Exploring Wireless Strain Gauges: Principles and Innovations
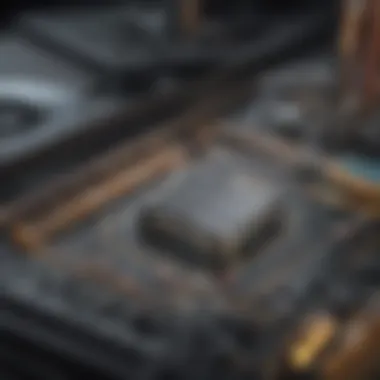
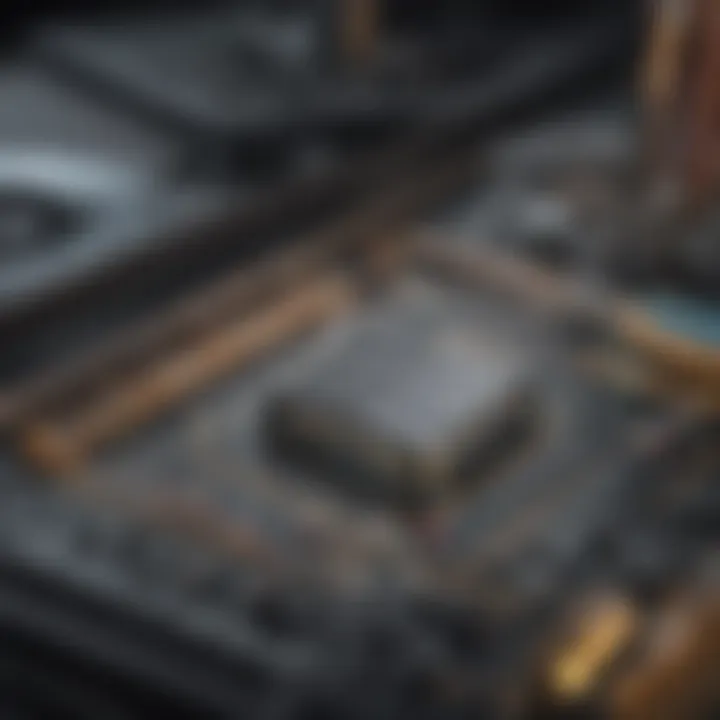
Intro
Wireless strain gauges represent a significant evolution in the field of measurement technology. These devices are crucial for monitoring stress and strain in various materials and structures. With advancements in wireless technology, the potential applications of strain gauges have expanded remarkably. Industries now leverage wireless strain gauges for real-time data collection, reducing the need for cumbersome wiring. This shift not only simplifies installations but also enhances the accuracy of measurements.
In this article, we will explore a range of topics regarding wireless strain gauges. We will examine the fundamental principles behind their operation, outline their various applications, and look at the innovations shaping their future. Moreover, we will analyze the advantages they hold over traditional wired solutions and outline the challenges that remain in their development.
The aim is to provide a comprehensive and insightful resource for students, researchers, educators, and professionals interested in understanding the impact of wireless strain gauges in modern engineering and beyond.
Research Highlights
Key Findings
Wireless strain gauges have been found to offer significant advantages over traditional wired gauges. The reduction in installation complexity and the improvement in measurement accuracy are particularly noteworthy. Studies show that wireless systems minimize the risk of interference due to external electrical signals, thereby providing clearer data.
Moreover, they facilitate long-distance monitoring applications in civil engineering, aerospace, and industrial settings. Their ability to operate on low power consumption makes them ideal for remote or inaccessible locations.
- Enhanced data precision
- Decreased installation time
- capabilities for remote monitoring
"The adoption of wireless strain gauges significantly reduces maintenance costs while improving data integrity across various applications."
Implications and Applications
Several sectors utilize wireless strain gauges for diverse applications. In civil engineering, they monitor the structural health of bridges and buildings. In aerospace, they measure stress on aircraft components during flight tests. Industrial applications include monitoring machinery and equipment for detrimental wear.
