Understanding Laser Technology: Principles and Innovations
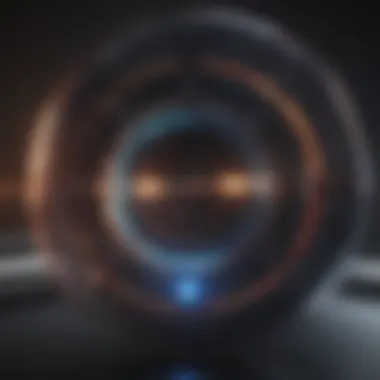
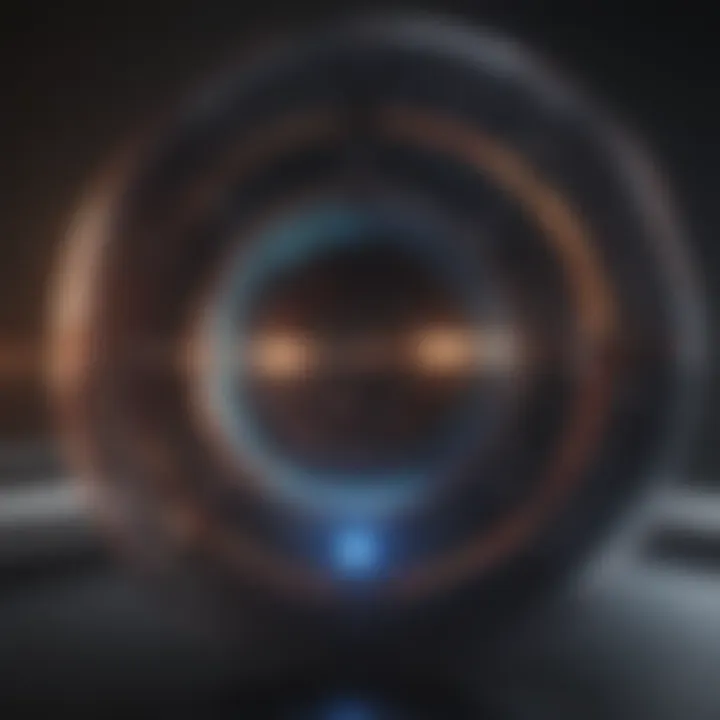
Intro
Laser technology stands as one of the most transformative advancements of the modern era. Since its inception, the principles governing lasers have been harnessed across various fields, making significant contributions to science, industry, and healthcare. This article seeks to unravel the intricate workings of laser technology, diving deep into the theoretical aspects that drive its operation. We will examine the fundamental physics behind laser emission, the components that enable the generation of coherent light, and the diverse applications that have emerged from this powerful technology.
From the medical arena, where lasers are employed in surgical procedures and imaging, to communication systems utilizing fiber optics, the versatility of lasers highlights their crucial role in contemporary advancements. As innovations continue to develop, understanding laser principles becomes increasingly important for students, researchers, educators, and professionals alike. Through this article, we aim to bridge complex scientific concepts with practical applications, enriching your knowledge of an essential technological phenomenon.
Prelims to Laser Technology
Laser technology is a pivotal topic in modern science and engineering. Its relevance extends beyond basic theoretical understanding; it integrates complex physics with practical applications that significantly enhance various fields. Exploring this topic is crucial because lasers have transformed how we approach processes in medicine, manufacturing, and communication.
Laser technology operates on the principle of light amplification through stimulated emission of radiation. This concept, once confined to theoretical discussions, has found profound applications, allowing for precise cutting, medical surgeries, and the development of secure communication technologies.
Understanding laser technology provides numerous benefits. It equips researchers and professionals with the necessary knowledge to innovate and implement laser solutions effectively. Additionally, being familiar with the operational principles and components encourages effective decision-making in selecting appropriate laser systems for specific applications.
There are several considerations when delving into this topic. One must grasp the foundational elements, such as the physics behind laser operation, and the impact these have on modern applications. The implications of emerging technologies also warrant attention as the field progresses at a rapid pace.
In summary, laser technology represents a confluence of theoretical understanding and practical application, making it crucial for students, researchers, and professionals.
