve as docking sites for RNA polymerase, the enzyme that transcribes DNA into RNA. Enhancers, on the other hand, can be situated far from the gene they regulate but serve to amplify the transcription levels significantly.
One key characteristic of enhancers is their ability to boost transcription levels, making them invaluable for fine-tuning gene expression. Their versatility allows multiple regulatory proteins to bind, providing a complex and nuanced control over when and how much a gene is expressed. The unique feature here is that enhancers can work across considerable distances, influencing genes that are not even close in genetic sequence. However, this intricate relationship can also lead to potential misregulations, contributing to various diseases when things go awry.
Repressors and Activators
Opposite to enhancers, repressors and activators play pivotal roles in controlling gene expression in a manner that reflects the organism's needs. Activators are proteins that increase transcription, while repressors inhibit it. The delicate balance between these elements is what directs cellular functions effectively.
A hallmark of activators is their ability to engage with RNA polymerase, facilitating a swift and efficient transcription process. This characteristic makes them vital in scenarios where rapid gene expression is crucial to respond to environmental changes. On the contrasting side, repressors bring a sense of order, ensuring that unnecessary genes are not expressed, thus conserving energy and resources. However, the downside is that excessive repressive activity can stifle normal cellular processes, leading to health issues.
Environmental Influences
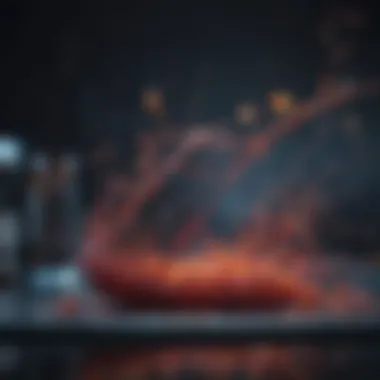
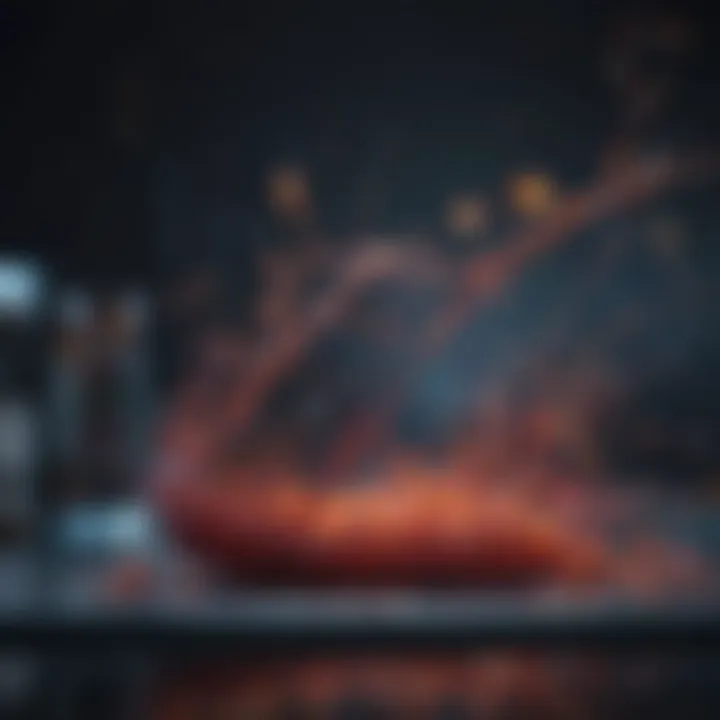
Temperature
Temperature serves as a significant environmental factor influencing gene expression. This aspect is particularly relevant when discussing organisms that thrive in varying climates. Changes in temperature can directly affect the activity of enzymes and proteins involved in transcription and translation, ultimately impacting gene expression levels.
One key characteristic of temperature influence is its ability to induce stress responses in organisms, leading to altered expression patterns. For instance, many cold-blooded animals will exhibit different gene expressions during warmer seasons compared to colder ones.
The unique feature here is that temperature not only modifies how proteins work but can also lead to adaptations over evolutionary timescales, offering organisms advantageous traits. However, abrupt temperature changes can also be detrimental, severely impacting survival rates.