The implications of these advancements extend to research and development as well. By integrating wireless strain gauges into experimental setups, researchers gain unprecedented accuracy and efficiency in collecting data and monitoring critical parameters during tests.
Methodology Overview
Research Design
This assessment of wireless strain gauges involves both qualitative and quantitative research methods. Experimental procedures focus on comparing wireless options with traditional wired counterparts. Gathering results from different environments will provide insight into performance capabilities.
Experimental Procedures
In an experimental setup, various models of wireless strain gauges will be deployed in several locations. Data will be collected over a specified period to analyze performance and reliability. The metrics of interest include battery life, accuracy, and signal strength. Adopting statistical analysis will facilitate a thorough comparison of these devices against traditional designs.
Foreword to Wireless Strain Gauges
Wireless strain gauges represent a remarkable evolution in the field of measurement technology. They are pivotal in capturing data on material deformation without traditional wiring constraints. This innovation allows for flexibility in various applications across industries. The significance of wireless strain gauges lies in their ability to monitor structural integrity and mechanical stress in real-time, enhancing safety and performance.
Definition and Overview
A wireless strain gauge is a device that measures the amount of deformation (strain) experienced by an object when subjected to force. Unlike their wired counterparts, these gauges operate without physical connections, transmitting data wirelessly. This advancement not only simplifies setup but also reduces the potential for cable-related damage or interference.
In essence, a strain gauge usually consists of a sensor that deforms in response to stress. This deformation alters an electrical signal, which is then transmitted wirelessly to a monitoring system for analysis. There are various types, including resistive strain gauges and optical fiber strain gauges, each offering distinct advantages depending on specific applications.
Historical Context
The concept of strain measurement dates back to the early 20th century. Initially, wired strain gauges were developed, relying on electrical resistance changes. Over decades, as technology advanced, the need for greater flexibility and efficiency became evident. Thus, the shift towards wireless systems began.
In the early 21st century, developments in wireless communication and miniaturization of electronics paved the way for the emergence of wireless strain gauges. These innovations have expanded the application scope, making them integral in fields such as aerospace, civil engineering, and biomedical innovations.
Today, the continuous evolution in sensor technology and battery life significantly enhances the relevance of wireless strain gauges in modern engineering solutions. Leveraging these devices results in improved data acquisition processes, thus contributing positively to safety protocols and engineering advancements.
Fundamental Principles of Strain Measurement
Understanding the fundamental principles of strain measurement is essential for grasping the operation of wireless strain gauges. This section explores the underlying concepts and mechanisms that define how strain is detected, interpreted, and utilized across various applications. A solid grasp of these principles allows for effective implementation and enhances the overall reliability of measurements.
The Concept of Strain
Strain refers to the deformation of a material when subjected to an external force. It is a critical parameter that engineers and scientists measure to assess the structural integrity and performance of materials. Strain can be classified into various types, including tensile, compressive, and shear strain, depending on the nature of the applied force.
Mathematically, strain is defined as the ratio of the change in length to the original length of the material. It is expressed as:
[ \textStrain (\epsilon) = \frac\Delta LL_0 ]\
Where:
- (\Delta L) = Change in length
- (L_0) = Original length
Strain can be measured in a variety of conditions. Understanding the relationship between strain and stress in materials involves grasping concepts such as elasticity and plasticity. The elasticity of a material determines how well it returns to its original shape after the removal of stress. This is relevant in applications ranging from construction to aerospace engineering, where materials must withstand substantial forces without failing.
