Understanding Primary and Secondary Antibodies in Western Blotting
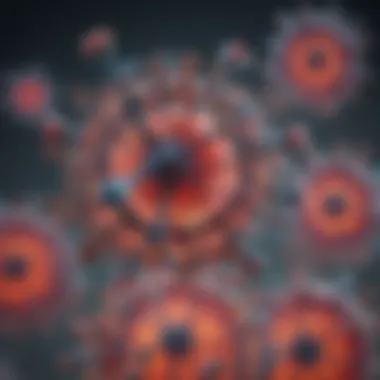
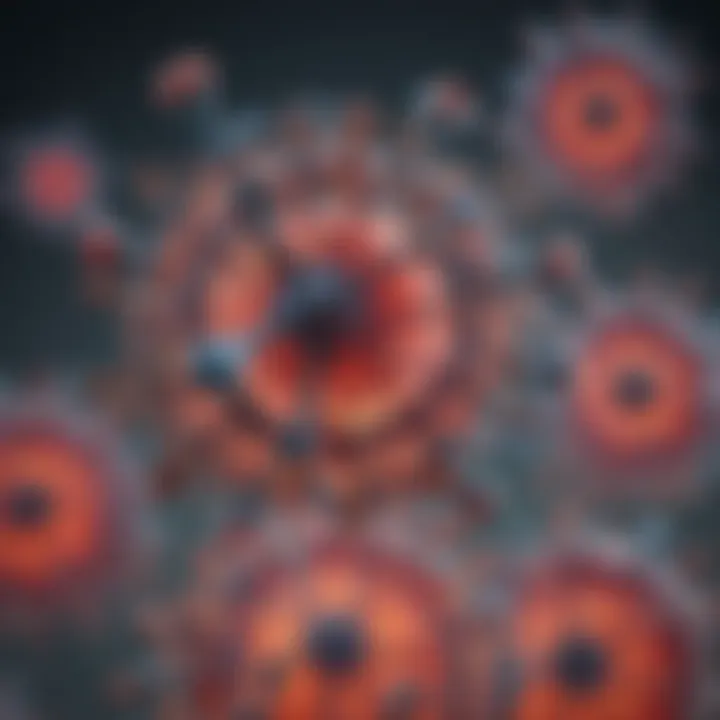
Intro
Western blotting stands as a crucial technique in molecular biology. It allows researchers to detect specific proteins in a sample, shedding light on various biological processes. Central to this method are primary and secondary antibodies. These antibodies play distinct but complementary roles in the detection and quantification of proteins, serving as the backbone of the western blotting process. Understanding the nuances of these antibodies can significantly enhance the efficacy of experimental outcomes. This article aims to explore those roles in depth, providing insights that benefit students, researchers, educators, and professionals.
Research Highlights
Key Findings
The significance of primary and secondary antibodies in western blotting cannot be overstated. Primary antibodies bind specifically to the target protein, enabling targeted detection. In contrast, secondary antibodies, which are often conjugated to enzymes or fluorophores, amplify the signal once the binding occurs. This mechanism allows for both increased sensitivity and specificity in protein detection. Key findings from recent studies illustrate that:
- Selecting high-quality primary antibodies tailored to the specific protein of interest enhances detection accuracy.
- Utilizing secondary antibodies with low cross-reactivity minimizes background noise in the results.
- The ratio and concentration of primary and secondary antibodies can significantly influence the sensitivity of the assay.
Implications and Applications
The insights gained from understanding the dynamics of these antibodies extend beyond the lab bench. Improved antibody selection and usage pave the way for more reliable data interpretation. Consequently, researchers can better assess protein expression levels in various conditions, leading to advancements in:
- Disease diagnosis and treatment strategies.
- Understanding cellular mechanisms in health and disease.
- Developing novel therapeutics based on protein interactions.
Methodology Overview
Research Design
The methodology related to western blotting encompasses various critical design considerations. Each step is tailored to optimize the interactions between antibodies and proteins. Thus, a robust methodology enables reproducible and reliable results. Essential components of the design include:
- Sample Preparation: Careful lysate preparation is vital to preserve protein integrity.
- Gel Electrophoresis: Sodium dodecyl sulfate polyacrylamide gel electrophoresis (SDS-PAGE) separates proteins based on size.
- Transfer: Proteins are transferred to a membrane, which facilitates antibody binding.
Experimental Procedures
Experimental procedures must adhere to established protocols for successful outcomes. Important steps include:
- Blocking: Minimizing nonspecific binding through the use of blocking agents such as BSA or non-fat dry milk.