Nutrient Availability
Nutrient availability is another major player in the world of gene expression. Cells often alter gene expression in response to the availability of essential nutrients, such as glucose and amino acids. This regulation ensures that cells use resources efficiently and survive in varying conditions.
A striking characteristic of nutrient availability is its role as a signaling mechanism; when nutrients are ample, genes responsible for growth and metabolism are upregulated, and conversely, during scarcity, genes linked to stress response and conservation are activated.
The unique aspect here is that nutrient availability governs not just the immediate responses but also long-term adaptations at the genetic level, such as metabolic reprogramming, enhancing resilience. The downside is that nutrient deprivation or imbalance can lead to detrimental effects on overall health and cellular function.
Stress Responses
Stress responses in organisms can have profound impacts on gene expression. Factors such as physical stress, oxidative stress, and biochemical stress provoke a myriad of changes within the cellular landscape, altering gene expression to manage and mitigate damage.
A key characteristic is how quickly cells can modulate gene expression in response to stress, an essential survival strategy. Some genes responsible for repair and defense mechanisms are activated, while others are downregulated.
The unique feature of stress responses lies in their complexity; they integrate signals from various stressors to orchestrate a concerted response at the transcriptional level. Despite its advantages, the downside is that chronic stress can lead to over-activation of certain genes, contributing to diseases such as cancer or autoimmune disorders.
Epigenetic Modifications
Methylation
Methylation is one of the most significant epigenetic modifications affecting gene expression. This process involves the addition of methyl groups to specific DNA segments, which can suppress gene activity. The typical role of methylation in gene silencing highlights its importance in regulating gene expression patterns across various developmental stages and cellular contexts.
A notable quality of methylation is its ability to offer stability; while DNA sequences might change, the methylation marks can preserve the expression profile, adapting without altering the underlying genetic information.
However, the unique aspect of this modification can also pose risks, as improper methylation patterns are linked to various disorders, including cancers.
Histone Modification
Histone modification is another key player in the epigenetic regulation of gene expression. Histones are proteins around which DNA is wrapped, and their modification can either promote or inhibit gene expression. Common modifications include acetylation and phosphorylation, which typically lead to a more open chromatin structure and increased gene activity.
A distinctive characteristic of histone modifications is their dynamic nature, enabling rapid alterations in gene activity in response to environmental stimuli. This ability makes histones flexible regulators of gene expression, allowing quick reactions to cellular demands. However, while beneficial for adaptability, irregularities in histone modifications can disrupt gene expression patterns, leading to serious implications for health.
In summary, understanding the myriad factors influencing gene expression equips researchers and practitioners with the insights necessary for powerful interventions in health, disease treatment, and biotechnological applications.
The Role of Gene Expression in Cellular Function
Gene expression holds a central position in the tapestry of cellular function, serving as the blueprint for how organisms develop and respond to their environment. At its core, gene expression involves the intricate orchestration of transcription and translation, culminating in the production of proteins that perform a myriad of functions essential for life. To fully grasp the significance of this process, one must explore two main areas: cell differentiation and homeostasis.
Cell Differentiation
Cell differentiation is a fascinating process where unspecialized cells transform into specialized cell types with distinct functions. It’s a complex dance that relies heavily on gene expression. Early in development, stem cells possess the potential to become any cell type. This versatility is harnessed through the selective expression of genes. For instance, a stem cell might express genes that lead to the formation of neurons, while another expression profile might push a cell to become a muscle cell.
- Key Factors in Cell Differentiation:
- Transcription Factors: These proteins bind to specific DNA sequences, activating or repressing target genes, thus influencing a cell’s fate.
- Epigenetic Modifications: Changes that do not alter the DNA sequence but can affect gene expression patterns, allowing cells to maintain their identity as they divide.
The consequences of gene expression in differentiation are profound. For instance, in the human body, the differentiation of hematopoietic stem cells into various blood cell types is meticulously regulated by specific gene activity. When this regulation goes awry, it can lead to disorders, including anemia and leukemia. Hence, understanding gene expression in cell differentiation not only sheds light on developmental biology but also on potential therapeutic strategies for various diseases.