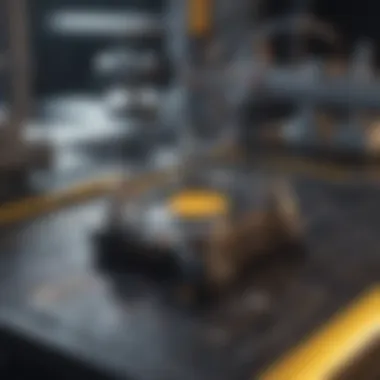
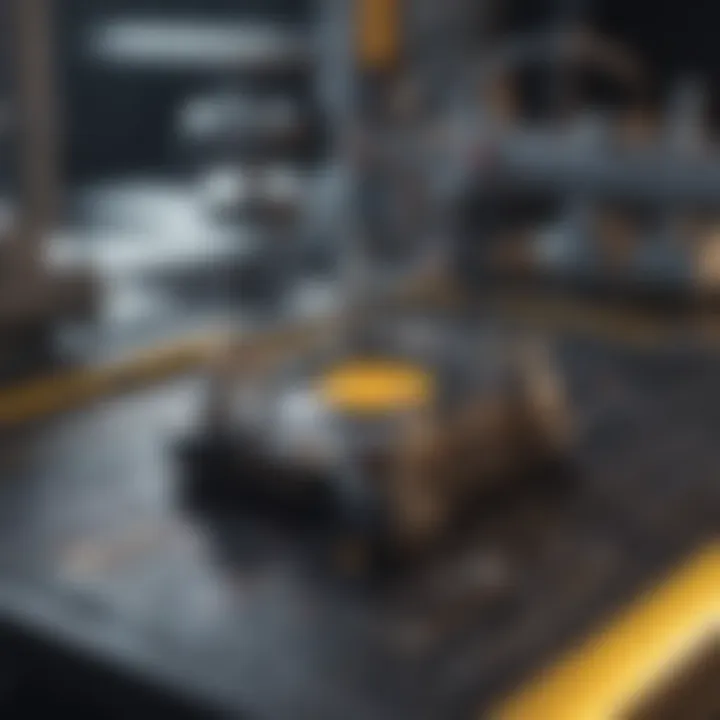
Mechanisms of Strain Gauging
Strain gauging is a technique used to measure the amount of deformation a material undergoes when subjected to stress. Strain gauges work on the principle that the resistance of a conductor changes with deformation. When a conductor is stretched or compressed, its length and cross-sectional area change, resulting in a change in electrical resistance.
The two main types of strain gauges are:
- Metallic Wire Gauges: These consist of fine wires crafted into a grid pattern. As the material deforms, the metal experiences stress, altering its resistance.
- Semiconductor Strain Gauges: Using silicon as the sensing element, these offer higher sensitivity than metallic gauges. They exploit the fact that the resistive changes in semiconductors are more pronounced with strain variations.
In practical applications, a strain gauge is affixed to the surface of the material being tested. The gauge then converts the mechanical deformation into an electrical signal, which can be measured and interpreted. A key advantage of wireless strain gauges is their ability to transmit data without physical connections, enhancing installation flexibility and reducing environmental vulnerability.
"The integration of modern technology in measuring strain offers improvements in accuracy, reliability, and mobility in various industries."
Understanding these fundamental principles not only aids in selecting the appropriate strain gauge for specific applications but also in developing innovative solutions tailored to industry needs. This knowledge is invaluable for both researchers and practitioners alike, ensuring effective monitoring of material performance and integrity.
Design and Components of Wireless Strain Gauges
The design and components of wireless strain gauges are critical to understanding their functionality and effectiveness. A well-designed strain gauge can provide accurate measurements, transmit data wirelessly, and withstand challenging environments. This section will explore various aspects of these components, shedding light on how they come together to create efficient and reliable measurement systems that cater to diverse applications.
Materials Used in Fabrication
The selection of materials in the fabrication of wireless strain gauges significantly influences their performance. Typically, strain gauges are made from materials that exhibit high sensitivity to deformation. Common materials include metallic foils, polyimides, and silicon-based sensors.
- Metallic Foils: These are the most widely used materials. Aluminum and constantan (a nickel-copper alloy) are prevalent due to their stable electrical properties and good gauge factor.
