Understanding Surface Tension and Contact Angle
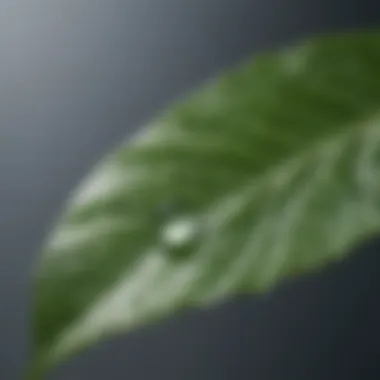
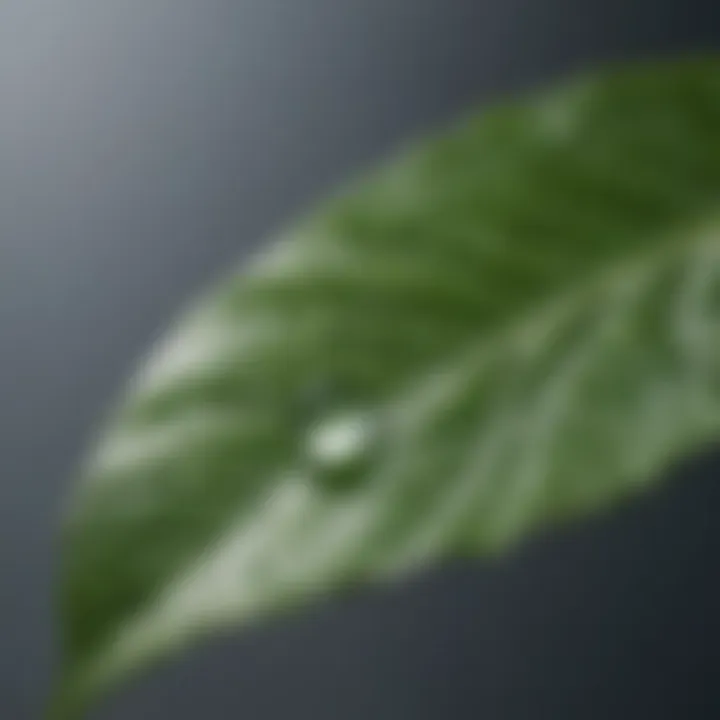
Intro
Surface tension and contact angle are not just scientific terms thrown around in classrooms or textbooks; they represent fundamental concepts in understanding fluid dynamics and material interactions. Picture a raindrop resting on a leaf, that perfectly formed bead not spilling over speaks volumes about the forces at play. These forces are driven by the interactions at the liquid-gas and solid-liquid interfaces. In both nature and engineered environments, grasping the methods behind surface tension and contact angle becomes paramount for fields ranging from material science to nanotechnology and biomedical engineering.
Understanding surface tension means peeling back layers of physics that dictate how liquids behave, while the contact angle offers a glimpse into how surfaces interact with those liquids. The interplay between these two phenomena reveals critical insights not only into natural occurrences, like the behavior of water on different surfaces, but also into applications in industries such as coatings, adhesives, and even in biological systems. This article will take a deep dive into the theory, experimental techniques, and practical implications of these concepts, showcasing why they matter in a variety of contexts.
Research Highlights
Key Findings
- Surface tension is primarily the result of cohesive forces among liquid molecules, which minimizes the surface area of the liquid and dictates its shape at rest.
- The contact angle is a measure of how a liquid droplet interacts with a solid surface, determining whether a surface is hydrophilic or hydrophobic.
- Experimental studies have shown that variations in surface texture and chemical composition can significantly alter the contact angle, thereby influencing wettability.
"The angle formed between the tangent to the liquid surface at the contact point and the solid surface is crucial in determining the liquid’s affinity for the solid material."
Implications and Applications
- Understanding surface tension has implications in designing more efficient detergents and surfactants, which lower the surface tension of water to improve wetting and cleaning.
- Industries leveraged knowledge of contact angles for creating high-performance coatings that repel water and dirt, enhancing surface longevity.
- In biomedicine, the principles of surface tension and contact angle can inform the development of implants with optimal tissue compatibility, improving healing rates and reducing rejection risks.
Methodology Overview
Research Design
The study of surface tension and contact angle often relies on both theoretical and empirical approaches. Theoretical models are developed to predict behaviors based on molecular dynamics, while experimental setups are crucial for validation. Experiments may involve drop shape analysis, where the curvature of a droplet is directly measured to derive contact angles against various surfaces.
Experimental Procedures
To explore these concepts practically, various methodologies may be employed, including:
- Wilhelmy Plate Method: A thin plate made of a material is partially immersed in a liquid, and the force acting on the plate is measured to derive surface tension values.
- Sessile Drop Method: This involves placing a liquid drop on a solid surface and using imaging techniques to capture the profile for analysis of contact angle.
- Spin Coating: A technique used to apply thin films to substrates, facilitating the study of how surface texture affects wettability.
Through a detailed exploration of both experimental and theoretical aspects, the nuances of these interfacial phenomena can be revealed. The interplay of surface tension and contact angle not only enhances our understanding of liquids and solids but also propels innovation across various scientific and industrial fields.