Homeostasis and Regulation
Homeostasis refers to the process by which living organisms maintain a stable internal environment despite external changes. Gene expression is equally crucial for homeostasis, as it governs how cells respond to environmental stimuli. In response to fluctuating conditions such as nutrient availability or temperature shifts, cells engage specific genes to maintain their balance.
Consider the example of glucose regulation in humans. When blood sugar levels rise, insulin—a peptide hormone—is expressed. This activation leads to the uptake of glucose by cells, facilitating energy production and lowering blood sugar levels. Conversely, when glucose levels drop, glucagon is expressed to mobilize stored energy. This Push-Pull relationship showcases how gene expression is pivotal in regulating bodily functions to maintain homeostasis.
- In summary, several key aspects contribute to homeostasis:
- Feedback Mechanisms: Whether positive or negative, feedback loops rely on gene expression to stabilize physiological parameters.
- Signal Transduction Pathways: External signals are translated into cellular responses through gene activation or repression, enabling quick adaptability to changes.
By harnessing the power of gene expression, cells can fine-tune their responses, safeguarding the balance necessary for survival.
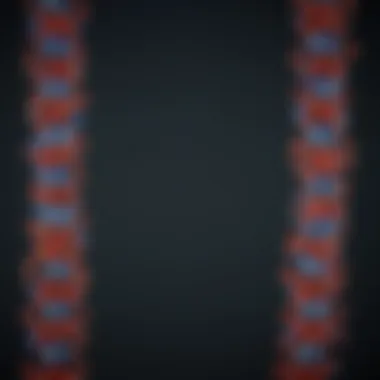
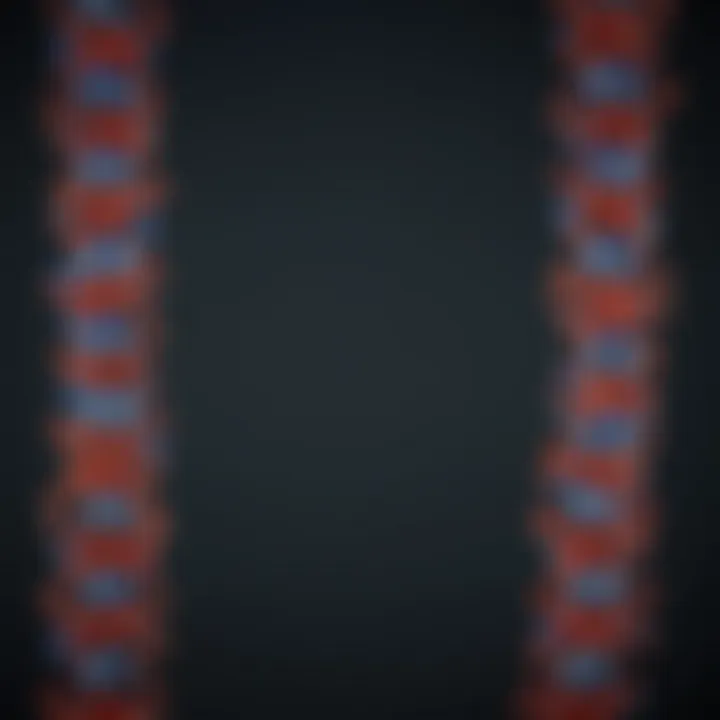
Gene expression intricately weaves through cell differentiation and homeostasis, signifying its paramount importance in the grand design of life.
Gene Expression in Health and Disease
Gene expression plays a pivotal role in understanding how our bodies function and malfunction. It is the process by which the information in a gene is translated into a physical product, often proteins, that are crucial for various biological processes. Disruptions in this pathway can lead to health issues ranging from minor ailments to severe diseases. This section delves into two critical aspects: oncogenes and tumor suppressor genes, and their relation to genetic disorders, shedding light on their significance in health and disease.