- Primary Antibody Incubation: Applying the right concentration and time can vastly improve the specificity of the assay.
- Detection: Following the incubation with secondary antibodies, detection methods such as chemiluminescence or fluorescence provide visualization of the protein bands.
Adhering to these methodologies leads to consistent results, which are essential for effective data analysis and interpretation.
"The careful selection and implementation of primary and secondary antibodies are pivotal for successful western blotting, significantly impacting the overall clarity of experimental findings." - Expert Researcher
By integrating detailed understanding and advanced methodologies, researchers can harness the full potential of western blotting to yield insightful biological data.
Prolusion to Western Blotting
Western blotting is a crucial technique in molecular biology for detecting specific proteins in a sample. The importance of this technique stems from its versatility and ability to provide insights into protein expression and regulation. By using specific antibodies, researchers can identify proteins of interest even in complex mixtures. This capability is essential in various fields such as biochemistry, immunology, and pathology.
Overview of the Technique
Western blotting involves several steps that allow for the effective separation and identification of proteins. The process begins with gel electrophoresis, where proteins are separated based on size. Once separated, proteins are transferred to a membrane, usually made of nitrocellulose or PVDF. This is followed by the application of primary and secondary antibodies, which are essential for the detection process.
The role of primary antibodies is to bind to the target protein, while secondary antibodies facilitate the visualization process. Secondary antibodies are labeled with enzymes or dyes that produce a detectable signal upon interaction with the primary antibodies. This multi-step process enhances specificity and sensitivity, making it possible to detect even low-abundance proteins.
Here are key components of the technique:
- Sample Preparation: Cells or tissue samples are lysed to extract proteins.
- Gel Electrophoresis: Proteins are separated by size.
- Transfer to Membrane: Proteins are transferred from the gel to a solid support.
- Blocking: Non-specific sites on the membrane are blocked to prevent background signal.
- Antibody Incubation: Both primary and secondary antibodies are used for detection.
- Signal Development: Detection methods reveal the presence of the target protein.
Historical Background and Development
The Western blot technique was first introduced in the 1970s. Its origins trace back to the need for a reliable method to identify specific proteins in complex samples. The name "Western blot" was coined in 1979 as a play on the existing techniques labeled as Eastern and Southern blots, which focus on DNA and RNA, respectively. Over the years, the methodology has evolved considerably.
Initially, the protocols were labor-intensive and often yielded inconsistent results. However, technological advancements have led to improved specificity and reproducibility of results. For instance, the introduction of enhanced chemiluminescence and various labeling methods has improved detection sensitivity.
Furthermore, the Western blot technique has undergone standardization, making it a reliable assay in research and clinical settings. Researchers today benefit from a wealth of protocols, kits, and reagents designed to optimize the technique, ensuring accurate analysis of protein expression and function.
Understanding Antibodies
In the context of Western blotting, a deep understanding of antibodies is essential for accurate and reliable results. Antibodies are proteins that play a central role in the immune response, and their usage in laboratory diagnostics and research is foundational. Primary antibodies are designed to target a specific protein of interest, while secondary antibodies provide an amplification mechanism to enhance detection. This distinction is crucial because the selection and effectiveness of these antibodies directly correlate with the quality of the analysis. Choosing the right antibodies can significantly affect sensitivity, specificity, and ultimately, the success of an experiment.
Moreover, understanding the properties and functionality of antibodies helps researchers optimize their protocols and anticipate potential issues that can arise during analysis. Considerations such as cross-reactivity, binding affinity, and the method of detection play pivotal roles in ensuring the reliability of the obtained data.
