Understanding Infrared Imaging: Principles and Applications
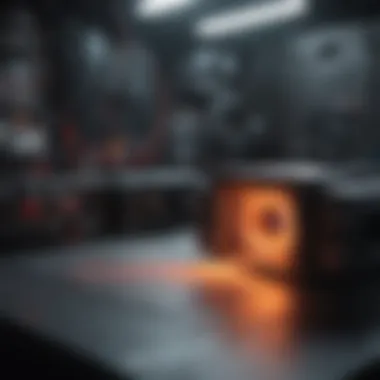
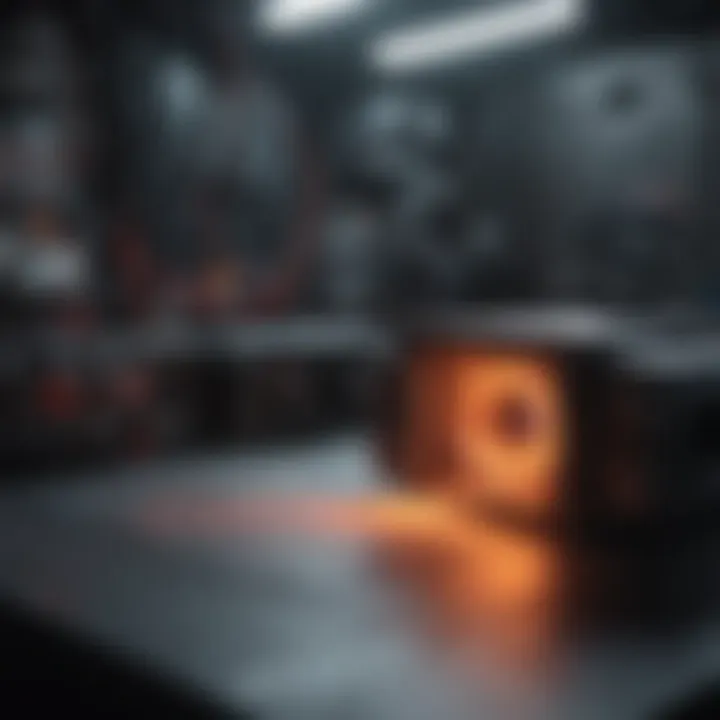
Intro
Infrared imaging is a technology that captures images using infrared radiation, which is emitted or reflected by objects based on their temperature. Unlike visible light imaging, this method allows for the detection of heat, revealing information that the human eye cannot perceive. This capability finds utility in numerous fields. The increasing sophistication of infrared sensors has expanded applications in biology, engineering, and environmental science. Understnading the foundational concepts of infrared imaging aids in comprehending its potential impacts.
Research Highlights
Key Findings
Infrared imaging operates on principles of thermal radiation. Objects that are warmer than their surroundings emit infrared radiation. Sensors convert this radiation into electronic signals, which then create images that represent temperature variations. Research has unveiled several important aspects in the field:
- Sensitivity: Modern sensors can detect minute temperature differences, improving precision in various applications.
- Real-Time Imaging: Infrared imaging enables the analysis of dynamic processes, offering insights as they occur.
- Non-Invasive Techniques: The method allows for monitoring without physical contact, crucial in medical and environmental applications.
Implications and Applications
The implications of infrared imaging extend across various disciplines:
- Biology: Monitoring animal behavior, detecting diseases in crops, and studying physiological responses.
- Physics: Analyzing thermal properties of materials and studying energy transfer.
- Environmental Science: Assessing heat patterns in ecosystems, aiding climate research, and monitoring habitat changes.
- Engineering: Quality control in manufacturing and thermal inspections in structures.
"Infrared imaging provides a lens into the unseen thermal world, enhancing both scientific understanding and practical applications."
Methodology Overview
Research Design
Understanding infrared imaging entails comprehending both the technology and its applications. The design of research focuses on the integration of advanced infrared sensors, their calibration, and data analysis techniques. Specific studies often employ a combination of field experiments and controlled laboratory conditions to validate findings across various environments.
Experimental Procedures
Experimental procedures typically follow these steps:
- Calibration of Sensors: Ensuring accuracy in measurements is crucial.