- Polyimides: They are flexible and can conform to various surfaces, making them ideal for applications requiring adaptability.
- Silicon-Based Sensors: Silicon sensors utilize semiconductor properties and provide high precision. They often operate under different conditions, offering robust solutions for extensive applications.
The choice of material not only affects the performance characteristics of the gauge but also its durability. For example, environmental resistance is crucial in applications subjected to harsh conditions. Indeed, the material must be capable of ensuring longevity without compromising the sensitivity or accuracy of the readings.
Sensor Architecture
The architecture of wireless strain gauges encompasses both the design of the sensor itself and the integration of wireless communication technology. Generally, a typical wireless strain gauge consists of three main components: the sensing element, the signal conditioning circuitry, and the transmitter.
- Sensing Element: This part directly detects strain. It is usually encapsulated in a protective layer to enhance durability and to ensure reliability under challenging conditions.
- Signal Conditioning Circuits: These circuits serve to amplify and filter the signals from the sensing element, preparing them for transmission. They are essential for accurately interpreting strain data and contribute to data integrity.
- Transmitter: The transmitter wirelessly sends the processed signals to a central data collection system, such as a computer or a cloud-based platform.
The layout and design of these components significantly influence the overall performance, including factors such as measurement range, sensitivity, and battery life. Optimization of sensor architecture requires a balance between size, complexity, and functionality to maximize efficiency without unnecessary constraints.
Power Supply and Communication Technology
Wireless strain gauges must use an efficient power supply system to maintain performance without the encumbrance of wired connections. Battery technology plays a significant role in this aspect. Many wireless strain gauges incorporate lithium-ion or lithium-polymer batteries, known for their high energy density and long life.
In addition, innovative energy harvesting techniques, such as solar cells or vibrational energy converters, are being explored to extend operational lifetimes without frequent battery replacements.
On the communication side, various protocols are used to transmit data wirelessly. Common options include:
- Bluetooth Low Energy (BLE): This is popular for its energy efficiency and relatively high data rates, making it suitable for many applications.
- Zigbee: Favored for its mesh networking capability, facilitating communication over larger distances while maintaining low power consumption.
- LoRa (Long Range): Known for its long-range capabilities, LoRa works well in remote monitoring applications, allowing data transfer over vast expanses.
The choice of communication technology must align with the application’s requirements regarding range, data latency, and durability. Each option has its strengths and potential limitations, which must be factored into the design process.
"The design and components of wireless strain gauges determine not only their performance but also their applicability across various industries. Choosing the right combination of materials, architecture, and technology can enhance efficiency in capturing and translating strain data."
In summary, the design and components of wireless strain gauges are integral to their functionality and efficiency. Understanding their materials, architecture, and technology enables industries to select suitable configurations suitable for specific applications, paving the way for enhanced performance in strain measurement.
Advantages of Wireless Strain Gauges
Wireless strain gauges present a significant breakthrough in measurement technology, offering numerous benefits over their wired counterparts. These advantages not only enhance the efficacy of strain gauging but also contribute to broader applications across various fields. In this section, we will discuss several key benefits, namely the elimination of wires, enhanced flexibility and mobility, and lower maintenance requirements. Each of these elements plays a crucial role in the overall functionality and appeal of wireless strain gauges.
Elimination of Wires
One of the most prominent advantages of wireless strain gauges is the elimination of physical wiring. Traditional strain gauges rely on a complex network of wires to transmit data, creating potential points of failure and complications during installation. With wireless designs, the need for extensive cabling is reduced or even removed entirely. This innovation simplifies the installation process, allowing for easier placement in hard-to-reach areas.
Additionally, removing wires minimizes safety hazards associated with electrical connections, especially in environments where moisture or extreme temperatures may affect cable performance. Less wiring also reduces the overall weight of measurement systems, making them easier to manage. In many cases, eliminating wires leads to improved aesthetic outcomes, providing a cleaner and more professional look in engineering applications.
