Understanding Protein Function: A Comprehensive Exploration
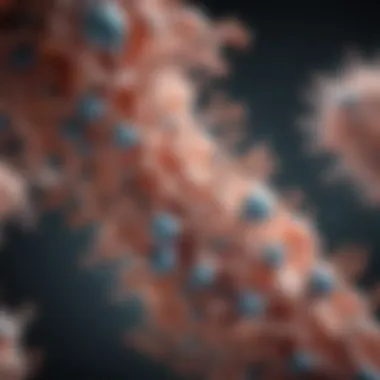
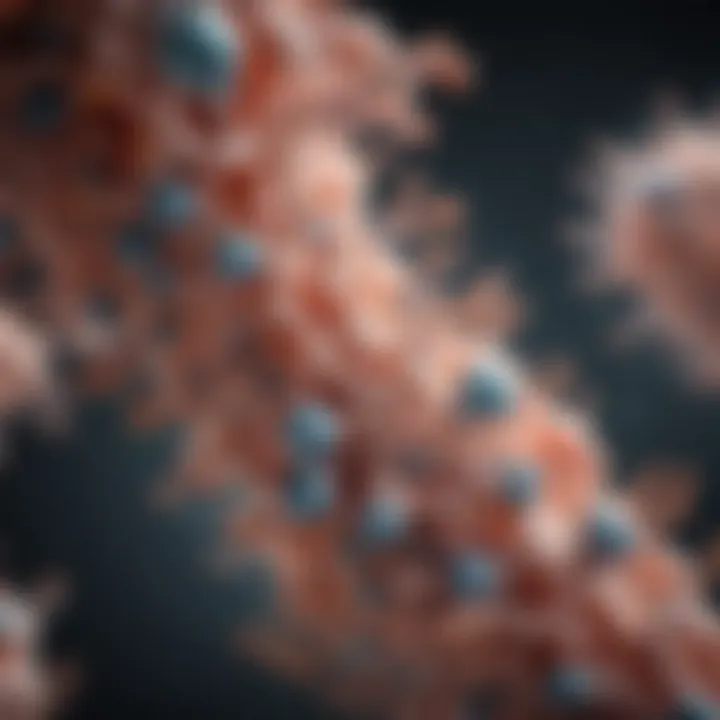
Intro
Proteins are fundamental biomolecules that play a crucial role in the functioning of all living organisms. They are not just mere structural components; they are involved in a multitude of biological processes that dictate life itself. This article seeks to demystify the complex world of proteins, providing a thorough understanding of their structure, function, and importance in health and disease.
Proteins are composed of amino acids, which are linked together in specific sequences to form unique three-dimensional structures. These structures determine their functionalities, allowing proteins to catalyze metabolic reactions, provide structural support, and regulate biological processes. Each protein has distinct characteristics and is tailored to fulfill specific roles within the cell.
Research shows that proteins can be categorized into various groups based on their structure and function. Enzymatic proteins, structural proteins, signaling proteins, and transport proteins are just a few examples. Understanding how these proteins interact with one another and with other biomolecules is vital for grasping their roles in cellular and organismal biology.
As we explore protein functionality, it is essential to consider not only their contribution to normal physiological processes but also their implications in diseases. From genetic disorders to infections, proteins are often at the forefront of biological dysfunction. Thus, knowledge of protein function has significant ramifications for medical research and therapeutic development.
This comprehensive exploration will guide readers through the intricacies of protein action, highlighting key insights and breakthroughs that have advanced our understanding of these essential molecules.
Intro to Protein Function
Proteins are fundamental components of all living organisms. Understanding their function is crucial for grasping the complexity of biological systems. This section serves to outline the major roles that proteins play, setting the stage for a deeper exploration of their characteristics and classifications.
Defining Proteins and Their Roles
In simplest terms, proteins are large, complex molecules made up of chains of amino acids. These amino acids are linked together by peptide bonds. The sequence of amino acids in a protein is determined by the corresponding gene. This sequence influences the protein's shape and, ultimately, its function.
Proteins can perform a wide range of functions within an organism. Some of the key roles include:
- Enzymatic activity: Proteins called enzymes catalyze biochemical reactions.
- Structural support: Proteins like collagen provide framework for cells.