Prolusion to Surface Tension and Contact Angle
In the realm of fluid dynamics and material science, surface tension and contact angle emerge as pivotal concepts. These phenomena are not just theoretical constructs; they have profound implications in both natural processes and engineered systems. Understanding these principles is essential for grasping how liquids interact with one another and with solid surfaces beneath them. This article aims to shine a light on these fundamental ideas, exploring their significance across various fields such as biology, chemistry, and even engineering.
Surface tension can be observed in everyday life. For instance, when water forms droplets on a leaf, it is a direct display of surface tension at work. Similarly, contact angle is the angle formed at the interface of a liquid with a solid surface. This angle serves as a crucial indicator of how well a liquid wets a surface, impacting everything from paint adhesion to the efficiency of coatings in manufacturing processes.
By delving into these concepts, readers will gain valuable insights that extend far beyond academic knowledge. The material covered will highlight the importance of these interactions in diverse applications—from improving cleaning products to enhancing inkjet printing technologies. Understanding these ideas can also pave the way for innovative solutions in nanotechnology and microfluidics. Hence, the significance of surface tension and contact angle cannot be overstated, as these principles connect fluid behavior to a multitude of industrial and natural processes.
Defining Surface Tension
Surface tension can be defined as the force that acts on the surface of a liquid, causing it to behave like a stretched elastic membrane. At an atomic level, this phenomenon arises due to cohesive forces between molecules. In simple terms, molecules at the surface experience a different environment than those in the bulk of the liquid. While molecules within the liquid are pulled equally in all directions, those on the surface are subject to net inward forces, which results in surface tension.
This concept is often quantified in units of force per unit length, commonly expressed in dynes per centimeter or newtons per meter. A high surface tension indicates a strong cohesive force among molecules, as seen in mercury, while lower surface tension can be observed in soapy water.
Moreover, the effects of surface tension are observable in many contexts. For example, the ability of small insects to walk on water without sinking is a direct consequence of surface tension. Surface tension effects are also pivotal in processes such as emulsification, where two immiscible liquids are combined, as well as in droplet formation during spraying.
Understanding Contact Angle
The contact angle is a measure of how a liquid interacts with a solid surface. It is formed at the three-phase boundary where the solid, liquid, and vapor meet. This angle provides insight into the wettability of a surface, which is crucial for applications like coating, printing, and cleaning processes.
A low contact angle, typically below 90 degrees, means that the liquid spreads on the surface, indicating good wettability. Conversely, a high contact angle, greater than 90 degrees, signifies that the liquid beads up, reflecting poor wettability. Consider how water forms beads on a waxy surface, as opposed to how it spreads out on a clean glass surface. The differences in contact angles can be attributed to the chemical properties of the surfaces involved.
Understanding these angles can help researchers and engineers tailor materials for specific applications—like designing self-cleaning surfaces or optimizing ink formulations for print quality. The intimate relationship between surface tension and contact angle opens doors to advancements in various technological domains, reinforcing the need for a detailed exploration of these phenomena.
Physical Principles of Surface Tension
Understanding the physical principles of surface tension is crucial for grasping its implications in both theoretical and practical contexts. Surface tension manifests as the cohesive forces between liquid molecules, especially at their interfaces. These cohesive forces result in behaviors that can sometimes baffle the mind. The examination of surface tension paves the way for insights about fluid behavior, stability of emulsions, and even the dynamics of raindrops clinging to leaves.
Molecular Interaction at Interfaces
In the world of liquid interfaces, molecular interaction is akin to an elegant dance. Molecules at the liquid's surface experience a different environment than those within the bulk phase. While those burrowed deeper enjoy the comfort of mutual attraction, the surface molecules are tugged along by their neighbors while also facing an expanse of air or another phase. This disparity is what creates surface tension, often quantified in units of force per unit length, such as dyne per centimeter.
Surface tension is not merely an abstract concept; it has tangible impacts on everyday occurrences. Consider water droplets perched on a freshly waxed car hood—they form near-perfect spheres instead of flattening out. This is due to the cohesive forces at play, which are stronger than the adhesive forces between the water and the waxy surface.
"The phenomenon of surface tension, while often unnoticed, orchestrates the behaviors of many natural processes, from insect locomotion across liquid surfaces to the formation of clouds high in the sky."
To illustrate, let’s take a closer look at how the phenomenon aids in life. Small creatures, like water striders, exploit surface tension to skim across water, thanks to their specially adapted legs that distribute their weight more than a drop of rain would. This reflects not only the principles of physics at work but also the marvels of evolution.
Energy Considerations in Surface Dynamics
Energy plays a critical role in surface tension dynamics, as it directly ties into how surfaces interact and behaves. When a liquid droplet is formed, energy is exerted to create that surface area. This energy investment is evident in the concept of surface energy, which can be thought of as the excess energy at the surface of a material compared to the bulk.
To maintain a small droplet shape, the surface tension poses a significant barrier. Thus, whenever droplets merge—be it during rain showers or in inkjet printing processes—energy is released, leading to a decrease in surface area, thus stabilizing the merged droplet.