Enhanced Flexibility and Mobility
Wireless strain gauges enhance flexibility and mobility, advantages that are particularly valuable in dynamic environments such as construction sites or industrial facilities. Their lack of wires allows for easier repositioning of sensors as project requirements evolve. This level of adaptability is crucial in an era where projects often shift focus and requirements.
Moreover, the use of wireless technology permits remote monitoring and data collection. Engineers and researchers can gather real-time data without being physically present at the installation site, which enhances efficiency and allows for timely decision-making. This capability is especially essential in research settings or expansive monitoring applications, such as structural health assessments of bridges or large machinery.
Lower Maintenance Requirements
Another significant advantage of wireless strain gauges is their lower maintenance requirements. In traditional wired systems, maintaining the integrity of connections is critical. Regular inspections are needed to ensure that cables function correctly and are free from wear and tear. This upkeep can be labor-intensive and costly over time.
On the contrary, wireless systems have fewer mechanical components subject to wear. Without wires connecting the sensors, there is a reduced chance of connection failure. The overall durability of wireless strain gauges is often greater, leading to longer operational life and reduced need for replacement or repair. Consequently, these devices become more cost-effective when considering the total expenditure over time.
"The elimination of wires in strain gauges marks a significant shift in measurement technology, contributing to efficiency across various industries."
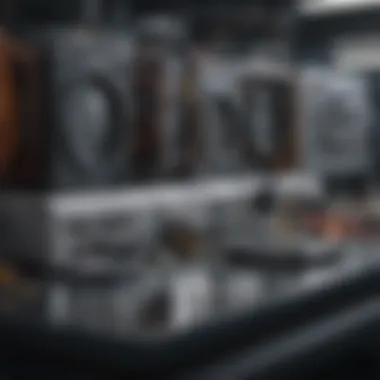
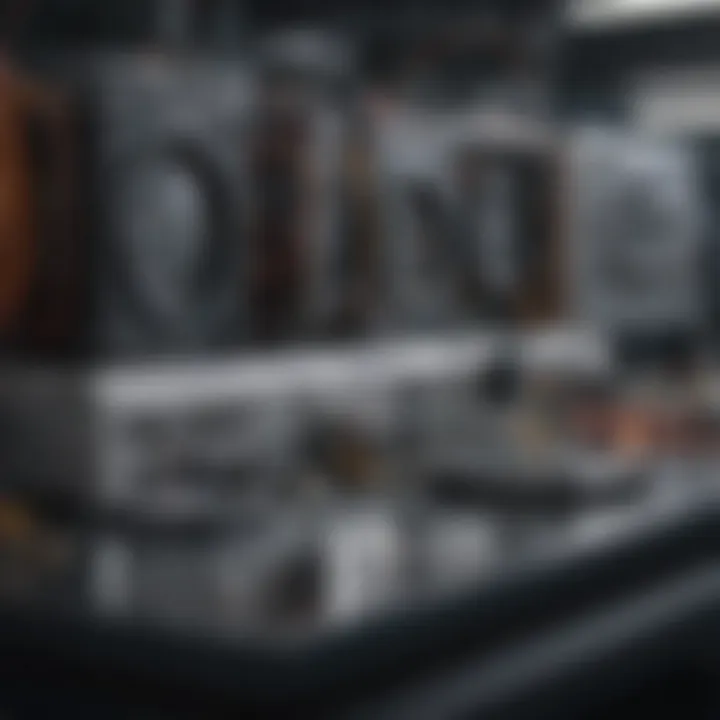
In summary, the advantages of wireless strain gauges, encompassing the elimination of wires, enhanced flexibility and mobility, and lower maintenance needs, position them as advanced tools for modern applications. Their design optimizations cater to dynamic industrial demands, allowing for effective monitoring and analysis across diverse sectors.