- Data Collection: Gathering infrared images under different conditions for comprehensive analysis.
- Image Processing: Using software to interpret thermal data and create visual representations.
- Analysis: Comparing results against known standards to assess performance in terms of precision and reliability.
Prelims to Infrared Imaging
Infrared imaging is a critical technology that finds applications across various fields, including biology, environmental science, and engineering. This section will clarify the significance of infrared imaging and its wide-ranging implications for research and practical applications. Understanding the underlying principles can enhance both academic and practical outcomes for students, researchers, educators, and professionals alike.
Definition and Importance
Infrared imaging is a technique that captures images based on infrared radiation emitted by objects. Unlike visible light, infrared radiation is not detectable by the human eye, yet it plays a vital role in assessing thermal characteristics of various materials and living entities. The importance of this technology derives from its ability to provide valuable data in conditions where traditional imaging fails.
Some benefits of infrared imaging include:
- Non-invasive Measurement: This technique can analyze subjects without requiring physical contact, which is essential in fields like medical diagnostics.
- Enhanced Visibility: Infrared imaging can provide thermal insights in low-light or obscured environments, useful for surveillance or nighttime operations.
- Diverse Applications: The technology is not restricted to one field; it is utilized in ecology, engineering, and beyond, demonstrating its versatility.
Historical Context
The foundation for infrared imaging began in the early 19th century with the discovery of infrared radiation by Sir William Herschel in 1800. His experimentation involved passing sunlight through a prism and measuring temperature variations in different colors of light. This initial exploration laid the groundwork for future advancements in infrared detection technology.
Through the 20th century, the development of infrared detectors and sensing devices advanced rapidly. Military applications spurred innovation in infrared technologies, particularly for night vision and target acquisition. As infrared imaging technology became more accessible, it transitioned into civilian applications, expanding into fields such as medicine, environmental monitoring, and industrial inspections. The historical context underscores the evolution of infrared imaging from a scientific curiosity to a crucial tool in various domains.
"Infrared imaging has transformed our approach to imaging in ways that were once unimaginable, offering insights that enhance understanding across disciplines."
Understanding these foundational elements in the historical development and importance of infrared imaging is essential as it sets the stage for exploring its fundamental principles and applications in the sections that follow.
Fundamental Principles of Infrared Imaging
Infrared imaging is a technology that fundamentally relies on the detection of infrared radiation. Understanding its principles is crucial for its effective application across various fields. This section explores the basic physics underlying infrared radiation, mechanisms for detection, and the role of infrared sensors and cameras, all of which contribute to the versatility of this imaging method.
Basic Physics of Infrared Radiation
Infrared radiation is a type of electromagnetic radiation with wavelengths longer than those of visible light, typically ranging from 700 nanometers to 1 millimeter. It is emitted by all objects based on their temperature. The amount of infrared radiation emitted increases with temperature, a relationship described by Planck's Law. Different materials absorb and emit infrared radiation differently based on their physical properties.
The detection of infrared radiation involves focusing on two critical concepts: spectral ranges and emissivity. Devices that implement infrared imaging are tuned to specific wavelength bands. Understanding the characteristics of these bands informs us how various materials will interact with infrared light. Higher emissivity materials tend to radiate heat more effectively than low emissivity materials. This inherent property is central to thermal imaging applications, allowing scientists and engineers to evaluate the thermal signatures of different objects.