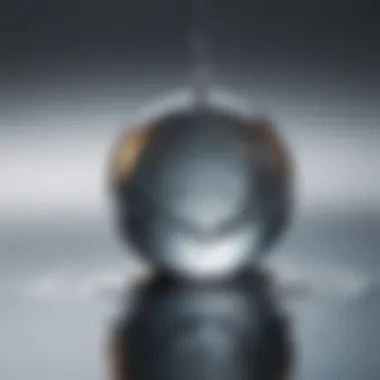
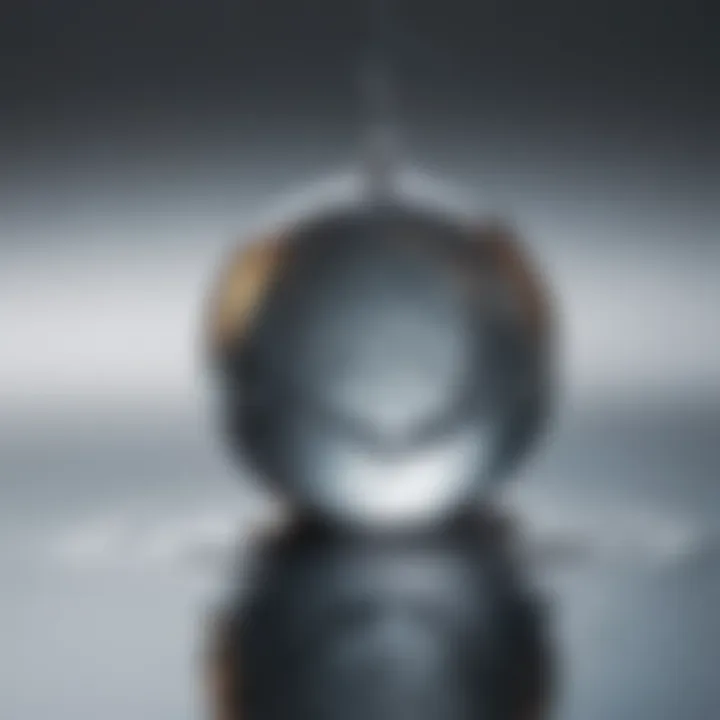
Also worth noting is that surface tension is influenced by temperature. As heat enters the equation, molecules gain kinetic energy, leading to more chaotic molecular motion and potentially disrupting cohesive interactions. This is evident in scenarios where boiling occurs, and surface tension decreases, allowing bubbles to rise and eventually escape.
Measurement Techniques for Surface Tension
Understanding how to accurately measure surface tension is crucial in both academic research and industrial applications. This section emphasizes the significance of various measurement techniques, spotlighting their mechanisms, advantages, and limitations. Each method carries unique insights into surface behavior, thereby giving researchers and professionals the tools to analyze the physical properties of liquids effectively.
Surface tension is not merely a theoretical concept; its measurement has implications across numerous fields, from chemistry to biological systems. Furthermore, different contexts demand different methods; hence, selecting a suitable technique can dramatically affect the results and interpretations of experiments.
Du Noüy Ring Method
The Du Noüy Ring Method is widely recognized for its accuracy and reliability in measuring surface tension. With a simple yet effective design, it employs a wire ring to determine the force required to detach a ring from the liquid surface. The fundamental principle here is that as the ring is pulled upward, it experiences an upward force due to surface tension.
- Procedure Overview: A thin platinum ring is submerged to a known depth in the liquid. As it rises, the surface tension acts against the force pulling it up. The maximum force before detachment serves as a measure of the surface tension.
- Advantages: This approach is particularly appreciated for its ease of use and strong repeatability. It can be adapted to a variety of liquids, including those with impurities or a range of viscosities.
- Limitations: However, factors like ring cleaning and the effects of any volatile components in the liquid can introduce errors in measurement. Careful calibration and consistency with the method are key to accurate results.
Wilhelmy Plate Method
The Wilhelmy Plate Method is another traditional technique employed to evaluate surface tension. It involves a thin vertical plate that is partially immersed in the liquid. The weight of the plate and the forces acting on it are balanced to derive the surface tension value.
- Principle of Operation: The force exerted on the plate, due to surface tension, can be expressed as:[\gamma = \fracFL ]where (F) denotes the force, and (L) is the width of the plate.
- Advantages: This method offers a quick reading and is adaptable to various liquid types, including very low surface tension liquids.
- Limitations: A downside is that it can be sensitive to changes in liquid height and may not suit all experimental setups due to its dependence on precise measurements of weight.
Pendant Drop Technique
The Pendant Drop Technique measures surface tension by evaluating the shape of a droplet suspended from a syringe or nozzle. It's particularly useful when studying liquids that exhibit high volatility or those that form unique droplet shapes under different conditions.
- Methodology: By analyzing the profile of the droplet, researchers can derive surface tension values based on the Young-Laplace equation, which relates pressure difference across a curved surface to the surface tension:[\Delta P = 2\gamma/R]where (\Delta P) is the pressure difference and (R) is the droplet's radius of curvature.