Applications Across Industries
The application of wireless strain gauges spans a variety of industries and plays a critical role in monitoring and improving systems. Their innovative features enable real-time data collection, which helps in making informed decisions. As technology advances, the importance of these gauges grows, enhancing functionality while reducing costs and complexity. In many cases, they provide insights that were previously unattainable with traditional wired devices.
Structural Health Monitoring
Wireless strain gauges are essential in the field of structural health monitoring. They enable engineers to understand the degeneration of infrastructure over time. Accurate strain measurement helps identify weaknesses in bridges, buildings, and other structures.
- The advantages include:
- Real-time data transmission: Allows for immediate analysis, which is vital for safety.
- Reduced installation complexity: Without wires, sensors can be placed in hard-to-reach areas.
Potential risks are mitigated through constant monitoring. Early detection of flaws prevents costly repairs or catastrophic failures.
Aerospace Applications
In aerospace, weight is critical. Wireless strain gauges contribute to reducing the weight of sensor systems, which benefits aircraft performance. They provide important data on the load and stress on various parts of an aircraft during flight.
- Key points include:
- Efficient data collection: Wireless gauges enhance data collection during real-time tests.
- Improved safety: Provides insights that ensure flight safety by monitoring structural integrity.
Their application supports the rigorous standards of the aerospace industry, where precision and reliability are paramount.
Biomedical Engineering
In biomedical engineering, wireless strain gauges can be utilized in various applications, such as prosthetics and biomechanical assessments. These sensors help in understanding how different forces act on the body.
- Notable benefits:
- Enhanced patient experience: Reduces wires, improving comfort during assessments.
- Fine-tuned data: Collects detailed data necessary for optimizing designs and treatments.
By examining strain in action, healthcare professionals can better analyze patients’ conditions and improve medical devices.
Automotive Industry Innovations
The automotive industry also sees substantial advancements from wireless strain gauges. They are integral to testing the performance of components under different strains and loads during vehicle operation.
- Important aspects:
- Streamlined testing: Allows for flexible placement on vehicles for optimal data.
- Data-driven insights: Facilitates enhancements in design and safety features for vehicles.
Wireless strain gauges thus enable more innovative designs in automotive engineering, where safety and efficiency play critical roles.
Wireless Strain Gauges in Research Settings
The utilization of wireless strain gauges in research settings marks a significant advancement in measurement technology. These tools allow researchers to collect precise data without the complications of direct wiring. The ease of installation and the ability to place sensors in difficult-to-reach locations enable more extensive and varied data collection. Additionally, data can be transmitted in real-time, which provides immediate insight and facilitates more dynamic experimentation.
Focus on Academic Studies
In academic research, wireless strain gauges play a crucial role in various studies. They are used in mechanical engineering, civil engineering, and materials science. For example, when studying the structural integrity of materials, the placement of these gauges can yield more accurate results compared to traditional methods. Wireless sensors can monitor changes in strain while exposed to different temperature conditions, enabling researchers to gain insights into material behavior under stress.
Furthermore, the flexibility provided by wireless technology allows for innovative experimental setups. Researchers can design tests that were previously unfeasible. Data collection happens without the hindrance of wires, which can interfere with the integrity of the experimental conditions.
Collaborations with Industry
Collaboration between academic institutions and industry is greatly enhanced by the application of wireless strain gauges. These partnerships can lead to joint research projects that bridge the gap between theory and practical application. For instance, aerospace companies can work alongside universities to develop advanced strain measurement techniques that improve aircraft performance and safety.
Industry collaboration also equips academia with access to real-world data and application scenarios. Industries benefit from cutting-edge research while researchers gain insight into practical challenges. This synergy fosters innovation, ultimately contributing to advances in technology and engineering practices.
"The integration of wireless strain gauges in research not only enhances academic exploration but also drives real-world applications, creating a feedback loop for continuous improvement."