Defining Laser
A laser is a device that produces light through a process known as stimulated emission. The term 'laser' itself is an acronym for Light Amplification by Stimulated Emission of Radiation. Lasers can emit light in a narrow beam that is both coherent and monochromatic, meaning the light waves are in phase and have a single wavelength. This unique quality differentiates lasers from traditional light sources, making them highly useful for a range of applications, from barcode scanners to precision medical instruments.
Historical Overview
The history of laser technology is marked by significant milestones that reflect advancements in scientific understanding and technological innovation. It is essential to explore these developments to appreciate how far laser technology has come and where it is headed.
Early Experiments
In the mid-20th century, scientists started experimenting with the concept of light amplification. These early experiments involved exploring the interactions between photons and materials, laying the groundwork for later advancements. The key characteristic of these experiments was the focus on light's behavior under various conditions. This focus is beneficial for understanding how lasers function today.
Unique features of these early experiments included the exploration of gas and solid-state mediums for light emission. The primary advantage of these early experiments was the idea that light could be stimulated to produce coherent outputs, a concept that would eventually lead to the creation of the laser. However, the technology was largely theoretical and not yet practical.
The Birth of the Laser
The conceptual leap that led to the first operational laser occurred in 1960 when Theodore Maiman successfully created the first working laser using a ruby crystal. This marked the birth of the laser as we know it today. This critical development highlighted the importance of gain mediums, which are essential for the operation of lasers. This invention opened new doors in technology and set the stage for various applications in daily life and industry.
The unique feature of the first laser was its use of a solid-state medium, contrasting sharply with the gas lasers that were being explored concurrently. The advantage of Maiman's ruby laser was its ability to produce intense, focused light in a coherent beam. This breakthrough led to a rapid expansion of laser technology into various sectors, marking a significant point in the evolution of laser applications.
Theoretical Foundations of Lasers
The theoretical foundations of lasers are essential in comprehending how laser technology operates. This section aims to discuss the principles that govern the physics of lasers. Understanding these foundations allows for a clearer insight into their practical applications and innovations in the field. In laser technology, key elements such as light behavior, stimulated emission, coherence, and population inversion play significant roles in the functionality and efficiency of lasers.
Physics of Light
Light is an electromagnetic wave. Its properties, including speed, frequency, and wavelength, are crucial for laser operation. The behavior of light in terms of reflection, refraction, and diffraction is important when designing laser systems. The interactions of light with materials enable various applications, including industrial cutting, medical surgery, and telecommunications.
Stimulated Emission and Amplification
Overview of the Process
Stimulated emission is the process by which an incoming photon stimulates an excited electron in a gain medium to drop to a lower energy state. This action leads to the emission of a second photon. Both photons are coherent, meaning they have the same phase and direction. This characteristic is vital because it ensures that the light produced by lasers is highly collimated and intense. This makes stimulated emission a popular choice in laser technology for generating amplified light.
Energy Level Diagrams
Energy level diagrams visually represent the electronic states of atoms or molecules. They show how electrons transition between different energy states during stimulated emission. These diagrams help to illustrate the amount of energy needed for an electron to move from its ground state to an excited state. Such representations are helpful in understanding the design and function of various lasers, especially concerning their efficiency and output characteristics.
Coherence and Monochromaticity
Coherence refers to the phase relationship between different waves of light. It is a necessary quality of laser light, allowing multiple laser beams to overlap without losing their original properties. Monochromaticity indicates that laser light consists of a single wavelength, contributing to its precision and clarity. These two properties are what distinguish laser light from regular light sources, making it incredibly useful in applications requiring high accuracy.