- Advantages: The flexibility of use in different settings and conditions is a highlight of this technique. It allows for studying complex liquid behaviors under varied environmental conditions.
- Limitations: However, it's worth mentioning that the droplet shape can be influenced by external factors such as vibrations or airflow, leading to potential inaccuracies.
In summary, each measurement technique for surface tension has its own set of strengths and weaknesses. The choice of method largely depends on the specific requirements of the study at hand. Selecting a method that aligns best with your research context can ensure more precise and meaningful outcomes in the study of fluid dynamics.
Contact Angle Measurement Methods
Understanding how liquids interact with solid surfaces is essential in lab and industrial settings. This section about contact angle measurement methods provides an insight into these interactions. These methods are crucial for determining wettability, which can influence everything from paint adhesion to how well a liquid spreads on a surface. Knowing the contact angle helps professionals predict product performance and tailor surface properties to meet specific needs.
Sessile Drop Method
The sessile drop method is one of the most commonly used techniques for measuring contact angles. The principle behind it is rather straightforward: a drop of liquid is placed on a solid surface, and the contact angle is measured at the three-phase boundary where the solid, liquid, and vapor phases meet. This method offers a few advantages, especially its simplicity and general applicability for different solid surfaces.
In practical terms, the measurement can be done using a goniometer, which captures images of the droplet. Using image analysis software, one can accurately determine the contact angle by analyzing the profile of the drop. However, it’s important to ensure that the droplet remains static during the measurement to avoid any misleading results.
Several factors can affect the accuracy of this method:
- Surface cleanliness: Any contaminants on the surface can skew the results, leading to inaccurate contact angle readings.
- Drop volume: If the droplet is too small, the measurement might not capture the typical surface interactions. Conversely, too large a droplet may cause spreading, which complicates the reading.
While the sessile drop method is highly effective, it is worth noting that the environment can also play a significant role. Humidity and temperature fluctuations can affect the liquid's behavior and, thus, the contact angle. Overall, the sessile drop method remains an important touchstone in contact angle measurements, providing reliable data when executed with care.
Dynamic Contact Angle Measurement
Dynamic contact angle measurement provides additional depth to understanding wettability by assessing how contact angles change with motion. This approach is particularly useful in scenarios where surface interactions are not static and can be influenced by various external factors. There are two main sub-methods to consider: advancing and receding contact angles.
The advancing contact angle is measured as the droplet is added, while the receding contact angle is observed when the droplet retracts from the surface. By analyzing both angles, one can obtain a more dynamic view of surface behavior.
This measurement technique is vital in fields like coatings, where the performance during application can differ from what static measurements might suggest. Thus, it helps in understanding how a liquid wets a surface during actual usage scenarios.
Simple setups allow researchers to gain valuable insights:
- Surface composition: Different materials can yield varying advancing and receding angles, illustrating surface heterogeneity.
- Liquid properties: Changes in viscosity and surface tension can directly influence how a liquid behaves when interacting with a given surface.
Measuring dynamic contact angles can be a telling window into real-world applications and phenomena, validating theoretical frameworks and leading to better product formulations. By marrying both methods of static and dynamic measurements, researchers and engineers can achieve a fuller perspective on wettability and surface interactions.
"Understanding contact angles is not just an academic exercise; it has real implications in product design and efficiency."
Integrating these methodologies helps professionals navigate the complex interplay between liquids and solids, leading to innovations across many industries.
Factors Affecting Surface Tension
Understanding the factors affecting surface tension is essential for grasping the full implications of fluid behavior in various contexts, whether natural, industrial, or experimental. When surface tension is dissected, it becomes clear that several elements contribute to its variations, impacting everything from how droplets form to how bubbles behave. This section sheds light on these determinants with a focus on temperature dependence, impurities and surfactants, and the influences of pressure and volume.
Temperature Dependence
Surface tension is not a static property; rather it fluctuates with changes in temperature. As the temperature increases, the kinetic energy of molecules in a liquid rises. This increase in energy can disrupt the cohesive forces that contribute to surface tension. Consequently, one can observe a marked decrease in surface tension as the temperature climbs. For instance, water at 20°C exhibits a surface tension of about 72.8 mN/m, whereas at 100°C, this value drops to around 58.9 mN/m.
- Critical Temperature Influence: The phenomenon of temperature affecting surface tension is particularly significant during processes such as boiling or condensation. At critical temperatures, liquids begin to lose their distinctive boundaries, which directly impacts how they interact with solids.
- Practical Implications: Recognizing how temperature affects surface tension is crucial in fields like meteorology, where raindrop formation is dependent on alterations in temperature, and in industries such as plastics manufacturing, influencing the behavior of materials during processing.
Impurities and Surfactants
The presence of impurities and surfactants is another major player in determining surface tension. Surfactants can dramatically lower surface tension by aligning themselves at the liquid's surface, effectively disturbing the cohesive forces at play.