Detection Mechanisms
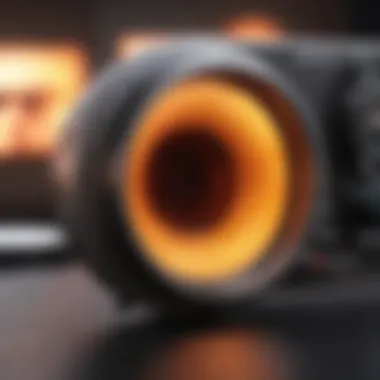
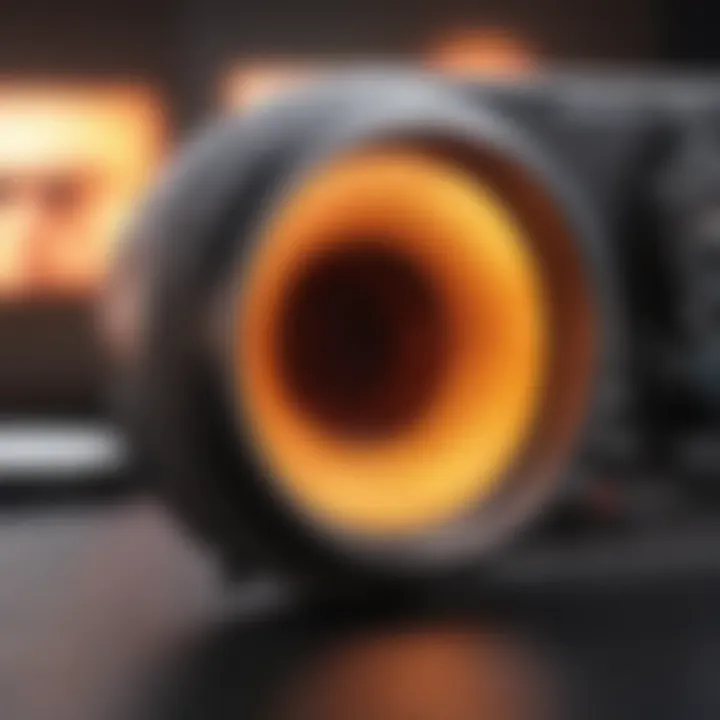
Detection mechanisms in infrared imaging rely on several technologies to interpret the infrared radiation emitted by objects. There are two primary methods: thermal detection and photon detection. Thermal detectors, like bolometers, convert heat energy into an electrical signal. They are sensitive to minute temperature changes, which makes them suitable for applications where subtle temperature variations are crucial.
On the other hand, photon detectors rely on the interaction of incoming infrared photons with specific materials. These detectors, often used in higher temperature applications or where greater sensitivity is necessary, convert light into an electrical response. Understanding these mechanisms helps researchers select the appropriate technology based on the particular requirements of their projects or examinations.
Infrared Sensors and Cameras
Infrared sensors and cameras are vital parts of infrared imaging systems. They convert infrared radiation into visible images or signals for analysis. The most common types of infrared sensors are uncooled microbolometers and cooled detectors. Uncooled microbolometers operate at ambient temperatures, making them suitable for compact, portable applications. They are widely used in handheld thermal imaging devices.
Cooled detectors, however, operate at cryogenic temperatures and offer heightened sensitivity and resolution, which is advantageous for scientific and military applications. The choice between these technologies depends on the specific needs, such as resolution, sensitivity, and operational environment.
Understanding infrared sensors and their operational principles plays an integral role in the advancement of infrared imaging nethods. They enhance the accuracy of detection, which in turn affects the outcome of various applications—from medical diagnostics to environmental monitoring.
"The advancements in detection mechanisms and sensor technology are crucial for the effectiveness of infrared imaging, enabling precise measurements across diverse applications."
Through these foundational elements, infrared imaging has been able to establish its significance in various domains, providing insights that would be difficult to achieve with visible light techniques alone.
Data Acquisition and Processing
Data acquisition and processing constitute critical elements in the realm of infrared imaging. These stages not only influence the quality of the resultant images but also determine their applicability across varied fields. Understanding these processes helps researchers and practitioners to optimize the performance of infrared sensors and cameras. Effective data handling is essential for producing reliable thermal images, where even minor discrepancies can lead to significant errors in interpretation.
Signal Processing Techniques
Signal processing techniques form the backbone of data analysis in infrared imaging. Raw data collected from infrared sensors are often incomplete or contain noise. This necessitates the application of various algorithms to enhance the image quality. Among the most common techniques are filtering, noise reduction, and image enhancement.
Filtering techniques, like Gaussian and median filtering, effectively smooth out the noise while preserving essential details. Noise reduction is vital in improving the contrast of the images, making them more interpretable. Image enhancement algorithms, including histogram equalization, can improve the visibility of crucial features in thermal images.
Effective signal processing can dramatically enhance the clarity and accuracy of infrared images, leading to more insightful analysis.