- Transport and storage: Hemoglobin transports oxygen in blood.
- Regulation of biological processes: Proteins can regulate gene expression or cell cycles.
- Defense mechanisms: Antibodies are proteins that protect against pathogens.
This diversity of roles illustrates why understanding protein function is of utmost importance. Knowing how proteins operate can give insights into everything from metabolic pathways to disease mechanisms.
The Importance of Proteins in Biological Systems
Proteins are vital for maintaining the integrity of biological systems. They facilitate essential processes that sustain life. The significance of proteins includes:
- Catalyzing metabolic reactions: Without enzymes, many biochemical reactions would occur too slowly to sustain life.
- Maintaining structure: Proteins contribute to the architecture of cells and tissues. For example, keratin reinforces hair and nails.
- Facilitating communication: Proteins are involved in signal transduction, relaying information between cells. This is crucial for processes such as growth and immune responses.
"Proteins are not merely building blocks; they are dynamic participants in every aspect of cellular function."
In summary, proteins are essential in various biological processes. They enable life as we know it, influencing health and disease outcomes. By delving into the functional aspects of proteins, this article emphasizes the centrality of these molecules in biological research and applications.
Classification of Proteins
Protein classification offers a framework for understanding the diverse functionalities that proteins perform in biological systems. This classification is essential for researchers, students, and healthcare professionals alike. By categorizing proteins into distinct classes, one can analyze their roles, mechanisms of action, and implications in health and disease. This structured approach aids in the effective study and application of proteins in various scientific fields.
Enzymatic Proteins
Enzymatic proteins, or enzymes, are catalysts that accelerate chemical reactions crucial for life. They lower the activation energy required for reactions, thus speeding up biological processes without being consumed in the reaction. Enzymes are highly specific to their substrates, ensuring that each reaction proceeds with incredible efficiency. Examples include amylase, which breaks down starches into sugars, and DNA polymerase, which is involved in DNA replication. The study of enzymatic proteins is vital, as it allows scientists to understand metabolism and develop drugs targeting specific enzymes.
Structural Proteins
Structural proteins provide support and shape to cells and tissues. They form the framework of various biological structures, including muscles, skin, hair, and bones. Notable examples of structural proteins are collagen and keratin. Collagen is found in connective tissues and is crucial for skin elasticity and strength, while keratin is the main component of hair and nails. Understanding structural proteins helps in areas such as tissue engineering and regenerative medicine, where restoring or mimicking tissue structure becomes necessary.
Transport Proteins
Transport proteins are involved in the movement of molecules across cell membranes and throughout the body. They facilitate the transport of essential substances like oxygen, nutrients, and ions. Hemoglobin, for instance, is a transport protein that carries oxygen in red blood cells. Other examples include albumin, which transports fatty acids, and membrane transport proteins like glucose transporters. The study of transport proteins is crucial for understanding cellular communication and nutrient absorption, which are fundamental to maintaining homeostasis.
Regulatory Proteins
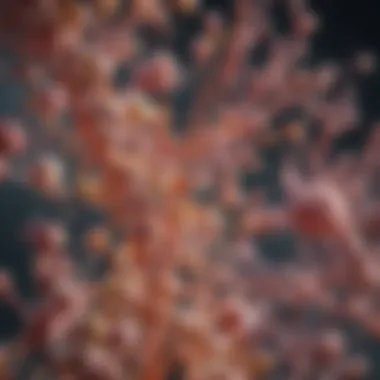
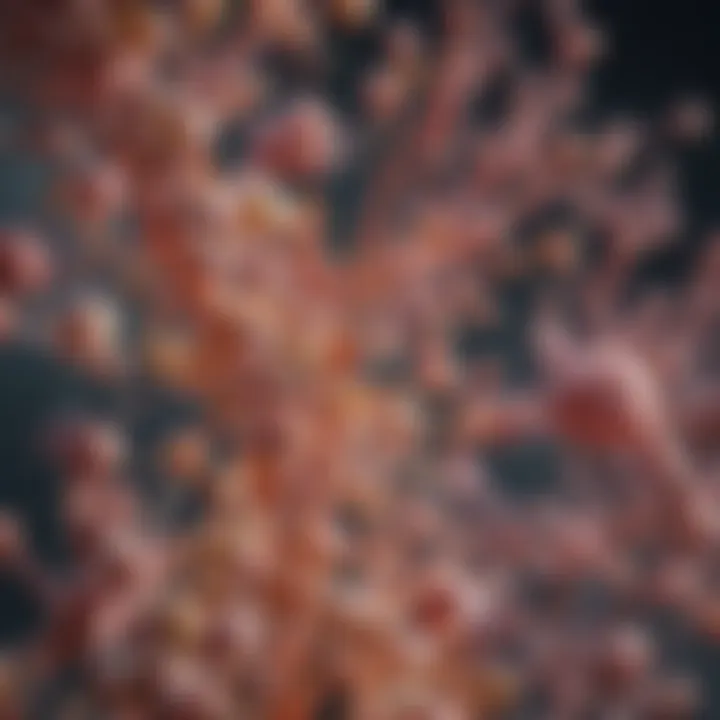
Regulatory proteins control various cellular processes, ensuring proper function and response to changes in the environment. They include transcription factors that regulate gene expression and enzymes that modulate metabolic pathways. These proteins act as molecular switches, either activating or inhibiting specific biological functions. For example, insulin is a regulatory protein that helps control blood glucose levels. Understanding regulatory proteins can lead to advancements in therapies for diseases related to dysregulation, such as diabetes and cancer.