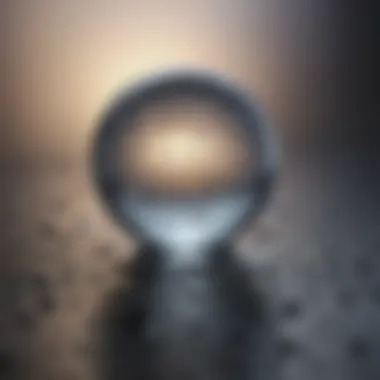
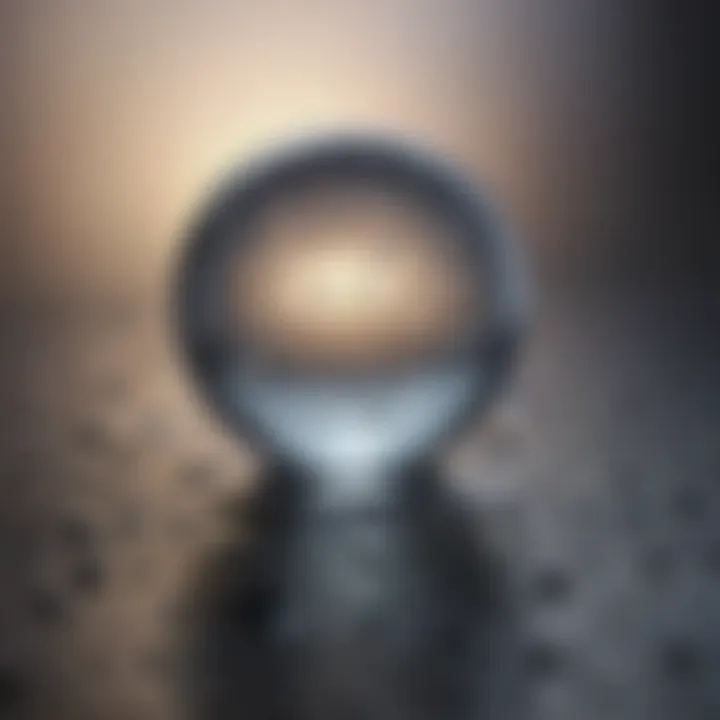
- Role of Surfactants: For instance, the incorporation of soap into water can reduce its surface tension significantly, allowing bubbles to form more easily. This property is exploited in household cleaning products and various industrial applications, like oil recovery and paint formulations.
- Environmental Interaction: Impurities can come from a wide range of sources, including environmental pollutants and additives in industrial processes, thus complicating surface interactions in water bodies or any liquid system.
Important Note: The understanding of surfactants and impurities is crucial for fields that rely on liquid behavior, such as environmental science, because they can alter how pollutants behave in water.
Effects of Pressure and Volume
Surface tension is also influenced by changes in pressure and the volume of liquid systems. While pressure increments have a notable effect on gases, liquids are generally incompressible. However, under specific conditions, such as in confined spaces or microfluidics, the alterations in pressure can have significant implications for surface tension.
- High Pressure Impacts: At elevated pressures, liquid molecules are forced closer together, which might, under certain circumstances, affect surface tension positively or negatively depending on the liquid’s nature. For example, in high-pressure systems used in petroleum extraction, surfactant efficiency variations may be consequential.
- Volume Considerations: The volume of liquid can also shape surface tension behaviors. Smaller volumes, analogous to droplets, generally exhibit higher surface tension relative to larger bodies of liquid, stemming from the ratio of surface area to volume being significantly higher in small volumes.
Overall, grasping how temperature, impurities, and pressure influence surface tension not only enriches the theoretical understanding but also has practical ramifications across scientific and industrial applications.
Influence of Surface Roughness on Contact Angle
The interaction between surfaces and liquids is markedly influenced by the surface roughness of materials. It's no small matter; understanding this influence is key to multiple fields, from material science to engineering. As a liquid droplet comes into contact with a surface, its shape can tell you a lot about the surface's characteristics. The contact angle, the angle formed between the tangent to the liquid surface and the solid surface at the three-phase boundary, varies due to changes in roughness. Therefore, the way a surface is engineered—be it intentionally or through wear and tear—is significant in dictating how liquids behave on it.
There are specific models that help explain these interactions, notably the Wenzel and Cassie-Baxter models. By delving into these models, one can gauge how surface texture and chemistry intertwine to influence wettability.
Wenzel and Cassie-Baxter Models
The Wenzel model posits that when a droplet rests on a rough surface, it penetrates into the valleys of the texture, resulting in an increased apparent contact area between the droplet and the solid. This model suggests that surface roughness enhances the wettability. The formula for the Wenzel situation is as follows:
[ \cos\theta_W = r \cdot \cos\theta\n]
Where (\theta_W) is the apparent contact angle on a rough surface, (\theta) is the contact angle on a smooth surface, and (r) is the roughness ratio (the ratio of the actual surface area to the projected area).
Conversely, the Cassie-Baxter model deals with situations where the droplet remains suspended over the peaks of the rough surface rather than penetrating it deeply. This scenario leads to a decrease in the contact area and can result in higher water-repellent properties. The equation for the Cassie-Baxter model is:
[ \cos\theta_C = f_1 \cdot \cos\theta_1 + f_2 \cdot \cos\theta_2\n]
Here, (\theta_C) refers to the contact angle in the Cassie state, while (f_1) and (f_2) are the fractions of the surface covered by each phase. Both models provide insight into how the micro- and nano-structured surfaces can be engineered to achieve desired wettability effects.