Several software solutions are available that allow researchers to apply these techniques efficiently. Tools like MATLAB and Python libraries have built-in functions for signal analysis, making the processing more accessible for users.
Image Calibration
Image calibration is another essential step in ensuring the accuracy of infrared imaging results. This process involves adjusting the raw output from sensors to account for various factors that may distort the thermal readings. Calibration corrects for sensor drift, temperature variations, and environmental influences, which can all affect image quality.
The calibration process typically includes the following steps:
- Dark current subtraction: This step removes any residual signal that is not due to the actual thermal emissions from the objects being measured.
- Non-uniformity correction: It adjusts for pixel-to-pixel sensitivity variations within the sensor array.
- Temperature scale adjustment: This ensures that the thermal readings are consistent with the real-world temperature measurements.
Proper calibration not only enhances the accuracy of thermal image readings but also fosters reproducibility in research settings. Many researchers rely on standard calibration methods outlined in literature or established protocols in specialized applications.
In summary, a thorough understanding of data acquisition and processing techniques in infrared imaging leads to more reliable, clear, and scientifically valuable thermal images.
Applications in Biology
The role of infrared imaging technology in biology is fundamentally transformative. It provides researchers with unique insights into biological processes that are often not observable through conventional methods. Its applications span across ecological studies and medical diagnostics, offering specific benefits that enhance our understanding and exploration in these fields.
Thermal Imaging in Ecology
Thermal imaging in ecology serves as a crucial tool for wildlife research. It allows for the non-invasive monitoring of animal behaviors and habitats without disturbing them. For instance, researchers can observe nocturnal animals that might otherwise remain hidden from sight. Different species emit varying amounts of infrared radiation, making it possible to identify and track individuals based on their thermal signatures.
Using infrared cameras, scientists study the effects of temperature fluctuations in wildlife populations, as temperature can greatly influence migration patterns and mating behaviors. This is particularly important for understanding how climate change impacts ecosystem dynamics.
Moreover, thermal imaging is beneficial for habitat assessments. It can provide data on vegetation health and density by detecting heat retention differences between healthy and stressed plants. Such detailed ecological monitoring aids in conservation efforts and helps devise strategies to protect endangered species.
Medical Diagnostics
In the realm of healthcare, medical diagnostics utilizing infrared imaging has progressed significantly. This technology provides a distinct approach to disease detection and patient monitoring. Infrared thermography can reveal abnormal temperature patterns associated with various medical conditions, including inflammation, infection, and malignancies.
For example, increased skin temperature can signify underlying issues such as infection. Physicians utilize infrared cameras to detect these anomalies, thus facilitating early intervention. This capability enhances diagnostic precision and potentially improves patient outcomes.
Another application in this domain is in breast cancer screening. Infrared imaging can serve as a supplementary tool alongside traditional mammography. By identifying abnormal heat patterns, it provides additional layers of information for radiologists.
Applications in Environmental Science
Infrared imaging technology plays a crucial role in the field of environmental science. Its ability to capture thermal radiation provides vital data that enhances our understanding of various ecological dynamics. The applications are diverse, ranging from climate monitoring to wildlife conservation. Each use of infrared imaging addresses specific environmental challenges, ultimately aiding in better decision-making and policy formulation. The incorporation of this technology leads to more informed conservation efforts while improving scientific research outcomes.