Challenges in Wireless Strain Gauge Technology
The deployment of wireless strain gauges technology has brought a range of advantages, but it comes with its own set of challenges. Addressing these challenges is essential for ensuring reliable performance and broadening the application possibilities of strain gauges in various industries. Understanding the limitations and obstacles of this technology helps researchers and professionals refine their approaches to utilizing these tools effectively.
Signal Interference and Data Integrity
One critical hurdle in wireless strain gauge systems is signal interference. Wireless communication can be easily disturbed by surrounding electronic devices, physical barriers, or environmental factors. If interference occurs, the data transmitted from the strain gauge to the receiver station may become corrupted or lost. This issue is particularly significant in industrial environments where many machines operate simultaneously, creating a noisy electromagnetic environment.
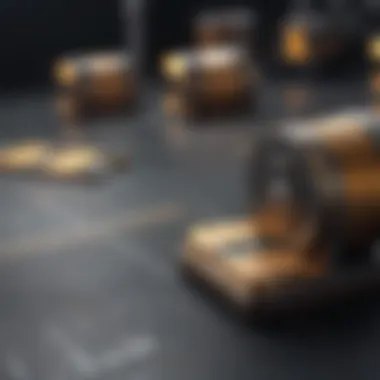
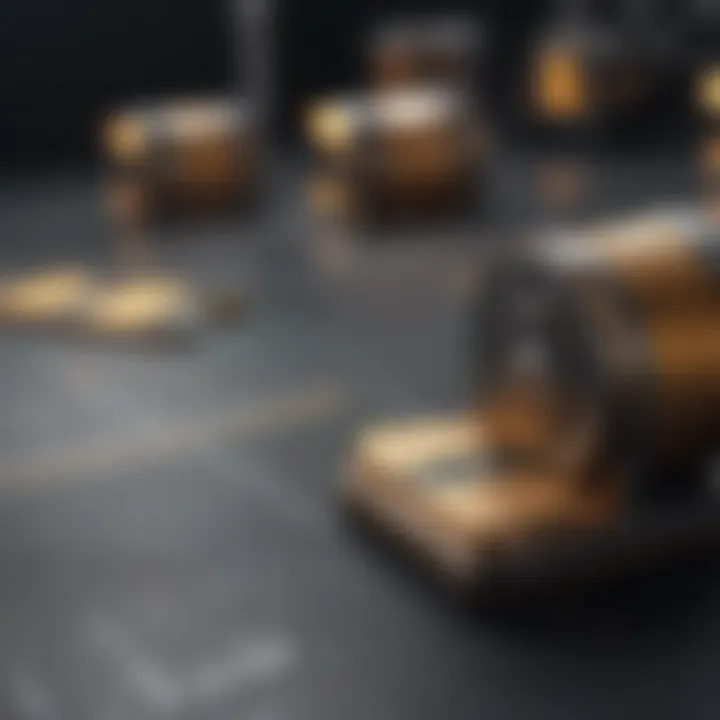
Maintaining data integrity is also paramount. The information collected needs to be accurate and reliable. Any disruption can lead to incorrect readings, which can have serious consequences, especially in safety-critical applications like structural health monitoring.
To mitigate these issues, engineers often utilize advanced encoding methods and error-correction algorithms. These techniques help ensure that the data received matches the data sent. Additionally, implementing frequency-hopping spread spectrum (FHSS) communication can help in avoiding interference by rapidly switching frequencies during transmission. This allows the system to adapt to the best available channel, reducing the likelihood of signal loss.
Battery Life Considerations
Another significant challenge encountered with wireless strain gauges is battery life. Wireless systems depend on batteries for power, and extended operation periods are crucial in many scenarios. Strain gauges that are deployed in remote or hard-to-reach areas may be difficult to access for battery replacements.
Power consumption is a critical factor for wireless sensor networks. Continuous operation of communication modules can drain batteries quickly. Therefore, it is essential for developers to strike a balance between functionality and power usage. Here are some strategies to consider:
- Low Power Modes: Implementing sleep modes can reduce consumption during periods of inactivity.