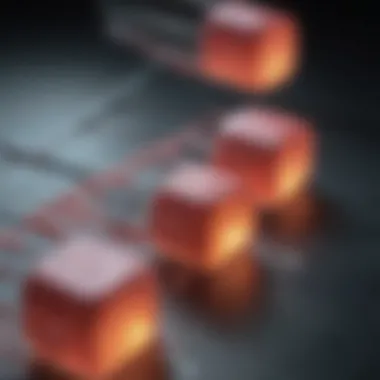
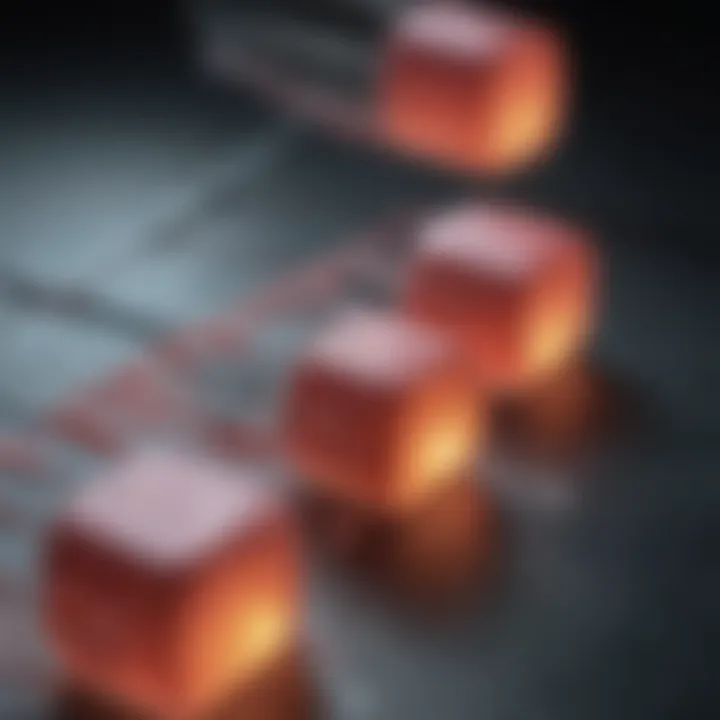
What are Primary Antibodies?
Primary antibodies are crucial components in the Western blotting process. They bind specifically to the target protein, enabling its detection. These antibodies can be derived from various species, including rabbits, mice, and goats, and they can be either polyclonal or monoclonal. Polycolonal antibodies are produced by different B cell lines, resulting in a mixture that can recognize multiple epitopes on a single protein. Conversely, monoclonal antibodies originate from a single clone of B cells, thereby offering higher specificity to one particular epitope.
When selecting primary antibodies, researchers must consider several aspects:
- Source of the antibody: Understanding which species the antibody was raised in is critical as it affects cross-reactivity and experimental design.
- Immunogen: Information about the immunogen used to generate the antibody helps determine its specificity for the target protein.
- Validation data: Reliable antibodies come with comprehensive validation data to ensure they perform consistently in experiments.
By understanding these foundational elements, researchers can make educated choices that enhance the accuracy and reliability of their findings.
Characteristics of Secondary Antibodies
Secondary antibodies serve as facilitators in the Western blotting workflow. They bind to the primary antibodies and are generally linked to a detectable marker, such as enzymes or fluorophores. This amplification mechanism allows clearer visualization of proteins of interest, thus making detection more sensitive.
Some essential characteristics of secondary antibodies include:
- Species-specificity: Secondary antibodies are often raised against the species in which the primary antibody was produced. For example, if the primary antibody is raised in a mouse, then a goat anti-mouse secondary antibody will be appropriate.
- Labeling: The type of label attached to the secondary antibody influences the detection method. Common labels include horseradish peroxidase (HRP) for colorimetric detection or fluorescent dyes for imaging.
- Cross-reactivity: A well-chosen secondary antibody should have minimal cross-reactivity with non-target proteins. High specificity ensures that only the antibodies bound to the target protein are visualized effectively.
These characteristics enhance the overall performance of the antibody-based assay and optimize the experimental results. The interplay between primary and secondary antibodies creates a robust platform for protein analysis in a variety of fields, making the understanding of these molecules vital for scientific advancements.
The Role of Primary Antibodies in Western Blotting
Primary antibodies are crucial in the western blotting technique. Their primary function is to bind specifically to the target protein, which allows researchers to identify and quantify these proteins in complex samples. Without effective primary antibodies, the entire ethos of western blotting—precise detection—dissolves. This highlights the importance of selecting antibodies that not only recognize the target but do so with high affinity and specificity.
By understanding the role of primary antibodies, researchers can enhance the reliability of their results. They must consider several factors when choosing primary antibodies, such as their concentration, binding characteristics, and compatibility with the secondary antibodies they plan to use. The quality of the primary antibody directly impacts the clarity of the signal obtained in the final outcome. Thus, a deep comprehension of their role holds significance for optimal experimental results.
Selection Criteria for Primary Antibodies
Selecting the right primary antibody involves multiple criteria, each aiming to ensure effective protein detection. The first consideration is specificity; the antibody must recognize the intended target protein with minimal cross-reactivity with non-target proteins. This specificity can often be validated by examining data from previous studies or protocols. Another factor is the source of the antibody. Primary antibodies are often generated from different species, such as rabbits, mice, or goats. Knowing the host origin may influence the choice of the secondary antibody used later in the process.