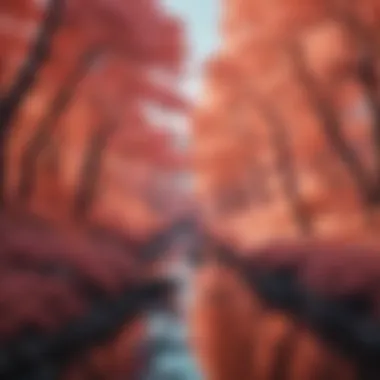
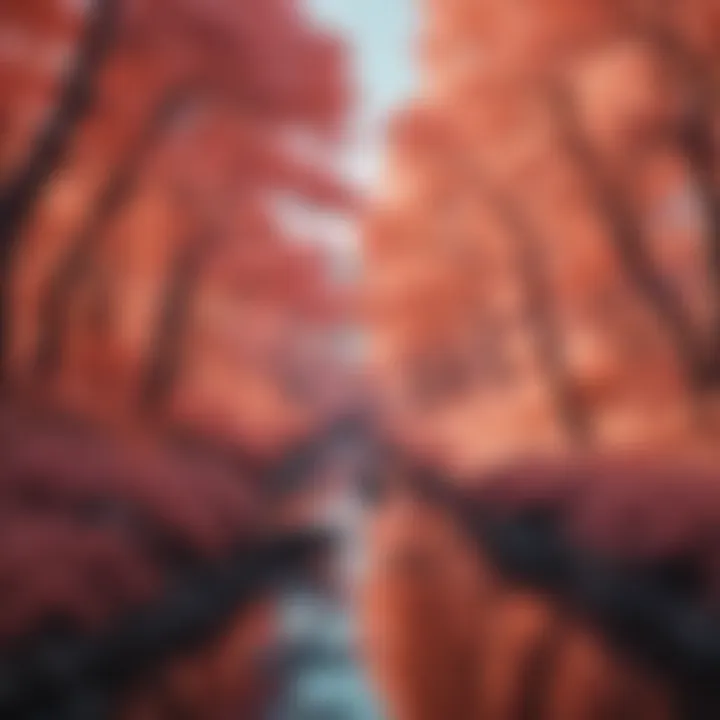
Climate Monitoring
Climate monitoring is an essential aspect of environmental science. Infrared imaging allows scientists to observe temperature variations across large areas. This ability to detect thermal patterns helps in understanding climate change and its impacts on different ecosystems. By analyzing infrared data, researchers can map out heat islands and track changes in vegetation over time.
The information gathered can be vital for numerous reasons:
- Monitoring Surface Temperatures: Infrared sensors provide detailed temperature profiles of urban areas versus rural landscapes, helping to identify the heat retention effects of urbanization.
- Understanding Ice Melt: Infrared imagery is instrumental in observing glacial and polar region changes, indicating climate change trajectory.
- Tracking Environmental Changes: This technology helps monitor shifts in ecosystems due to changing weather patterns.
One significant advantage of infrared imaging in climate monitoring is its capacity for large-area coverage. Satellite-based thermal imaging systems can assess climate-related variables from a distance, allowing for continuous monitoring without physical interference.
"Infrared imaging provides a non-invasive method for evaluating our changing climate, offering real-time data crucial for proactive responses."
Wildlife Conservation
Wildlife conservation efforts benefit immensely from infrared imaging technology. Infrared cameras enable researchers to monitor animal behaviors and populations in their natural habitats without disturbing them. This capability is essential for the conservation of endangered species and the management of protected areas.
Key applications in wildlife conservation include:
- Habitat Analysis: Understanding how animals inhabit and use various terrains aids in crafting effective conservation strategies. Infrared imagery reveals hidden patterns that are not visible in regular light.
- Population Monitoring: Tracking animal populations, especially elusive species, requires tools that do not impact their normal activities. Infrared cameras provide data without the need for human presence.
- Studying Behavioral Patterns: Researchers can observe how animals respond to environmental changes, helping to develop adaptive management plans to ensure their survival.
The use of infrared imaging in wildlife conservation raises important ethical considerations. It fosters a respectful approach to studying wildlife while minimizing human interference in their habitats. By integrating this technology, conservationists can devise more effective plans to protect biodiversity while ensuring sustainable interactions between humans and wildlife.
Applications in Engineering and Industry
Infrared imaging technology plays a pivotal role in engineering and industry. Its applications are vast, ranging from quality control in manufacturing to energy efficiency audits. By harnessing the unique properties of infrared radiation, professionals in various fields can achieve more accurate assessments and ensure optimal operational efficiency. This section will discuss two key applications of infrared imaging: non-destructive testing and energy auditing.
Non-Destructive Testing
Non-destructive testing (NDT) is a method used to evaluate materials and structures without causing damage. It is crucial in ensuring the safety and reliability of products in sectors like aerospace, construction, and manufacturing. Infrared imaging serves as a powerful tool in NDT by enabling the detection of deficiencies that may not be visible to the naked eye. This includes identifying heat stress, insulation faults, and structural weaknesses in components.