Oncogenes and Tumor Suppressor Genes
In the grand scheme of cellular mechanisms, oncogenes and tumor suppressor genes hold immense importance. Oncogenes are essentially mutated versions of normal genes that drive uncontrolled cell division. They act like a car accelerating down a highway without brakes. Their activity can lead to the formation of tumors. Common examples include the RAS gene and MYC gene, both notorious for their role in various cancers.
On the flip side, tumor suppressor genes, such as TP53, function as the body's natural defenses against tumor growth. These genes can halt the cycle of cell division or even initiate apoptosis, which is the process of programmed cell death. Think of them as the brakes in that car — when functioning properly, they help maintain normal cellular operation.
The balance between the activities of oncogenes and tumor suppressor genes is critical for cellular health. When oncogenes are overactive or tumor suppressor genes are defective, the risk of cancer escalates significantly.
"The intricate dance between oncogenes and tumor suppressor genes is paramount in dictating whether a cell remains healthy or slips into cancerous behavior."
Role in Genetic Disorders
Considering genetic disorders, the nuances of gene expression become even more significant. Genetic disorders often arise when a gene involved in critical processes malfunctions. For example, mutations in the CFTR gene lead to cystic fibrosis, emphasizing how a single gene hiccup can cause substantial health implications.
Moreover, gene expression can be influenced by epigenetic factors, which don't change the DNA sequence itself but affect how genes are turned on or off. This can explain why identical twins might not always share the same health outcomes, as environmental factors can modulate gene expression differently.
Here are some common examples of genetic disorders:
- Cystic Fibrosis: Caused by mutations in the CFTR gene leading to severe respiratory problems.
- Sickle Cell Disease: Hemoglobin structure is altered due to a mutation in the HBB gene, resulting in irregularly shaped red blood cells.
- Huntington’s Disease: A neurodegenerative disorder linked to expansions in the HTT gene, leading to motor dysfunction.
This interplay between gene expression and genetic disorders further underlines the necessity of understanding gene expression for both disease prevention and therapeutic strategies. Recognizing the implications of gene expression enables researchers and healthcare professionals to make informed decisions in treating or even preventing such conditions.
Biotechnological Applications of Gene Expression Research
The study of gene expression has birthed a multitude of biotechnological applications, lending itself to innovations that push the boundaries of medicine, agriculture, and industry. Understanding how genes are turned on or off can provide invaluable insights into improving human health, tackling genetic disorders, and enhancing crop yields. The significance of gene expression research cannot be understated; it serves as a foundation in creating cutting-edge solutions tailored to meet urgent global challenges.
Gene Therapy Techniques
Gene therapy is one of the most promising applications of gene expression research, aiming to treat or prevent diseases at their genetic root. In essence, this technique involves the alteration of gene expression by introducing new or modified genes into patient cells. This can be achieved through several approaches, such as:
- Direct Gene Replacement: In diseases caused by defective genes, replacing them with correctly functioning ones has shown potential. For instance, conditions like cystic fibrosis could theoretically be addressed by delivering a healthy version of the CFTR gene directly into lung cells.
- Gene Editing: Technologies like CRISPR-Cas9 allow for precise editing within the genome. This means that malignant mutations can be corrected, which can possibly lead to cures for genetic diseases, including sickle cell anemia and certain forms of muscular dystrophy.
- RNA Interference (RNAi): This method silences the expression of undesirable genes. By using small interfering RNA (siRNA), scientists can target specific mRNA molecules to inhibit their translation, proving beneficial in diseases such as cancer where overactive genes are at fault.
The successful implementation of gene therapy techniques hinges not only on understanding the mechanics of gene expression but also on addressing ethical concerns surrounding genetic manipulation. Limited trials are pivotal, and robust ethical guidelines must govern the exploration of such revolutionary treatment paths.
Biopharmaceutical Production
The influence of gene expression on biopharmaceutical production cannot be ignored, especially considering the increasing reliance on biological substances for medication. Gene expression plays a critical role in producing proteins, antibodies, and enzymes that serve therapeutic purposes. Here's how it unfolds in practice:
- Recombinant Protein Production: Many recombinant proteins, such as insulin and growth hormones, are synthesized in genetically modified organisms, like Escherichia coli or yeast species. By exploiting the mechanisms of gene expression in these microorganisms, scientists can produce large quantities of therapeutic proteins efficiently and cost-effectively.