Additionally, the availability of different antigen epitopes plays a role. The selected primary antibody must bind to an epitope on the protein that is available after gel electrophoresis and transfer. Researchers might also consider the antibody's isotype, which can affect the downstream applications and outcomes.
Affinity and Specificity
Affinity and specificity are two intertwined concepts in the context of primary antibodies. Affinity refers to the strength of the binding interaction between the antibody and its specific target. A high-affinity antibody binds tightly to the target protein, reducing background noise and enhancing the signal.
Specificity, on the other hand, denotes how selective the antibody is for its target. An antibody with high specificity will showcase little to no binding to non-target proteins, reducing false positive signals. Researchers frequently employ methods such as ELISA, dot blots, or pre-absorption assays to characterize these attributes before commencing their western blotting experiments.
"A primary antibody must possess both high affinity and specificity for accurate protein detection in western blotting."
Application in Protein Detection
The main application of primary antibodies in western blotting is to detect and quantify specific proteins of interest from a mixture. After gel electrophoresis, proteins are transferred to a membrane, where the primary antibody is introduced. This interaction forms a complex that is essential for subsequent detection steps.
The detection of the specific proteins involves utilizing various labeling techniques applied to the primary antibodies. For instance, if the primary antibody is conjugated to a fluorescent dye, it enables visualization of the protein under a suitable imaging system. Other methods include the use of enzymatically labeled primary antibodies, which create a detectable colorimetric change.
Selecting the right antibody and understanding its role allows researchers to delve deeper into the protein landscape of a sample, facilitating studies on protein expression, modification, and interactions.
The Role of Secondary Antibodies in Western Blotting
Secondary antibodies play a critical role in the western blotting process. They amplify the signal generated by primary antibodies, enhancing the detection of specific proteins. The importance of this function cannot be overstated. Without secondary antibodies, the sensitivity and specificity of protein detection would be greatly diminished. Secondary antibodies are designed to bind to primary antibodies, and they are often labeled with enzymes or fluorescent tags to facilitate visualization of the target protein.
Purpose of Secondary Antibodies
The primary purpose of secondary antibodies is to bind specifically to the primary antibodies that have attached themselves to the target protein. This binding creates a bridge that can be exploited for signal amplification. By doing so, secondary antibodies allow for increased detection sensitivity. The presence of multiple secondary antibodies can significantly boost the signal, making it easier to visualize proteins even in complex mixtures. Additionally, secondary antibodies can introduce further layers of specificity. If they are polyclonal antibodies, they can bind to various epitopes on the primary antibody, enhancing the overall capture of the target.
Enhancing Signal Detection
Enhancing signal detection is one of the most important functions of secondary antibodies. This enhancement comes from the manner in which secondary antibodies are conjugated to detection agents. For example, enzymes such as horseradish peroxidase (HRP) or alkaline phosphatase can be used. These enzymes catalyze reactions that produce colored products or generate luminescent signals. Fluorescently labeled secondary antibodies can also be utilized to allow for easy identification under a fluorescence microscope.
By using secondary antibodies that have high affinity and are conjugated to powerful labels, researchers can detect low-abundance proteins that would otherwise remain hidden in a blot.
Types of Secondary Antibodies
There are several types of secondary antibodies, each catering to specific experimental needs. They can be classified based on their source and their labeling method:
- Polyclonal Secondary Antibodies: These are derived from multiple clones of B cells and can recognize multiple epitopes on the primary antibody. This gives them a higher propensity to bind and enhance signal detection.
- Monoclonal Secondary Antibodies: These are produced by identical immune cells and recognize a single epitope. They offer consistency and specificity but may be less effective in certain applications compared to polyclonal antibodies.
- Conjugated Secondary Antibodies: These antibodies are labeled with enzymes or fluorophores. Their choice depends on the detection method used in the experiment. For instance, HRP is commonly used for colorimetric detection, while fluorescein isothiocyanate (FITC) is used for fluorescence.