The advantages of using infrared imaging in NDT include:
- Speed and efficiency: Inspections can be conducted quickly, reducing downtime in production lines.
- Safety: NDT using infrared imaging minimizes the risk of exposing personnel to hazardous conditions.
- Detailed analysis: The technology provides precise thermal data that can be analyzed to predict maintenance needs.
Overall, infrared imaging enhances the reliability of engineering assessments, leading to safer and more effective designs.
Energy Auditing
Energy auditing is another critical application for infrared imaging in the engineering field. It involves an assessment of energy use in a building or facility to identify opportunities for improvement. Infrared cameras can detect heat loss, air leaks, and insulation defects, providing valuable insights into how energy efficiency can be enhanced.
The process typically involves:
- Thermal scanning: Inspecting walls, roofs, and various systems through thermal imaging.
- Identifying deficiencies: Pinpointing areas where energy is not being utilized efficiently.
- Recommendations: Offering targeted solutions based on the imaging results to improve energy consumption.
The benefits of energy auditing with infrared technology include:
- Cost Efficiency: By addressing energy losses, organizations can significantly reduce their operational costs.
- Environmental Impact: Enhanced energy efficiency leads to lower carbon emissions, contributing to sustainability efforts.
- Regulatory Compliance: Many industries are required to meet specific energy standards. Infrared imaging assists in meeting these mandates effectively.
"Energy audits utilizing infrared technology can transform operational efficiency and environmental responsibility in industries across the board."
In summary, infrared imaging technology offers significant advantages in both non-destructive testing and energy auditing. Its capacity to assess and enhance safety, efficiency, and sustainability makes it an invaluable asset in modern engineering practices.
Advantages of Infrared Imaging Technology
Infrared imaging technology stands out due to its distinct advantages across various fields. This section examines the core benefits that this technology offers, showcasing its relevance in both scientific and industrial applications. Key features of infrared imaging include its non-invasive nature and the ability to conduct real-time analysis. These benefits are fundamentally important as they promote efficacy and safety in diverse practices, ultimately leading to improved outcomes.
Non-Invasiveness
One of the most compelling advantages of infrared imaging is its non-invasive property. This characteristic is especially valuable in fields such as medicine and environmental monitoring. Unlike traditional imaging techniques, infrared imaging does not require direct contact with the object being analyzed.
For example, in medical diagnostics, it allows for the assessment of body temperature and blood flow without the need for invasive procedures. This non-invasive approach minimizes patient discomfort and significantly reduces the risk of complications.
In ecology and wildlife studies, infrared imaging enables researchers to observe animals in their natural habitat without disturbance. This leads to more accurate behavioral data and helps conserve species that may be sensitive to human interaction. The non-invasive nature fosters ethical practices in research and conservation efforts.
Real-Time Analysis
Infrared imaging also offers the capacity for real-time analysis, which is critical in many applications. This means that data can be collected, processed, and evaluated on-the-fly, yielding immediate insights.