Defensive Proteins
Defensive proteins play a critical role in the immune response, protecting the body against pathogens. Antibodies are a prominent example, recognizing and neutralizing foreign invaders like bacteria and viruses. Other defensive proteins include antimicrobial peptides and clotting factors that prevent blood loss. Studying these proteins leads to insights into immune responses and the development of vaccines, which are vital for public health. As pathogens evolve, understanding defensive proteins becomes increasingly important in designing effective therapies and interventions.
The classification of proteins not only enhances our understanding of biological systems but also contributes significantly to medical research and therapeutic advancements.
Protein Structure and Function
Understanding the relationship between protein structure and function is essential for comprehending how proteins operate within biological systems. The structure of a protein often dictates its functionality. This section explores the different levels of protein architecture and how they contribute to the overall roles of proteins in metabolism, signaling, and biological pathways. Each structural level brings its own unique attributes that are crucial for protein activity.
Levels of Protein Structure
Proteins have four distinct levels of structure, each playing a pivotal role in defining their properties and functions.
Primary Structure
The primary structure of a protein refers to its linear sequence of amino acids. This sequence determines the unique identity of a protein and establishes the foundation for higher levels of structure. A key characteristic of primary structure is its specificity. Each protein's unique sequence leads to different functions within an organism.
One beneficial aspect of primary structure is that it directly influences how the protein will fold and function. However, if the amino acid sequence is altered due to mutations, it can lead to severe consequences, impacting health and function. Mutations in the primary structure can create misfolded proteins, leading to diseases such as sickle cell anemia. Thus, understanding primary structure is crucial for insights into how proteins work and their role in health and disease.
Secondary Structure
Secondary structure refers to local folded structures that form within a protein due to hydrogen bonding. The two most common types are alpha helices and beta sheets. This structure contributes to the overall mechanical properties of proteins while maintaining their functionality.
A significant characteristic of secondary structure is that it contributes to the protein's stability. This stability is important in supporting the protein's three-dimensional form which is essential for its function. The unique feature here is that these local structures can influence the protein's flexibility and interaction with other molecules. However, relying too heavily on secondary structures can sometimes limit a protein's dynamic range, potentially affecting how it interacts within biological systems.
Tertiary Structure
The tertiary structure represents the complete three-dimensional arrangement of a protein. It involves the folding and packing of secondary structures into a more complex form. A key characteristic of tertiary structure is that it allows for a greater variety of interactions, including hydrophobic and ionic interactions, which significantly enhance the protein’s overall function.
The unique feature of this level is that it determines the functional sites on the protein. These sites are often critical for enzyme activity and binding interactions. One disadvantage, however, is that the stability of tertiary structures can be adversely affected by changes in environmental conditions, such as pH and temperature. Such changes can lead to denaturation, resulting in the loss of function.
Quaternary Structure
Quaternary structure involves the assembly of multiple polypeptide chains into a functional protein complex. This structure is crucial for proteins that require multiple subunits to carry out their function, such as hemoglobin.