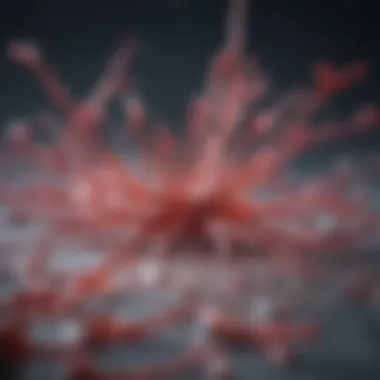
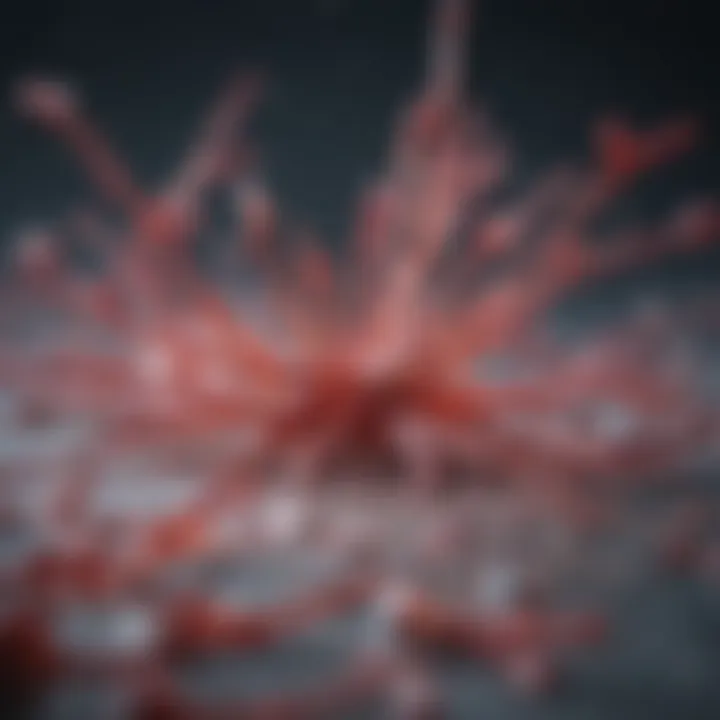
Each type of secondary antibody has its advantages and considerations. Choosing the right one depends on the specific goals of the western blotting experiment, including the required sensitivity and detection method.
Antibody Labeling Techniques
In Western blotting, the effectiveness of protein detection heavily relies on antibody labeling techniques. These techniques enhance the visibility of the proteins of interest and ensure accurate results. Through proper labeling, researchers can better distinguish specific proteins on the membrane, allowing for precise quantification and analysis. The choice of labeling technique is critical, as it affects sensitivity, signal strength, and overall assay quality.
Two prominent types of labeling techniques are enzymatic labels and fluorescent labels. Each of these methods comes with its unique benefits and considerations that researchers must weigh when selecting the appropriate strategy for their experiments.
Enzymatic Labels
Enzymatic labels use enzymes attached to antibodies to generate a detectable signal. Common enzymes include horseradish peroxidase (HRP) and alkaline phosphatase (AP). When substrates are added to the system, the enzymes catalyze reactions to produce colored or luminescent products. This process enables the visualization of bound antibodies and, by extension, the target proteins.
The advantages of enzymatic labels include:
- High sensitivity: Enzymatic reactions can amplify signals, allowing for the detection of low-abundance proteins.
- Versatility: Numerous substrates are available, each producing different detectable signals, making it adaptable to various settings.
- Cost-effectiveness: Enzymatic reactions often use common laboratory reagents, reducing the overall expense of the procedure.
However, some drawbacks exist. The enzymatic reactions can be sensitive to environmental conditions, such as temperature and pH, which may require specific experimental conditions. Thus, proper optimization is essential for reliable results.
Fluorescent Labels
Fluorescent labels utilize fluorescent dyes to tag antibodies. In response to specific wavelengths of light, these dyes emit fluorescence, allowing for visualization of the antibody-protein complexes through techniques such as fluorescence microscopy or imaging systems. Common fluorescent markers include FITC and Alexa Fluor.
The benefits of fluorescent labels include:
- Multicolor labeling: Researchers can use multiple fluorescent tags to detect various proteins simultaneously, making it easier to analyze complex samples.
- Quantitative data: Fluorescence allows for quantitative analysis, providing detailed measurements of protein expression levels.
- Compatibility with imaging techniques: Advanced imaging methods can enhance the visualization quality, helping to obtain clearer and more informative results.
Nevertheless, fluorescent labels may present challenges such as photobleaching, where the fluorescent signal diminishes over time when exposed to light. Additionally, the selection of appropriate filters and the need for specialized imaging equipment can increase the complexity of the experiment.