Population Inversion
Mechanisms Behind Population Inversion
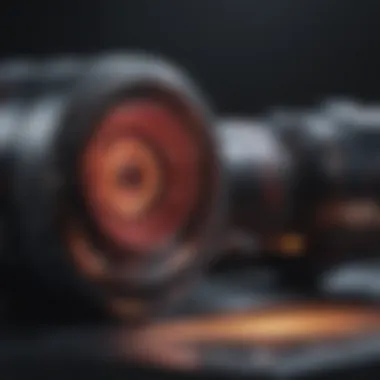
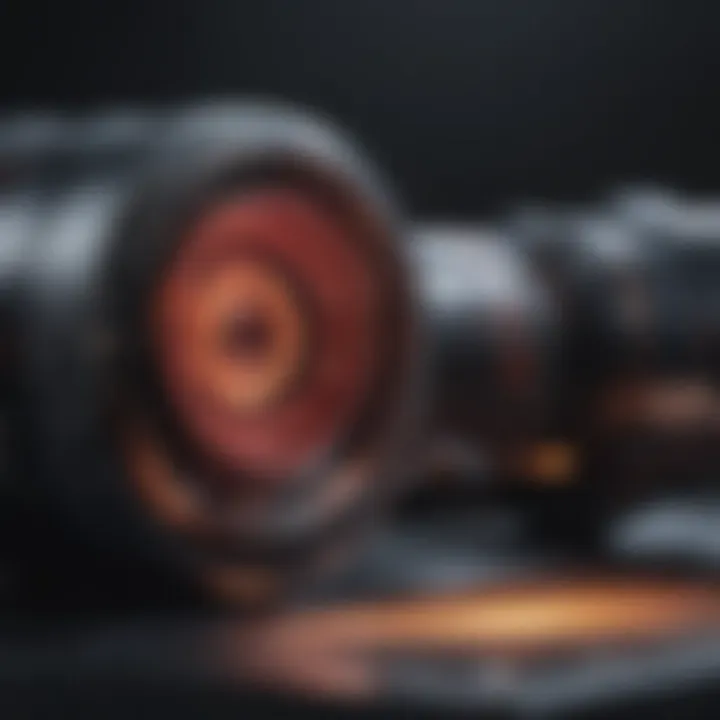
Population inversion is an essential condition for laser operation. It occurs when more electrons are in an excited state than in a lower energy state. Several mechanisms can achieve this, including optical pumping and electrical pumping. The main advantage of population inversion is that it enables more stimulated emissions to occur than absorption processes, contributing to laser action. In this article, understanding population inversion is key because it lays the groundwork for the efficiency of laser systems.
Applications of Population Inversion
Population inversion has various applications across different laser technologies. It is a crucial mechanism in solid-state lasers, gas lasers, and semiconductor lasers, allowing each type to achieve efficient light amplification. By grasping how population inversion functions, researchers and engineers can innovate and optimize laser designs for specialized applications, including telecommunications and medical devices.
The theoretical foundations outlined here illustrate the critical components that make lasers work. Their understanding is not only pivotal for the advancement of laser technology but also essential for fostering future innovations.
Components of a Laser System
The components of a laser system are crucial to its operation and functionality. Each element plays a specific role in generating the coherent light that lasers are known for. Understanding these components helps to appreciate how lasers work and their diverse applications in various fields. A well-designed laser system can increase efficiency and precision, leading to better outcomes in medical, industrial, and scientific applications.
Gain Medium
The gain medium is essential in the lasing process. It provides the material necessary for the stimulated emission of photons.
Types of Gain Media
There are several types of gain media used in laser systems, including solid-state, liquid, and gas. Solid-state gain media often consist of crystals or glass doped with ions, such as neodymium-doped yttrium aluminum garnet (Nd:YAG). This type is popular due to its stability and efficiency. Liquid gain media, like dye lasers, allow for tunability in wavelengths, making them versatile for various applications. Gas gain media, such as in CO2 lasers, are commonly used for cutting and welding due to their high power output.
The choice of gain medium impacts the overall performance of the laser system significantly. Each type has its unique characteristics, providing specific benefits depending on the application. For instance, solid-state lasers generally have a low maintenance requirement but may involve costly setups. In contrast, gas lasers can be less expensive but require a more complex operation.
Selection Criteria
When selecting a gain medium, several criteria must be considered, including efficiency, output wavelength, and cost. The efficiency of the gain medium is a crucial factor; higher-efficiency materials can generate more laser light from the same energy input. Output wavelength is also important; certain applications can only use specific wavelengths. For example, Nd:YAG lasers emit infrared light, making them suitable for certain medical applications but limiting their use in others.
One unique feature of gain medium selection is how it can affect the spectral properties of the laser. This has direct implications for applications like spectroscopy, where different wavelengths interact differently with materials, affecting measurement outcomes. The tradeoff can involve balancing performance and application needs.
Optical Cavity
An optical cavity reflects light back and forth through the gain medium. The design of this cavity profoundly influences the laser's behavior and output.
Design and Functionality
The design of the cavity is a key factor that determines the laser's efficiency and output characteristics. The functionality of an optical cavity relies on the arrangement and quality of its mirrors. These mirrors help build up light intensity, essential for effective stimulation of the gain medium.