A key characteristic of quaternary structure is its collaborative nature. Individual subunits can exhibit regulatory mechanisms that control the activity of the entire protein complex. The unique aspect here is that the interactions between the different subunits can lead to allosteric regulation, enhancing or inhibiting activity. However, this complexity can also pose challenges, as changes in one subunit can affect the entire protein complex.
Structure-Function Relationship
The relationship between a protein's structure and its function is foundational to biochemistry and molecular biology. Changes in structure can profoundly affect the functions that proteins perform within living organisms. For example, enzymes, which are proteins that catalyze biochemical reactions, rely heavily on their unique three-dimensional shapes to interact specifically with substrates. This specificity results from their structural features, highlighting the critical nature of structure in defining protein function.
"The structure of proteins determines their function and how they interact with other molecules, making it a critical aspect of biological research."
Understanding these structural levels can provide insights into protein design and evolution, thus informing research across numerous fields, including health and disease studies.
Mechanisms of Protein Action
The mechanisms by which proteins act are fundamental to understanding their diverse biological functions. Proteins do not merely exist in isolation; instead, they interact with various molecules, which is crucial for cellular activity and overall biological processes. Unraveling these mechanisms can provide insights into how life functions at a molecular level. This section will investigate three essential mechanisms: enzyme catalysis, molecular recognition, and signal transduction.
Enzyme Catalysis
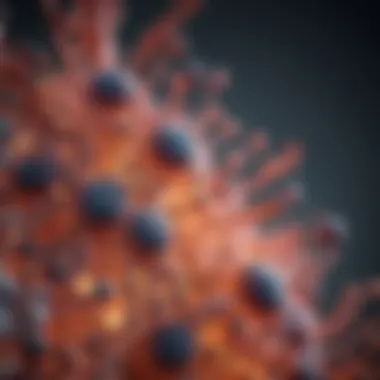
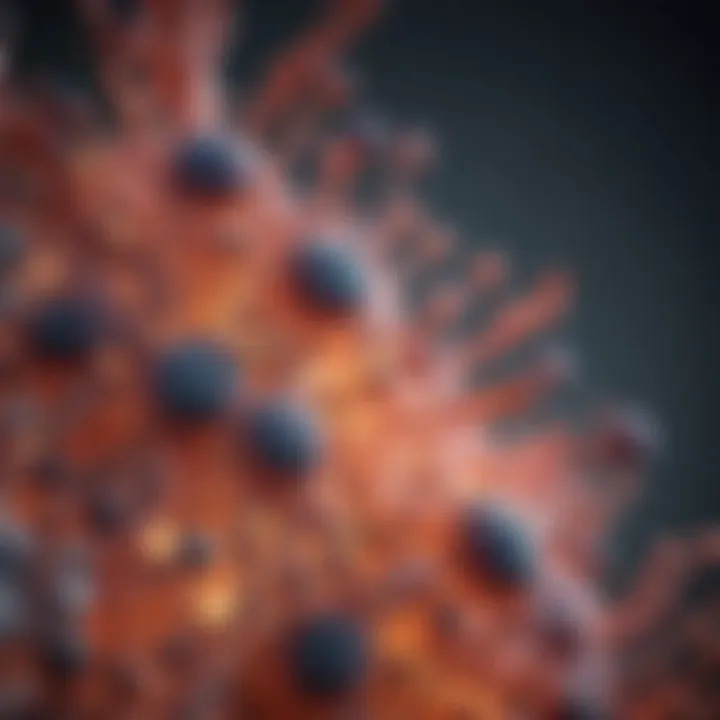
Enzymes are proteins that accelerate chemical reactions in organisms. They do this by lowering the activation energy required for a reaction to occur. The active site of an enzyme binds to substrate molecules, undergoing a conformational change that brings substrates into proximity and optimally orients them for a reaction.
- Specificity: Enzymes are highly specific for their substrates, which means they only catalyze certain reactions. This selectivity is key in metabolic pathways.
- Cofactors and Coenzymes: Many enzymes require additional non-protein molecules, such as cofactors or coenzymes, to function. These molecules can assist in substrate binding or in the actual chemical transformation.