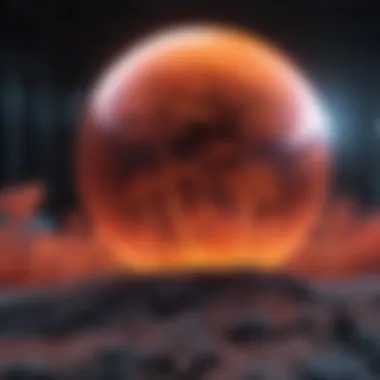
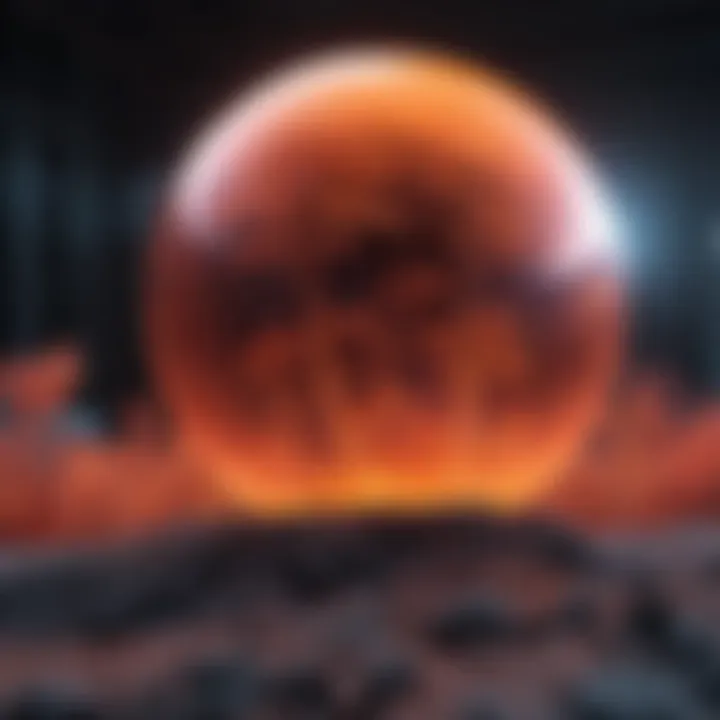
In industrial settings, the ability to monitor equipment temperature and identify hot spots can prevent equipment failure. For instance, in energy auditing, infrared cameras detect energy losses in buildings almost instantaneously. This quick feedback enables timely interventions, resulting in improved energy efficiency.
In the medical field, real-time monitoring of patients can aid in acute care situations, where every second counts. Health professionals can detect temperature abnormalities during fever monitoring or in assessing inflammation.
"Real-time analysis provided by infrared imaging is vital for prompt decision-making in critical situations."
The advantages of infrared imaging contribute significantly across various sectors. Its non-invasiveness and the capacity for real-time analysis allow for safer, more efficient operations. In the long run, leveraging these benefits can lead to enhanced research, conservation efforts, and improved industrial practices.
Challenges and Limitations
Understanding the challenges and limitations of infrared imaging is essential for anyone interested in its application across diverse fields. While infrared imaging offers numerous benefits, it is crucial to acknowledge the obstacles that may hinder its effectiveness. These challenges can affect the accuracy of the data collected and influence the decision-making processes in critical scenarios. Addressing these limitations requires a candid examination of the factors involved.
Environmental Interference
Environmental conditions can significantly impact the effectiveness of infrared imaging. Factors such as humidity, temperature fluctuations, and atmospheric conditions introduce variables that may distort the measurements collected. For instance, humidity can absorb infrared radiation, reducing the quality of the data captured. Similarly, temperature differences between the object being analyzed and its surroundings can lead to inaccurate readings.
This interference can complicate the interpretation of thermal images, making it essential for researchers and professionals to account for these variables when analyzing data. Employing advanced calibration methods and environmental sensors can help mitigate these effects to some extent. However, absolute accuracy can be elusive in uncontrolled settings.
Resolution and Sensitivity Issues
Resolution and sensitivity are critical components when discussing infrared imaging. High-resolution images are necessary for detailed analysis, especially in medical diagnostics or industrial inspections. Achieving high-resolution readings requires advanced sensors that can discern minute differences in temperature. Many standard infrared cameras may not provide the required sensitivity, leading to less precise results.
Sensitivity issues arise from the inherent limitations in sensor technology. Various factors, including the type of sensor, the spectral range, and the operating conditions, can affect performance. It is vital for researchers and industry professionals to select appropriate infrared cameras that match the specific requirements of their applications. This attention to detail can greatly enhance the accuracy of the infrared imaging processes.
"Just as understanding the limitations of a technology is crucial, the effective application hinges on the integration of advanced tools and methods to overcome hurdles in infrared imaging."
In sum, recognizing the challenges and limitations present in infrared imaging is vital for successful implementation in any field. Factors such as environmental interference and resolution restrictions can influence outcomes and need to be carefully considered in any analysis.
Recent Developments in Infrared Imaging
Recent advancements in infrared imaging have significantly transformed its applications and overall efficacy. These developments reflect ongoing research and innovation in sensor technology and data analysis. Recognizing these changes is essential for students, researchers, educators, and professionals who are looking to deepen their understanding of infrared imaging and its broader implications.