Real-World Examples
The ramifications of surface roughness and its impact on contact angles play out in many practical applications. Here are a few notable examples:
- Lotus Effect: The remarkable self-cleaning properties of lotus leaves arise from their microscopic texture, which promotes a high contact angle and, therefore, minimizes the adhesion of dirt and water. This effect is a prime example of the Cassie-Baxter model in action, as droplets bead up and roll off, taking dirt along with them.
- Textiles: In the fashion industry, surface treatments on fabrics can lead to water-resistant clothing. By manipulating the surface structure of the textiles, manufacturers can achieve varying contact angles, thus determining how the fabric interacts with moisture.
- Biotechnology: In implants and medical devices, the surface roughness can influence how biological fluids interact with devices. The wettability can either promote or hinder cell adhesion, thereby affecting healing and integration into the body.
Applications of Surface Tension
Surface tension is more than just a theoretical concept; it permeates various aspects of both natural phenomena and engineered systems. Understanding surface tension has profound applications in many fields. These include formulation industries, biomedical applications, and even everyday tasks like cleaning and painting. By exploring the importance of surface tension, we can better appreciate its real-world relevance and the intricate ways it interacts with different materials and conditions.
Emulsions and Foams
Emulsions and foams are classic examples where surface tension plays a crucial role. An emulsion, such as milk, is a mixture where tiny droplets of one liquid are dispersed in another. The science behind it involves balancing surface tension forces to keep these droplets suspended. Surfactants, which reduce surface tension, allow for the stabilization of emulsions. In industries like food and cosmetics, understanding how to create stable emulsions is key to product consistency and performance.
Conversely, foams are gas bubbles trapped in a liquid. Consider whipped cream; the texture and volume are a direct result of the balance of surface tension and the types of stabilizers used.
Through control of surface tension, manufacturers can innovate products with desirable properties. Therefore, knowledge of how to manipulate this physical phenomenon is indispensable in formulating stable emulsions and foams for various applications.
Inkjet Printing Technologies
Surface tension also finds its way into modern printing technologies, particularly inkjet printing. In this context, surface tension affects how ink droplets are formed and ejected from the print head. An ink’s formulation, which includes solvents and additives, must balance viscosity and surface tension to ensure precision in printing.
If the surface tension of the ink is too high, droplets may not form correctly, leading to blotches or incomplete prints. This demonstrates the importance of carefully designing inks for optimal performance.
Moreover, advancements in material science mean innovations like drop-on-demand printing, where the precise control of droplet formation is crucial. Innovations continue to emerge, making this a fascinating area to watch.
Microfluidics Systems
Microfluidics embodies surface tension in a realm that merges science and engineering. These systems manipulate tiny volumes of fluids, with applications ranging from diagnostics to drug delivery. The behavior of fluids in microchannels is critically affected by surface tension. It can either promote flow or cause issues like channel blockage.
One might ask, how can researchers control such a minuscule scale? Here, surface tension is paramount. For instance, the design of hydrophilic and hydrophobic surfaces can influence fluid behavior dramatically. Different methods for channel fabrication and surface modification can optimize performance, making microfluidic devices function effectively.
Ultimately, understanding surface tension is not just an academic pursuit; it is foundational to developing and optimizing technologies across diverse fields. Its application continues to evolve, providing solutions that ripple across industries.
Contact Angle and Material Science
Understanding the intricacies of contact angle is essential in the field of material science. This relationship is not merely academic; it underpins numerous practical applications spanning various industries. By exploring contact angle behavior, scientists and engineers can gain insights into how materials interact with liquids, thereby influencing product design, performance, and functionality.
Wettability and Surface Modification
Wettability, or the ability of a liquid to maintain contact with a solid surface, is largely characterized by the contact angle formed at the interface. When a drop of water is placed on a surface, the contact angle can tell a story about the surface's characteristics.
- High Contact Angle: A larger angle typically suggests poor wettability, indicating that the surface may be hydrophobic. Materials like Teflon display this behavior, repelling water efficiently.
- Low Contact Angle: Conversely, a small angle indicates hydrophilicity, meaning the material can readily interact with water. This property is advantageous in coatings that are designed to enhance wettability for applications such as paints and adhesives.