- Regulation: The activity of enzymes can be regulated by various factors, including inhibitors and activators. This regulation is crucial for maintaining homeostasis within cells.
Molecular Recognition
Molecular recognition refers to the specific interactions between proteins and other biomolecules. These interactions are often governed by the shape, charge, and hydrophobic properties of the molecules involved.
- Receptor-Ligand Binding: Proteins can act as receptors on cell surfaces, binding to signaling molecules (ligands) and initiating a response within the cell.
- Antibody-Antigen Interaction: Immunoglobulins, or antibodies, specifically recognize and bind to antigens, facilitating immune responses. This selective binding highlights the precision of molecular recognition.
- Protein-Protein Interactions: Many cellular processes depend on proteins binding to one another. For instance, signaling pathways often involve kinases that recognize and phosphorylate target proteins.
Signal Transduction
Signal transduction is the process through which cells respond to external signals. It involves a cascade of molecular interactions that convert a signal into a functional response.
- Pathway Components: Signaling often begins at a cell surface receptor, which can detect hormones, neurotransmitters, or environmental stimuli. Upon binding of the signal, a series of intracellular proteins get activated in a specific sequence.
- Amplification: Signal transduction pathways can amplify the signal, leading to a significant cellular response from a small number of input signals. This amplification is critical for effective cellular communication.
- Outcome: Ultimately, the response to a signal can alter cell behavior, such as changes in gene expression, metabolism, or cell division.
Understanding these mechanisms of protein action enriches our perception of biological processes and is critical for advances in fields like biotechnology, medicine, and molecular biology.
Proteins and Cellular Processes
Proteins are indispensable to numerous cellular processes. Their functions span a wide range, influencing metabolism, cell division, and the maintenance of cellular integrity. Understanding how proteins facilitate these processes is paramount for grasping their role in health and disease.
Cellular Metabolism
Cellular metabolism involves the chemical reactions that sustain life. Proteins serve as enzymes, catalyzing these necessary biochemical reactions. Without enzymatic action, metabolic pathways would be inefficient and ineffective. For instance, enzymes like amylase break down carbohydrates into simple sugars, which cells then use for energy.
Moreover, proteins regulate metabolic pathways by interacting with substrates and co-factors. This interaction ensures that metabolic processes are performed smoothly, adapting to cellular needs. Notably, enzymes can be modulated by various signals, this responsiveness plays a vital role in cellular homeostasis.
Key Points about Cellular Metabolism and Proteins:
- Enzymes accelerate metabolic reactions, crucial for energy production.
- They provide regulation through feedback mechanisms to maintain balance within cells.
- Proteins help adjust metabolic pathways in response to environmental changes.
Cell Cycle Regulation
The orchestration of the cell cycle depends heavily on proteins that control progression through various phases. Cyclins and cyclin-dependent kinases (CDKs) are chief among these. They ensure that cells proceed through the cell cycle in a timely and orderly fashion. If these proteins fail, it may result in uncontrolled cell division, possibly leading to cancer.
Regulatory proteins also act as checkpoints, preventing the cell from advancing to the next phase until certain conditions are met. This system is critical for maintaining genetic stability and ensuring proper cell function. The failure of these regulatory mechanisms highlights the importance of proteins in maintaining cellular integrity.
Considerations regarding Cell Cycle Regulation:
- Precision is key. Errors in protein function can lead to severe consequences, including tumorigenesis.
- Interactions matter. CDKs interact with numerous proteins to regulate the cycle, emphasizing the complexity of cellular control.
Protein Folding and Misfolding
Protein folding is an essential process that enables proteins to achieve their functional shape. This process is mediated by chaperone proteins, which assist in achieving the correct conformation. Misfolding can occur, leading to loss of function or even toxic aggregates. Diseases such as Alzheimer's and Parkinson's illustrate the ramifications of improper protein folding.
Moreover, understanding protein folding pathways provides insights into potential therapeutic strategies. Interventions that enhance proper folding or degrade misfolded proteins could mitigate the impact of related diseases.
Highlights of Protein Folding:
- It is a key determinant of protein functionality.
- Misfolded proteins are implicated in various neurodegenerative diseases.
- Therapeutic approaches focus on correcting or managing misfolded proteins through multiple strategies.