Optical cavities can vary in design, leading to different modes of operation. Some configurations provide higher modes for specific applications, while others may focus on efficiency. A well-optimized cavity can lead to a more stable and powerful laser output.
Types of Cavities
There are primarily two types of optical cavities found in laser systems: resonant cavities and non-resonant cavities. Resonant cavities are the most common and facilitate the buildup of light through multiple reflections between mirrors, while non-resonant cavities may allow for more complex light behavior sometimes beneficial for specific applications.
The unique feature of resonant optical cavities is their ability to create a standing wave pattern. This characteristic is beneficial for applications requiring precise measurements, such as in metrology. However, non-resonant cavities can sometimes offer easier maintenance and operational flexibility, depending on the user's needs.
Pumping Mechanisms
Pumping is the process of supplying energy to the gain medium to achieve population inversion. This is necessary for the laser action to occur.
Optical Pumping
Optical pumping uses light to excite electrons in the gain medium. High-intensity light sources, like flashlamps or LEDs, can be employed to achieve the desired population inversion.
One of the key advantages of optical pumping is its ability to provide high energy input quickly, leading to rapid pulsing of the laser output. Optical pumping is popular in many solid-state lasers for its efficiency and ability to offer variable output levels.
Electrical Pumping
Alternatively, electrical pumping involves using electrical currents to energize the gain medium. This technique is often used in semiconductor lasers where direct electrical excitation can lead to effective population inversion.
The main advantage of electrical pumping is its compactness and efficiency, especially in diode lasers widely used in consumer electronics. However, the requirement for specific materials for optimal efficiency can sometimes limit its application range in higher power laser systems.
Output Couplers
Output couplers are vital in determining how much laser light escapes from the optical cavity. Their design influences laser performance and efficiency.
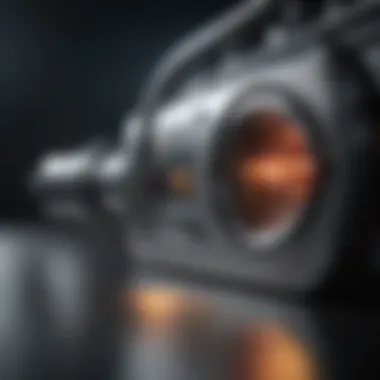
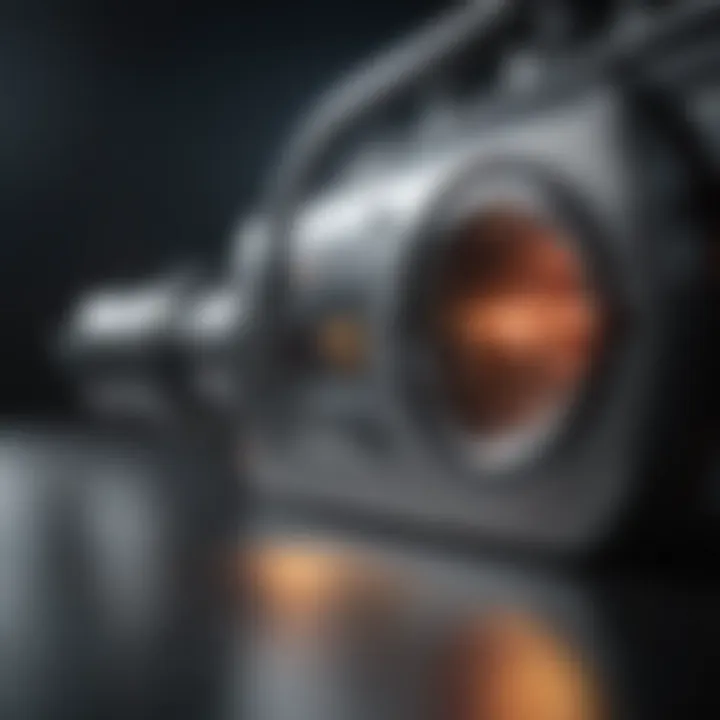
Output couplers are typically partially reflective mirrors. They allow a portion of the light to exit while reflecting the rest back into the cavity. The ratio of reflected to transmitted light can be adjusted, affecting the power output and characteristics of the laser beam.
This critical component ensures that the laser beam has the desired quality for its intended application, whether in medical usage for precision cutting or in industrial settings for high-efficiency processing.