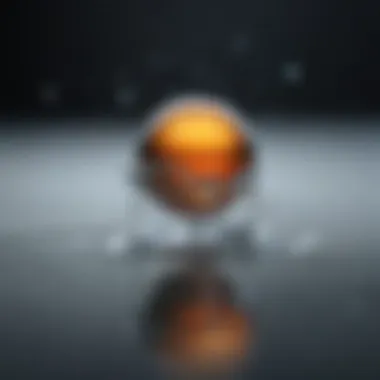
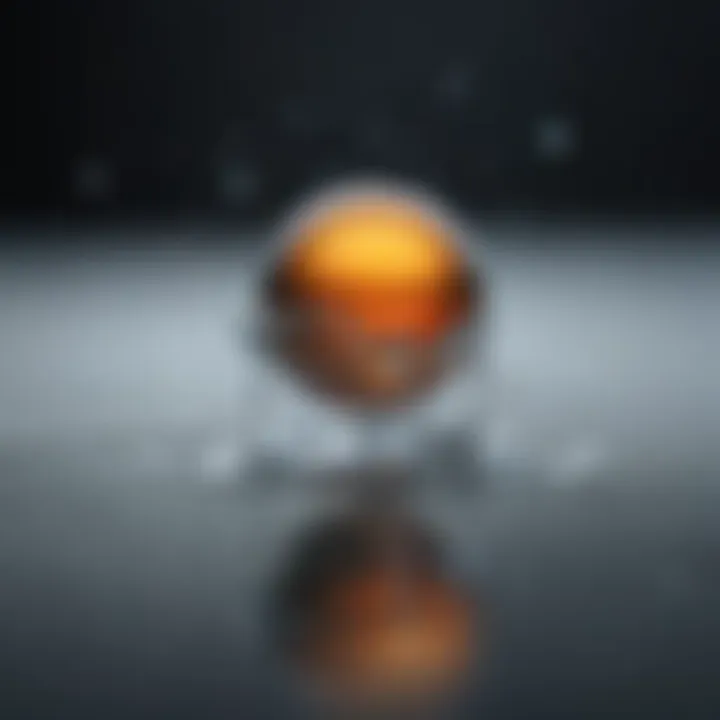
Surface modification techniques play a pivotal role in controlling wettability. By altering a surface's chemistry or texture, material scientists can tune the contact angle to achieve desired characteristics. Common methods include:
- Chemical Treatments: Applying silanes or polymers can alter chemical properties and affect contact angle.
- Plasma Treatments: These processes modify surface energy, leading to significant changes in wettability.
- Texturing: Techniques like laser etching create microstructures on a surface, which can enhance hydrophobicity or hydrophilicity.
The ability to manipulate these properties allows for innovative applications in fields ranging from biomedical devices to electronics, where controlling moisture management is crucial.
Self-Cleaning Surfaces
One fascinating application of understanding contact angle and wettability is the development of self-cleaning surfaces. These surfaces leverage nanoscale textures and specific chemical properties to ensure that dirt and debris easily wash away when exposed to moisture.
The principle behind self-cleaning surfaces often relates to the lotus effect, named after the lotus flower, which remains clean despite being in muddy water. The following are key elements contributing to the functionality of self-cleaning surfaces:
- High Contact Angle: By achieving a high contact angle, water beads up rather than spreading out. This beading action allows dirt particles to be lifted off the surface.
- Surface Texture: A rough surface increases the effectiveness of the water droplet in picking up dirt and contaminants.
- Hydrophobic Coatings: Specialized coatings can be applied that enhance the self-cleaning property further, often incorporating nanomaterials to achieve the desired roughness and hydrophobicity.
The ability to create surfaces that refuse to be stained or dirty holds significant potential in various industries, from construction to automotive – these innovations not only improve aesthetics but also minimize maintenance.
In summary, the connection between contact angle and material science is deep and multifaceted. By mastering wettability and exploring the means to engineer surfaces, scientists lay the groundwork for advances that can have a profound impact on technology, sustainability, and everyday life.
Environmental Significance of Surface Tension
Surface tension plays a crucial role in the environment, influencing both physical processes and biological activities. It acts as a barrier at the liquid-gas interface, governing how liquids behave in natural systems. Understanding the environmental significance of surface tension helps highlight its pivotal roles—from aiding in water retention in soils to promoting life forms at micro and macro levels.
Role in Biological Systems
Surface tension is essential in various biological processes. One notable example lies in the structure and function of cells. The cell membrane, primarily composed of a lipid bilayer, has interface properties that illustrate surface tension dynamics. This tension is crucial for maintaining cell shape and facilitating the movement of substances in and out.
In the respiratory system, a protein called surfactant reduces surface tension in the alveoli, aiding in efficient gas exchange. Without sufficient surfactant, alveoli could collapse, significantly impairing respiratory function. Moreover, aquatic organisms like water striders exploit surface tension to move gracefully across water surfaces, showcasing how this phenomenon supports life.
Impact on Ecosystem Dynamics
Surface tension isn’t just a standalone concept; it influences broader ecosystem dynamics as well. For instance, in wetlands, the interactions between surface tension and plant structures work together to support various habitats. The ability of water to form droplets means it can transport essential nutrients and pollutants, affecting everything from soil health to aquatic ecosystems.
- Infiltration and Erosion Control: Surface tension affects how water infiltrates the soil, influencing erosion rates. Waters with low surface tension may lead to faster infiltration, which impacts water retention and movement in the soil profile.
- Pollution Mitigation: Oils and other contaminants often disrupt surface tension. Understanding how surface tension influences pollutant behavior can provide insights into remediation strategies for contaminated water bodies.
- Influence on Climate: The dynamics of surface tension can also affect cloud formation and precipitation patterns, ultimately playing a role in climate regulation.
"Surface tension serves as a significant force in various biological and ecological processes that sustain life on Earth."