"Proteins are critical players in cellular processes, influencing everything from metabolism to disease outcomes."
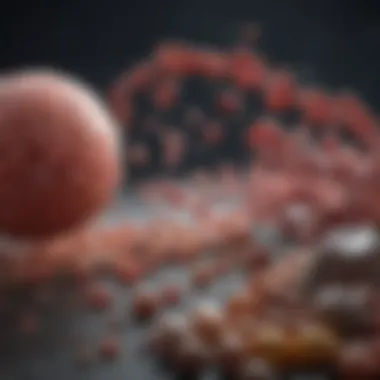
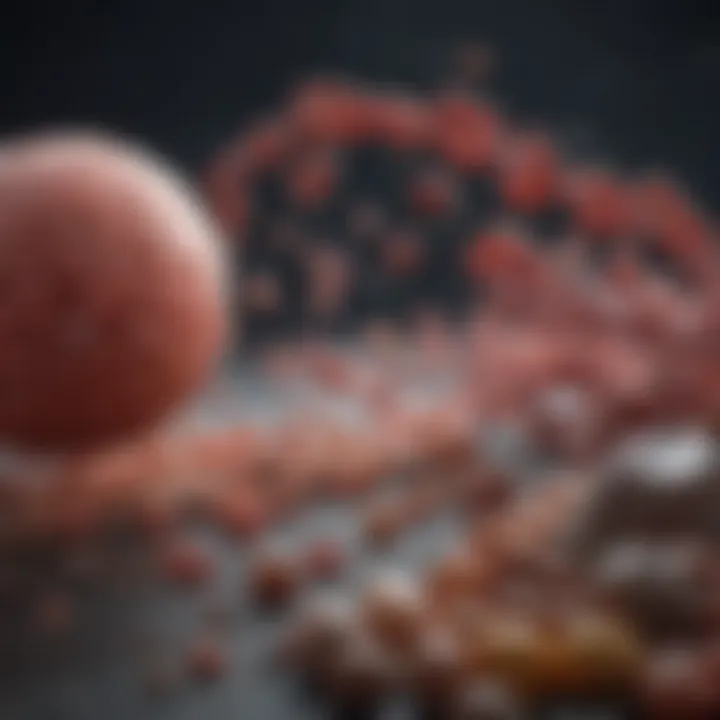
By grasping these cellular processes, one can appreciate the profound impact of proteins in both health optimisation and disease prevention. Understanding the role of proteins is invaluable for researchers and professionals aiming to unravel the complexities of cellular biology.
Proteins in Health and Disease
Proteins serve as essential components in biological systems, influencing numerous processes that govern health and disease. The relevance of this topic in the broader context of biological research cannot be overstated. Understanding how proteins function can lead to insights into various health conditions, providing pathways for diagnosis and treatment.
Role of Proteins in Disease Pathogenesis
Proteins play critical roles in disease development. Many diseases, especially those of genetic nature, are caused by malfunctions in protein structure or function. For instance, cystic fibrosis results from a defect in the CFTR protein, disrupting ion transport across cell membranes. Similarly, mutations in the BRCA1 and BRCA2 proteins are linked to an increased risk of breast and ovarian cancer.
Moreover, various pathogens employ proteins that alter host cellular functions, promoting disease. For example, several viral proteins interfere with the host’s immune responses, allowing the virus to persist and replicate. Understanding how these proteins contribute to pathogenesis can inform therapeutic interventions, guiding research towards protein-targeted drugs or vaccines.
Biomarkers and Therapeutic Targets
The use of proteins as biomarkers has greatly enhanced the ability to diagnose diseases at earlier stages. Specific proteins can indicate disease presence or progression, allowing for timely medical interventions. For instance, prostate-specific antigen (PSA) levels serve as a marker for prostate cancer, while the presence of amyloid-beta proteins is significant for diagnosing Alzheimer's disease.
Additionally, therapeutic strategies increasingly target proteins implicated in disease. Monoclonal antibodies, such as trastuzumab for HER2-positive breast cancer, illustrate how specifically targeting proteins can offer effective treatments. By inhibiting or enhancing protein functions, these therapies can leverage the body’s biological pathways for improved health outcomes.
Understanding the role of proteins in health and disease is pivotal not only for research but also for the development of effective treatments and diagnostic tools.