Advancements in Sensor Technology
The field of infrared sensor technology has witnessed remarkable progress over the last few years. New materials and designs have been crucial in enhancing sensor performance. For instance, quantum dot sensors have emerged, which provide higher sensitivity and resolution compared to traditional materials. This improvement is particularly beneficial in subtle temperature variations, allowing for more precise imaging. Additionally, the integration of microbolometers has made infrared imaging more accessible for various applications, from industrial inspections to medical diagnostics.
Another significant trend is the miniaturization of infrared sensors. Smaller, lighter sensors can be integrated into drones and handheld devices, expanding their usability in diverse environments. For example, drones equipped with advanced infrared cameras are increasingly utilized for ecological monitoring and search-and-rescue operations.
The development of multispectral and hyperspectral sensors is another milestone in infrared imaging. These technologies allow for capturing a wider range of wavelengths, enhancing data richness and improving analysis capabilities. As these sensors become more prevalent, they open up new avenues in fields such as agriculture, where they can monitor crop health with greater detail.
Integration with AI and Machine Learning
The integration of artificial intelligence (AI) and machine learning with infrared imaging technologies is arguably one of the most significant developments in this domain. By employing advanced algorithms, researchers can now analyze large sets of infrared data efficiently. This capability enhances image processing and can lead to more accurate interpretations of thermal patterns.
Machine learning models can be trained to identify anomalies or specific features in infrared images. In medical diagnostics, for example, these models can assist in detecting tumors or other health conditions by highlighting areas of concern in thermal images. Moreover, AI algorithms can improve the accuracy of predictions regarding how objects will behave under certain thermal conditions.
This synergy also promotes real-time analysis, enabling quicker decision-making in critical applications, such as disaster response and environmental monitoring. With continual improvements, the potential for AI to revolutionize infrared imaging is substantial, as systems become more intuitive and user-friendly.
"The ongoing advancements in infrared imaging technologies highlight not only the potential for improved applications but also the collaboration between engineering expertise and data sciences."
In summary, the recent developments in infrared imaging place a strong emphasis on both sensor technology advancements and AI integration. These innovations enable broader applications and enhance the precision of results across various fields, from biology to engineering. Professionals in these areas must remain informed about these shifts to leverage the technology effectively.
Future Prospects of Infrared Imaging
Understanding the future of infrared imaging is crucial for researchers, educators, and professionals across various fields. As technology evolves, infrared imaging continues to provide significant advancements and opportunities. This section delves into anticipated developments in this area, highlighting its potential to transform industries and enhance scientific research.
Expanding Applications
The scope of infrared imaging is broadening. Its applications are no longer limited to medical diagnostics or environmental science. Now, infrared imaging is being integrated into areas such as agriculture and security. In agriculture, farmers utilize this technology to monitor crop health, detect diseases, and optimize irrigation practices. Infrared imaging can help identify unhealthy plants before symptoms become visible.
In security, thermal cameras produce images based on heat signatures, providing crucial data for surveillance and monitoring. This capability greatly enhances the ability to detect unauthorized access or other threats, especially at night or in low-visibility conditions. Furthermore, industries like manufacturing and construction are starting to adopt infrared imaging for quality control, equipment maintenance, and energy efficiency audits. This wide array of applications signals a promising future for infrared technology.
Research Directions
As infrared imaging develops, research will play a pivotal role in enhancing its capabilities. Several key areas are emerging where researchers are focusing their efforts. One of them includes improving sensor technology. Researchers are exploring materials that can enhance sensitivity and resolution. This could lead to more accurate imaging in various conditions.
Another significant direction is the integration of infrared imaging with artificial intelligence and machine learning. With AI, infrared data can be analyzed rapidly, enabling real-time insights. This integration can improve medical diagnostics, automated security systems, and even predictive maintenance in engineering.
Investments in portable infrared imaging devices could also change the landscape. As devices become lighter and more accessible, widespread use in various fields will likely increase.
"The potential of infrared imaging technology is boundless. As we continue to explore its applications, we can expect transformative impacts across multiple sectors.”
Embracing these research avenues can lay the foundation for the future advancements of infrared imaging. Continuous innovation ensures that infrared technology remains relevant, effective, and indispensable in scientific and practical applications.