Types of Lasers
The classification of lasers into distinct types is crucial in understanding their broader implications and uses. Each laser type serves specific functions and is designed based on certain principles, which affect its performance and application. By exploring various types of lasers, this article covers several important facets, including efficiency, power output, and operational method. This detailed approach will clarify how different lasers can be employed effectively in various fields such as medicine, manufacturing, and telecommunications.
Solid-State Lasers
Characteristics and Examples
Solid-state lasers are a category that utilize a solid gain medium to amplify light. One major aspect of solid-state lasers is their robustness and versatility, which makes them suitable for a plethora of applications.
A key characteristic of solid-state lasers is their high power output, which makes them highly effective in industry settings. For example, the Nd:YAG (Neodymium-doped Yttrium Aluminum Garnet) laser is widely used for metal cutting and welding. This powerful laser can deliver significant energy due to its efficient energy conversion.
Another unique feature is their ability to be configured for various wavelengths. This means they can be adapted for specific applications, whether in surgery or manufacturing. While solid-state lasers offer many advantages, they can also be costly and require precise cooling methods to ensure safe operation.
Gas Lasers
Common Types and Uses
Gas lasers operate using a gas as the gain medium, with the most common being the He-Ne (Helium-Neon) laser. A notable characteristic of gas lasers is their simplicity and low cost, making them ideal for beginners and educational purposes.
The key advantage of gas lasers lies in their continuous wave output, which provides a stable beam. This is particularly beneficial in applications like laser pointers and barcode scanning. However, gas lasers may not offer the same level of power as solid-state lasers, making them less suitable for heavy industrial tasks. Their output might also be limited by the availability of suitable gas mixtures and the efficiency of gas discharge between electrodes.
Fiber Lasers
Benefits and Applications
Fiber lasers utilize optical fibers doped with rare-earth elements like ytterbium or erbium as the gain medium. One of the main advantages of fiber lasers is their compact size and high efficiency. These qualities allow for easier integration into manufacturing systems.
An important aspect of fiber lasers is their excellent beam quality, resulting in high precision for applications such as engraving and cutting. They also exhibit low maintenance requirements because the fiber medium eliminates many components present in other laser types. However, they can also be more complex to set up initially, requiring careful design to optimize performance and to meet specific user needs.
Semiconductor Lasers
Industry Applications
Semiconductor lasers, or diode lasers, are composed of semiconductor materials that emit light when electrically biased. The high efficiency of semiconductor lasers makes them a staple in various industries, particularly in telecommunications and consumer electronics.
The strength of these lasers lies in their integrability and miniaturization. With compact sizes, they can be built directly into devices like CD players and optical communication systems. However, they may suffer from limitations in output power compared to other types, which could limit their applications in heavy-duty environments.
In summary, the types of lasers discussed above each bring unique features and capabilities that cater to various needs across industries. Understanding these differences is essential to harnessing laser technology effectively in both current practices and future advancements.
Applications of Laser Technology
Lasers have transformed numerous industries due to their precision, speed, and versatility. The impact of laser technology extends well beyond simple illumination. Its applications span medical fields, industrial processes, communication systems, and scientific explorations. This article highlights several critical areas where laser technology plays a vital role.
Medical Applications
Surgery
Laser surgery offers specific advantages in modern medicine. It enables more precise incisions compared to traditional blades. This precision leads to reduced blood loss and faster recovery times for patients. Moreover, the ability to minimize damage to surrounding tissues is a notable characteristic of laser surgery. Its use in procedures such as LASIK eye surgery and various dermatological applications has made it a dependable choice. However, the unique feature of laser surgery can also come with disadvantages. Not every procedure is suitable for lasers, and sometimes cost can be a factor.
Therapeutic Uses
In addition to surgical applications, lasers are used for therapeutic purposes. Phototherapy utilizes laser light to treat skin conditions like psoriasis and acne. The key characteristic of therapeutic laser use is its ability to stimulate tissue repair and reduce inflammation. This non-invasive approach is beneficial for patients seeking relief from chronic pain or skin disorders. On the other hand, the unique feature of these therapeutic applications is that their effectiveness may vary among individuals. Although generally safe, there can also be side effects in sensitive patients.