Recent Advances in Protein Research
Recent advances in protein research have significantly changed our understanding of protein function. Proteins are key players in nearly every cellular process. As technology improves, researchers can analyze proteins on a more detailed level than before. This includes studying the structure, function, and interactions of proteins within various biological contexts. The implications of these advancements are considerable, providing insights that can lead to new medical therapies and enhanced biotechnological applications.
The growth of proteomics, the large-scale study of proteins, is central to these advancements. Through sophisticated techniques, scientists can examine protein expression, modification, and interaction networks. The ability to profile proteins in different conditions enables researchers to understand how they contribute to health and disease.
In addition, bioinformatics has emerged as a crucial tool for analyzing the vast amount of data generated by proteomic studies. This field harnesses computational methods to predict protein structures, interactions, and functions based on sequence data. By integrating biological information with advanced algorithms, bioinformatics aids in the identification of potential biomarkers and therapeutic targets.
Collectively, these advances in protein research hold great promise for fields as diverse as medicine, biotechnology, and environmental science. Understanding these developments is not only interesting but vital for advancing scientific knowledge and practical applications.
Proteomics and Its Applications
Proteomics has burgeoned into a critical field that enables scientists to explore the dynamic changes in protein expression and activity across different biological contexts. By studying proteomes, researchers can evaluate how proteins function in various physiological states and how they contribute to disease processes. Some applications of proteomics include:
- Disease Diagnosis: Proteomics can help identify specific protein markers associated with diseases like cancer or neurodegenerative disorders. This leads to earlier diagnosis and personalized treatment strategies.
- Drug Development: By understanding the interactions between proteins and potential drug compounds, scientists can design more effective medications. Targeting specific proteins can enhance efficacy while minimizing side effects.
- Biomarker Discovery: Proteomic profiling aids in discovering biomarkers that help in monitoring disease progression and treatment response.
Furthermore, proteomics also plays a role in understanding protein modifications like phosphorylation or glycosylation. These modifications can affect protein function, impacting signaling pathways and cellular responses.
Bioinformatics in Protein Study
Bioinformatics serves as a backbone for modern protein research. It involves the use of computational tools and methods to manage and analyze biological data. In protein studies, bioinformatics helps in:
- Sequence Analysis: Understanding the amino acid sequence of proteins is crucial for predicting their structure and function. Tools can identify homologous proteins across different species, shedding light on evolutionary relationships.
- Structural Prediction: Many bioinformatics platforms use algorithms to model protein structures based on sequences. This saves time and resources that would otherwise be spent on experimental methods.
- Interaction Networks: Bioinformatics also helps map out protein-protein interactions, allowing researchers to visualize and understand complex signaling pathways and cellular networks.
Closure
The conclusion of this article emphasizes the fundamental positioning of proteins within the biological framework of living organisms. Proteins are not simply byproducts of gene expression; they are pivotal components of cellular machinery and play critical roles in various physiological processes. Understanding the functional diversity of proteins contributes greatly to biochemistry and molecular biology.
The Future of Protein Research
As we look ahead, the future of protein research is promising and full of potential. Innovations in proteomics are enabling researchers to analyze the complex interactions and functions of proteins on an unprecedented scale. These developments can lead to breakthroughs in understanding diseases at the molecular level and designing targeted therapies.
Key Areas for Future Exploration:
- Novel Protein Functions: Identifying new roles for previously understood proteins can reshape our approach to biological processes.
- Protein Engineering: Enhancements through synthetic biology may create proteins with novel functionalities, offering therapeutic solutions.
- Systems Biology Integration: Studying proteins in the broader context of cellular networks can give insight into disease mechanisms.
Moreover, the integration of bioinformatics tools will enhance our ability to model and predict protein behavior under various conditions, aiding in drug discovery and development. As we advance our technical capabilities, collaborations between biologists, chemists, and computer scientists will be pivotal.
"A deeper understanding of protein functions engages multiple disciplines, highlighting the interconnectivity in biomedical research."
In summary, continuous advancement in protein research holds promises not only for the scientific community but also for health outcomes globally. By harnessing emerging technologies, researchers can further unravel the complexities of proteins, making them central to future discoveries in medicine and biotechnology.