- Monoclonal Antibody Generation: Through the manipulation of hybridoma technology, biopharmaceutical companies can create monoclonal antibodies used in treating conditions ranging from autoimmune diseases to cancer. By regulating gene expression, researchers can enhance the selection and production of desirable antibodies.
- Vaccine Development: Advances in gene expression research have paved the way for novel vaccine strategies. For example, mRNA vaccines, like those developed for COVID-19, depend on triggering an immune response by introducing mRNA that encodes a portion of the virus. This innovative application exemplifies how gene expression can lead to timely solutions during health crises.
"The potential of gene expression research is extraordinary, yet it is a double-edged sword. While it unlocks doors to miraculous cures, it demands a careful approach to its ethical and societal implications."
As science continues to delve deeper into gene expression, researchers and practitioners must remain vigilant about the potential risks and ethics surrounding these technologies. The promise of gene expression in biotechnology holds great potential, but navigating this landscape thoughtfully is key to its success.
Future Directions in Gene Expression Studies
The landscape of gene expression research is constantly evolving, presenting new avenues of inquiry that hold promise for profound advancements in biomedicine, agriculture, and environmental science. As we peel back the layers of molecular mechanisms, understanding how gene expression operates becomes foundational for addressing some of today's most pressing challenges, whether it’s developing targeted therapies or improving crop resilience.
In this exploration of future directions, we look closely at the significance of emerging technologies and the accompanying ethical considerations. These topics serve as critical bedrock for innovations that could reshape both our scientific endeavors and societal norms.
Emerging Technologies
The integration of cutting-edge technologies in gene expression studies holds immense potential for advancements. Among these, several standout innovations are worth highlighting:
- CRISPR-Cas9 Technology: This revolutionary gene-editing tool allows for precise alterations in the genome, making it possible to study gene function with unprecedented accuracy. Researchers are applying CRISPR to not only knock out genes but also to regulate their expression in real time, fostering a deeper understanding of genetic pathways.
- Single-Cell Sequencing: Unlike traditional methods, single-cell RNA sequencing enables scientists to analyze gene expression at the individual cell level. This high-resolution approach reveals heterogeneous cell populations and providing insights into how different cells within a single organism might express genes differently, permitting a more nuanced understanding of development and disease.
- Artificial Intelligence (AI) and Machine Learning: These technologies are increasingly used to analyze large datasets derived from gene expression experiments. By uncovering hidden patterns in gene activity, AI can aid in predicting how genes interact with one another and respond to various stimuli, accelerating hypothesis generation and validation.
Each of these technologies carries with it exceptional promise, yet they also provoke discussions about their use and the implications of manipulating life at such a fundamental level.
Ethical Considerations
As we forge ahead, the ethical dimensions of gene expression studies must be squarely on the table. The capabilities offered by emerging technologies prompt a re-evaluation of our moral compass regarding genetic manipulation. Considerations include:
- Safety and Efficacy: With techniques like CRISPR becoming commonplace, ensuring that gene editing can be done safely is paramount. Unintended consequences from off-target effects or unforeseen interactions in the genome must be thoroughly explored before widespread applications.
- Access and Equity: Who will benefit from advances? The disparity in access to gene therapies and manipulation technologies can exacerbate existing inequities. Ensuring fair access will be critical in the years ahead.
- Long-term Effects: As we edit genes, we must contemplate the implications for future generations. The possibility of heritable changes raises questions about consent and the potential to alter human evolution in unpredictable ways.
"As we stand on the threshold of genetic innovation, we must tread carefully, balancing the promises with the perils of our ventures into the genome."
Navigating these ethical waters is as essential to the future of gene expression research as the technologies themselves. Awareness and dialogue about these concerns will guide responsible research practices.
In summary, the future directions in gene expression studies are not just about enhancing scientific understanding; they involve deep ethical considerations. By embracing new technologies while maintaining a keen awareness of their societal implications, we can ensure that our research benefits humanity as a whole.