Industrial Applications
Material Processing
Material processing through lasers has become indispensable in manufacturing. Lasers can cut, engrave, and weld materials with high efficiency and precision. This characteristic is why many industries utilize lasers for tasks that require exactness. The unique advantage of laser processing is the minimal heat-affected zone, leading to better quality finishes. However, lasers may not be suitable for all materials, which can limit their applicability in certain situations.
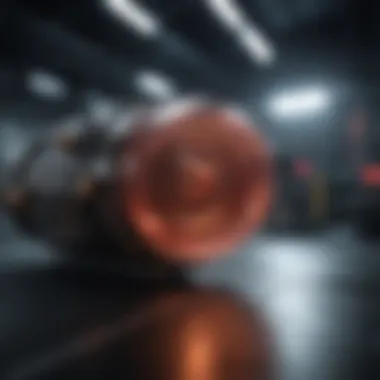
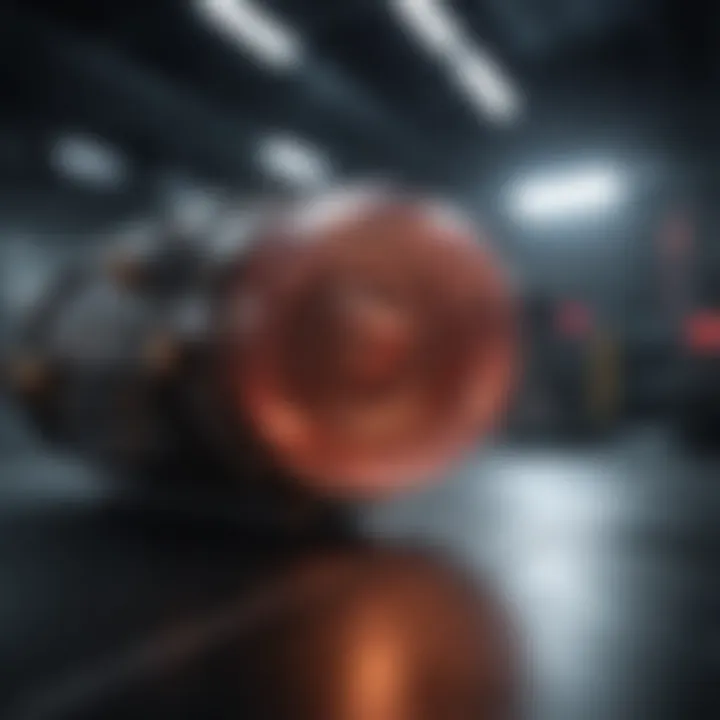
Manufacturing Techniques
In the realm of manufacturing, laser technologies enhance various techniques. For instance, additive manufacturing, commonly known as 3D printing, often utilizes lasers to fuse materials together layer by layer. The key characteristic here is the ability to create complex geometries that traditional methods might not achieve. This innovative approach is a popular choice among designers. Nevertheless, the unique feature of laser-based manufacturing is its reliance on specific material compatibility, and not all materials can be used effectively.
Communication Technologies
Fiber Optics
Fiber optics technology relies on lasers for transmitting data over long distances. The critical advantage of this method is the high bandwidth, allowing for fast communication. Fiber optics is popular in various sectors including telecommunications and internet services. A unique feature of fiber optics is the almost complete immunity to electromagnetic interference, making it a reliable choice for data transmission. However, the installation and maintenance of fiber optic systems can be costly.
Data Transmission Security
In the context of data transmission security, lasers contribute to the development of secure communication channels. The significance of using lasers in this area is that they can provide encrypted data transfer. This contributes to the overall goal of enhancing cybersecurity. The unique feature of laser-based encryption methods is their difficulty to intercept. While promising, these systems also face challenges, such as the need for precise alignment of equipment over time.
Scientific Research
Spectroscopy
Spectroscopy is a crucial field that extensively utilizes laser technology for studying materials. The ability to analyze chemical compounds and their interactions is essential for various scientific disciplines. Spectroscopy provides detailed information about molecular structures. The key characteristic of laser-based spectroscopy is its sensitivity, allowing researchers to detect even minute quantities of substances. However, a drawback is that it often requires sophisticated equipment and technical expertise.
Laser Interferometry
Laser interferometry has significant implications in scientific research, particularly in measuring distances and detecting gravitational waves. The unique aspect of this technology is its exceptional precision. This high level of accuracy is crucial for experiments that require minimal deviation. While laser interferometry is beneficial for many fields, including physics and engineering, it demands careful calibration and environmental control, which may be challenging in some scenarios.