Interdisciplinary Connections
Understanding surface tension and contact angle goes beyond the confines of chemistry and physics; it threads its way through an array of disciplines, forging valuable connections that enhance both theoretical knowledge and practical applications. In this section, we’ll delve into the implications of these phenomena within various fields, highlighting how they pivotally influence advancements across several scientific arenas.
Physics and Chemistry Interplay
The relationship between physics and chemistry is often described as a dance, where each step must be synchronized to achieve harmony. Surface tension, rooted in physical principles, reflects molecular interactions and forces. It reveals how particles in a fluid behave under varying conditions. For instance, consider the behavior of water droplets on a leaf; physics explains how the cohesive forces among water molecules create a shape, while chemistry brings in the understanding of the molecular structure of water itself.
Moreover, the concept of contact angle is vital in this interplay. A smaller contact angle suggests better wettability, influenced by both the physical surface properties and the chemical composition. Understanding this duality is crucial, especially in the development of solutions for fluid dynamics issues in scenarios such as microfluidics or biomedical applications. In laboratories, one can observe how modifying surface chemistry—perhaps by using a surfactant—affects wetting properties, merging physics with chemistry seamlessly.
Relevance to Engineering Fields
In the realm of engineering, the significance of surface tension and contact angle cannot be overstated. They play critical roles in material science, manufacturing processes, and environmental engineering. Engineers constantly grapple with issues that arise from the interactions between fluids and solid surfaces. Here, the principles behind surface tension inform the design of efficient systems and the selection of materials.
- Coatings: In the development of coatings, understanding the contact angle helps predict how well a substance will adhere to a surface. For example, when creating paints or sealants, where desired properties like durability and water-resistance are paramount, careful consideration of surface tension can lead to superior products.
- Biomedical Engineering: In designing medical devices, knowledge of how fluids behave on different materials can guide the choice of biomaterials that enhance compatibility. A classic instance is in the design of stents, where the ability of blood to flow around the device hinges on the interplay of surface properties.
- Environmental Applications: Surface active agents play important roles in pollution control. When designing treatments for oil spills, for instance, incorporating understanding of surface tension helps engineers select the right dispersants to optimize clean-up efforts.
Given these myriad connections to fields beyond pure science, it’s clear that exploring surface tension and contact angle equips professionals with insights that can revolutionize their respective fields. By fostering a multidisciplinary perspective, we open doors to innovative solutions and a deeper understanding of the intricate dynamics at play in our world.
Future Directions in Surface Tension Research
The domain of surface tension research is not a static field; it is ripe for innovation, exploration, and new methodologies. As the understanding of surface tension and contact angle continues to evolve, integrating advanced techniques and applications becomes imperative. This final section delves into the future directions that promise to enhance our comprehension and application of these pivotal phenomena in various scientific and engineering disciplines.
Innovative Measurement Techniques
In the pursuit of accurately measuring surface tension and contact angle, researchers are moving towards novel methodologies that offer greater precision and adaptability. Existing techniques, while reliable, often have limitations that newer methods can address. Innovations like the microfluidic-based measurements allow for the manipulation of fluids on a microscopic scale, providing insights that were previously unattainable.
Among these advanced techniques, optical methods are gaining traction. Techniques such as high-speed imaging and interferometry provide real-time data about droplet behavior, enabling scientists to observe dynamics unobserved by older methods. For instance, laser-induced fluorescence has been employed to visualize surfactant concentrations, shedding light on how additives influence surface behavior at minuscule scales.
Considerations for adopting these innovative techniques include:
- Cost: While more accurate, some new methods can be cost-prohibitive for smaller labs.
- Compatibility: Ensuring that new techniques work well with existing materials can pose a challenge.
- Training needs: Implementing advanced measurement techniques may require specialized training for researchers.
Emerging Applications in Nanotechnology
Nanotechnology stands as a frontier of modern engineering, and the role of surface tension within this field is both significant and multifaceted. As materials shrink to the nanoscale, the surface-to-volume ratio dramatically increases, thus amplifying the effects of surface tension. Understanding this phenomenon becomes crucial when fabricating nanomaterials that rely on precise control over their properties.
One promising application is in the development of smart coatings that employ surface tension dynamics for self-cleaning or anti-fogging properties. By engineering materials at the nanoscale, scientists can create surfaces where water droplets easily slide off, carrying dirt and contaminants with them. This is particularly useful in fields ranging from aerospace to medical devices, where cleanliness and efficiency matter.
Another field seeing the benefits of surface tension research is drug delivery systems. Here, nanoparticles can be designed to optimize their surface characteristics, thus improving how they interact with biological systems. This can lead to enhanced targeted therapies where medications are precisely delivered to diseased cells while minimizing side effects.
"The understanding of how surface tension behaves at the nanoscale is reshaping how we approach material design and biological applications."
As research continues to expand into these areas, the potential for breakthroughs that align with environmental sustainability and efficiency in diverse industries appears increasingly promising.
By focusing on innovative measurement techniques and cutting-edge applications in nanotechnology, future research in surface tension and contact angle holds the promise of profound impacts across multiple fields, inviting researchers and industry professionals alike to forge new paths in their endeavors.