The choice between enzymatic and fluorescent labeling depends on factors such as the experimental context, protein abundance, and the desired sensitivity. Each method serves unique purposes in Western blotting, underscoring the importance of strategic selection.
In summary, understanding the intricacies of antibody labeling techniques is vital for achieving reliable outcomes in Western blotting. By carefully selecting between enzymatic and fluorescent labels, researchers can enhance the detection of proteins, paving the way for meaningful results in their experiments.
Optimization of Antibody Concentration
Optimizing the concentration of antibodies in Western blotting is a crucial step that significantly impacts the quality of results. Proper optimization ensures that the antibody binds specifically to the target protein without excessive background noise. This balance is essential for achieving clear and interpretable signals in the final blot. An inadequate concentration can lead to poor detection, while an excess may yield nonspecific binding, complicating data interpretation.
Factors Affecting Antibody Concentration
Antibody concentration is influenced by various factors that researchers must consider during experimentation:
- Antibody Affinity: Higher affinity antibodies can often work effectively at lower concentrations. Conversely, antibodies with lower affinity may require higher concentrations to achieve adequate binding to the target.
- Protein Abundance: If the target protein is present in low amounts in a sample, a higher concentration of antibody might be necessary to ensure adequate detection.
- Sample Complexity: In biological samples, the presence of various proteins can lead to masking of the target. Optimizing the concentration is essential to improve the chances of specific binding amidst complex backgrounds.
- Detection Method: The choice of detection method (e.g., chemiluminescence, fluorescence) can dictate antibody requirements. Different methods may demand varying levels of sensitivity, which influences optimal concentrations.
As a result of these factors, it is critical to evaluate and adjust the concentration of both primary and secondary antibodies carefully.
Experimental Protocols for Testing Concentration
Testing antibody concentration is a systematic process that often follows several steps:
- Preparation of Dilution Series: Prepare a dilution series of the antibody. This typically involves making a series of dilutions in a buffer solution. Common dilutions might range from 1:100 to 1:10,000, depending on the initial concentration of the antibody.
- Sample Loading: Load equal amounts of sample containing the targeted protein onto the gel. Ensure that all other variables, such as buffer composition and gel concentration, remain constant.
- Blotting Procedure: Conduct the Western blotting process following standard protocols. This includes transferring the proteins from the gel to the membrane and blocking non-specific binding sites.
- Incubation with Antibodies: Incubate the membrane with the diluted primary antibody for a determined time, often overnight at 4°C or 1-2 hours at room temperature.
- Membrane Washing: Wash the membrane to remove unbound antibodies. This step helps reduce background noise and improve signal clarity.
- Detection: Proceed with secondary antibody incubation and detection according to the chosen method (e.g., using horseradish peroxidase for chemiluminescent detection).
- Analysis of Results: Analyze the blots for clarity and intensity of the bands. Compare the signal strengths across different dilutions to ascertain which concentration yields optimal results.
By following a structured approach for optimizing antibody concentration, researchers can enhance the reliability of their findings and facilitate more precise protein detection in Western blotting.
Common Challenges in Antibody Use
The use of antibodies in western blotting is pivotal, but it comes with several challenges. Understanding these common challenges is essential for ensuring accurate results and reliable interpretations in experiments. Among the issues, two often emerge as significant: cross-reactivity and non-specific binding. Addressing these challenges is key to optimizing antibody utility and enhancing the robustness of experimental findings.
Cross-Reactivity Issues
Cross-reactivity refers to the ability of an antibody to bind to multiple antigens or proteins, which can lead to misleading results. This occurs when an antibody is not exclusively selective for the target protein. In western blotting, this can manifest as additional bands on the membrane, leading researchers to incorrectly identify proteins.
To reduce cross-reactivity, it is crucial to:
- Select well-validated antibodies: Using antibodies that have been rigorously tested on similar systems can help minimize unexpected reactivity.
- Optimize dilution: Adjusting antibody concentration can mitigate background noise caused by cross-reactivity.
- Employ blocking reagents: These types of reagents can inhibit non-specific binding sites on the membrane, reducing the chance of cross-reactivity.
Being aware of potential cross-reactive interactions allows scientists to refine their approaches and make informed decisions about the antibodies they utilize.