"The versatility and precision of laser technology make it a cornerstone in medical, industrial, communication, and scientific applications."
Overall, the applications of laser technology illustrate its multifaceted role in advancing methodologies across various sectors. Understanding these applications not only highlights the importance of lasers but also sets the stage for ongoing innovations.
Future Trends in Laser Technology
The exploration of future trends in laser technology holds significant importance within the context of this article. As innovations continue to advance, understanding their implications becomes essential. The field of laser technology is evolving, encompassing emerging technologies and integrations with artificial intelligence, among other areas. This evolution can lead to enhanced efficiencies, expanded applications, and novel solutions that address contemporary challenges across industries.
Emerging Technologies
Quantum Lasers
Quantum lasers represent a frontier in laser technology, leveraging quantum mechanical principles for enhanced performance. One notable characteristic of quantum lasers is their ability to achieve unprecedented levels of precision and efficiency. This feature makes them a promising choice for various applications ranging from telecommunications to medical treatments. Quantum lasers harness coherence properties at the quantum level, leading to improved signal clarity and reduced energy consumption.
A unique aspect of quantum lasers is their capacity to function effectively at reduced power levels. This opens up possibilities for portable devices and applications in constrained environments, where energy resources may be limited. However, the technology is still developing, and practical challenges exist, including materials used and the integration of quantum systems into existing frameworks.
Plasma Lasers
Plasma lasers offer another innovative direction in the evolution of laser technology. These lasers capitalize on high-energy plasma states to generate powerful and short laser pulses. A key characteristic of plasma lasers is their ability to generate extreme intensities, making them suitable for applications in materials processing and scientific research.
One unique feature of plasma lasers is their adaptability in different conditions. This advantage allows for the exploration of new environments either on Earth or in space. Yet, this technology is still in a nascent stage and may face challenges related to cost and the complexity of the systems needed.
Integration with AI
The integration of artificial intelligence into laser technology promises to transform its applications further. AI can assist in optimizing laser processes in manufacturing, enhancing precision in medical procedures, and improving data analysis speeds. By utilizing machine learning algorithms, systems can adapt to variables in real-time, leading to more efficient operations and reduced waste/error rates.
"The advancement in laser technologies, especially with quantum principles and plasma applications, illustrates a fundamental shift in operational efficiencies and application scope across diverse industries."
Such innovations will likely impact a wide array of fields, making a thorough understanding of these trends vital for students, researchers, educators, and professionals.
Finale
In this article, we have explored various facets of laser technology, shedding light on its principles, applications, and innovations. The importance of understanding the laser principle is substantial in today's technological landscape. Lasers have become integral tools across multiple disciplines, from medicine to communications. Their unique properties such as coherence and monochromaticity allow for precision and efficacy that traditional sources of light cannot achieve.
Summary of Key Points
Throughout our discussion, several key points have been highlighted:
- Definition and Historical Context: We began by defining lasers and tracing their development from early experiments to the establishment of laser technology.
- Theoretical Foundations: The principles of stimulated emission, coherence, and population inversion were discussed, providing a solid framework for understanding laser operation.
- Components of Laser Systems: Essential components like gain media, optical cavities, and pumping mechanisms were detailed, illustrating how these elements work together to generate laser light.
- Types and Applications: Various types of lasers were examined, including solid-state, gas, fiber, and semiconductor lasers, along with their applications in fields such as medicine, manufacturing, and telecommunications.
- Future Trends: Emerging technologies and the integration of lasers with AI indicate that the field will continue to evolve and expand.
"Understanding the complexities of laser technology opens doors to innovations"
Significance for Future Applications
The significance of lasers in future applications cannot be overstated. As industries adapt to new challenges, laser technology will play a crucial role in enhancing efficiency and capabilities. For instance, in medicine, as precision becomes more paramount in surgeries, lasers provide a non-invasive alternative with better recovery times. In manufacturing, laser cutting and welding techniques improve precision and reduce waste, contributing to sustainable practices.
Moreover, advancements in quantum and plasma lasers suggest a future where new types of laser sources can be harnessed for applications we have yet to imagine. In telecommunications, lasers will continue to be fundamental in improving data transmission speeds and security.