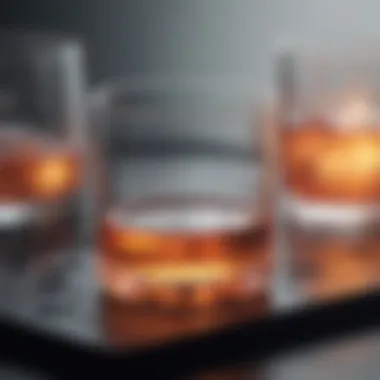
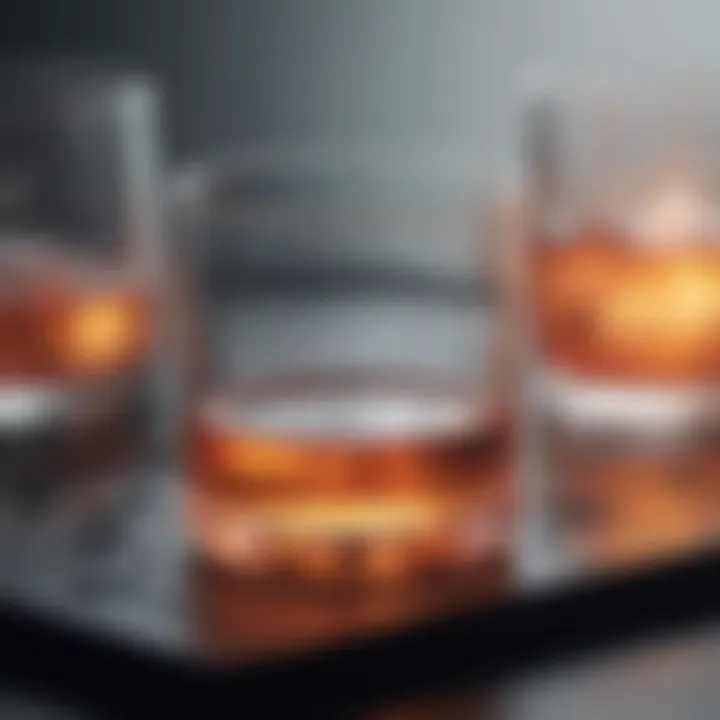
Non-Specific Binding
Non-specific binding is another prevalent challenge encountered during antibody application in western blotting. It occurs when antibodies bind to molecules that are not the specific target of interest. This can complicate the clarity of results, similar to cross-reactivity, often leading to artifacts in data interpretation.
To minimize non-specific binding, researchers can:
- Include proper controls: Utilizing controls can help discern specific bands from non-specific signals.
- Optimize washing steps: Increasing the stringency of wash buffers can help dislodge non-specifically bound antibodies.
- Use secondary antibodies with low background: Chosen secondary antibodies should display low rates of non-specific interactions, reducing unwanted signals.
By recognizing non-specific binding as a challenge, researchers can refine their techniques, increasing the validity of their data.
"The accuracy in western blot results is heavily dependent upon careful selection and application of antibodies, making it critical to address common challenges in antibody use."
Ultimately, addressing cross-reactivity and non-specific binding not only enhances data integrity but also fosters greater confidence in experimental outcomes.
Troubleshooting Western Blotting
Troubleshooting in Western blotting is crucial for obtaining reliable and reproducible results. This involves identifying and resolving issues that may arise at various stages of the procedure. Understanding these troubleshooting methods can improve the quality of data and help in accurately interpreting protein expressions. Some common challenges may include poor transfer, low signal intensity, or unexpected band patterns. The insights gained from troubleshooting can lead to significant improvements in experimental outcomes.
Interpreting Results
Interpreting results in Western blotting requires both careful analysis and an understanding of the context of the experiment. After completing the transfer and detection steps, results are usually visualized as bands on a membrane. These bands represent proteins bound by antibodies, with both intensity and position providing information about the proteins of interest.
- Position: The position of a band is indicative of the molecular weight of the target protein, which can help in confirming the identity of the protein.
- Intensity: Band intensity reflects the abundance of the protein in the sample. A stronger band indicates higher expression levels, while a faint band indicates lower levels.
It is essential to compare experimental results with proper controls. This includes analyzing positive and negative controls to determine if the observed bands are specific to the target. Any inconsistent or unexpected bands can signal issues with the experiment, such as cross-reactivity or non-specific binding, requiring a further review of methods used.
Adjusting Parameters for Improvement
Adjusting parameters is often necessary when troubleshooting Western blotting. Re-evaluating conditions can enhance protein transfer and detection. Some factors that may be adjusted include:
- Antibody concentration: Too much or too little antibody can lead to poor signal detection or high background.
- Wash conditions: Increasing the number or duration of washes can help reduce non-specific binding.
- Transfer time and conditions: Altering the voltage or transfer time may improve the transfer of proteins to the membrane.
Each of these adjustments should be made cautiously. Documenting any changes and their effects helps in developing a refined method. A structured approach to optimization can facilitate achieving optimal results.
Remember, systematic troubleshooting can turn challenges in Western blotting into opportunities for learning and refining techniques.
Future Directions in Antibody Research
The field of antibody research is evolving rapidly. As techniques and technologies advance, the role of antibodies—both primary and secondary—in applications such as Western blotting is expected to become more sophisticated. Understanding these future directions is critical for researchers aiming to improve their methodologies and outcomes. Emphasizing innovation, the potential for novel applications, and addressing emerging challenges will guide the next generation of antibody research.
Innovations in Antibody Design
Innovations in antibody design have the potential to significantly enhance the functionality and specificity of antibodies. High-throughput screening technologies are increasingly being used to identify antibodies that have higher affinity and specificity for target proteins. This approach allows researchers to rapidly generate diverse antibody libraries, improving the chances of finding optimal binders for Western blotting applications.
- Recombinant Antibodies: These are engineered antibodies that can be produced in vitro, allowing for better control over their properties. Recombinant antibodies can be customized for specific applications, enhancing their utility in various types of experiments.
- Monoclonal Antibodies: The development of hybridoma technology continues to evolve. Innovative methods, such as transgenic mice or single B-cell technologies, produce monoclonal antibodies efficiently with desired specificity.
- Synthetic Antibodies: Advances in synthetic biology enable the creation of fully synthetic antibodies that mimic natural ones. These antibodies may reduce reliance on animal models, generating less variability and improving ethical standards in research.
- Nanobodies: Derived from the immune systems of camelids, nanobodies are smaller than traditional antibodies. Their unique structure allows them to bind to specific targets that conventional antibodies may not access, making them valuable tools in both research and clinical contexts.
Potential Applications in Diagnostics
The application of antibodies in diagnostics is a promising area of future research. As understanding deepens, several potential applications emerge, which could improve disease detection and monitoring.
- Early Disease Detection: Antibodies that target specific biomarkers may enable earlier detection of conditions such as cancer or autoimmune disorders. Tailoring antibodies to identify early molecular changes could greatly enhance patient outcomes.
- Personalized Medicine: Antibodies can play a role in developing personalized treatment plans based on individual patient profiles. By targeting specific antigens present in patients, therapies can be more effective and safer.
- Point-of-Care Testing: Innovations in antibodies facilitate the development of rapid, on-the-spot diagnostic tests. These tests can be crucial in settings where laboratory access is limited, allowing for quicker medical decision-making.
The advancement of antibody technologies not only holds promise for research but also for improved healthcare outcomes through targeted diagnostics and therapeutics.
Ending
In this article, the pivotal role of primary and secondary antibodies in Western blotting is underscored. Understanding these components is essential for effective protein detection and analysis. Proper selection of antibodies is critical, as it directly influences both the specificity and the sensitivity of the assay. Researchers must consider various factors such as affinity, concentration, and labeling techniques. Moreover, navigating common challenges like cross-reactivity and non-specific binding is important for obtaining reliable results.
Summarizing Key Insights
Several insights emerge from our examination:
- Primary antibodies, chosen for their specificity, bind directly to target proteins. Their successful use is fundamental for accurate detection.
- Secondary antibodies enhance detection through signal amplification. They are typically labeled with enzymes or fluorescent tags, contributing to improved visibility of protein bands.
- The selection criteria impact the effectiveness of the Western blotting process. Researchers must ensure appropriate validation of antibodies to minimize errors.
- Considerations such as antibody concentration and proper blotted protein handling can significantly optimize results.
These insights not only facilitate a deeper understanding of the Western blotting technique but also serve to inform best practices in experimental design.
Implications for Future Research
The future of antibody research carries profound implications for molecular biology and diagnostics. Innovations in antibody design, such as synthetic and engineered antibodies, could lead to improved specificity and reduced cross-reactivity. This can drastically enhance the accuracy of Western blotting results. Developments in diagnostic applications are also promising. For instance, monoclonal antibodies tailored to specific disease markers could advance early detection techniques.
Further research into the mechanisms of antibody interactions with proteins can unveil new avenues for precision in Western blotting and beyond. As techniques evolve, staying informed about these developments will enhance the effectiveness of current assays and ensure researchers are equipped to tackle emerging challenges.
"The evolution of antibodies is crucial for advancing both research and clinical diagnostics."