- Energy Harvesting: Utilizing energy from the environment, such as solar power, can help prolong battery life.
- Optimized Data Transmission: Reducing the frequency of data transmission or sending only essential data can conserve energy.
By addressing battery life and signal interference, the reliability and usability of wireless strain gauge technologies can be significantly enhanced.
Recent Innovations in Wireless Strain Gauge Technology
The field of wireless strain gauge technology is undergoing significant transformations as research and development push the boundaries of what is possible. These innovations are essential, as they not only improve the performance of existing devices but also enable new applications across a variety of sectors. In this section, we will discuss two primary areas of innovation: advancements in materials and emerging communication protocols. These developments have the potential to reshape the landscape of strain measurement and enhance the overall effectiveness of wireless strain gauges.
Advancements in Materials
Recent advancements in the materials used for wireless strain gauges have been a key focus for researchers. Innovative materials lead to better sensitivity, durability, and adaptability to various environmental conditions. For instance, researchers are experimenting with nanomaterials and composites to form strain sensors that are lightweight and highly sensitive to deformation.
- Nanotechnology: The incorporation of nanostructured materials allows for enhanced strain sensing capabilities. These materials can respond to even minimal deformations effectively.
- Flexible Substrates: Advances in flexible electronics mean that strain gauges can now be integrated onto surfaces that were previously unsuitable. This is particularly beneficial in biomedical applications where the sensor can be applied directly onto the skin.
- Smart Materials: The use of shape memory alloys and piezoelectric materials opens new avenues for wireless strain gauges. These materials not only measure strain but can also absorb energy, providing a multifunctional approach.
Such enhancements in materials lead to greater accuracy, improved reliability, and wider application potential in fields like structural health monitoring and wearable technology.
Emerging Communication Protocols
Another area where significant innovation is taking place is in communication protocols used in wireless strain gauges. The choice of protocol affects how data is transmitted from gauges to monitoring systems. Improved communication protocols can increase data integrity, reduce latency, and enhance battery life.
- Low Power Wide Area Networks (LPWAN): Technologies such as LoRa and Sigfox are becoming popular in strain gauge applications due to their long-range capabilities and low power consumption. These features make them ideal for remote monitoring in construction or infrastructure health applications.
- Bluetooth Low Energy (BLE): BLE is also seeing increased use, particularly in consumer and medical devices. Its ability to transmit small packets of data efficiently at close range can adapt well to many applications requiring constant strain monitoring.
- 5G Technology: As 5G networks expand, the potential for real-time data transmission from wireless strain gauges increases significantly. This allows for immediate feedback and monitoring in critical applications such as aerospace and automotive industries.
"Advancements in communication technology are enabling wireless strain gauges to operate more efficiently and effectively, paving the way for smarter and more connected applications."
In summary, recent innovations in materials and communication protocols in wireless strain gauge technology present numerous benefits. They lead to enhanced performance, broaden application areas, and ultimately contribute to more efficient strain measurement solutions. This ongoing evolution underscores the importance of continuous research and development in maintaining the relevance and efficacy of these critical measurement tools.
Future Directions in Wireless Strain Gauge Research
As wireless strain gauge technology matures, its future directions demand immediate attention. With the pace of advancements in related fields, this area garners significant interest. Understanding the potential of future developments can inform both ongoing research and practical applications. This discussion emphasizes the critical elements of innovation in wireless strain gauges, including the integration of sophisticated technologies and the pursuit of more efficient materials.
Integrating AI and Machine Learning
The incorporation of artificial intelligence (AI) and machine learning into wireless strain gauge technology presents an exciting horizon. Machine learning algorithms can process extensive datasets generated from strain gauges, allowing for enhanced predictive analytics and real-time monitoring. This capability enables anomaly detection, leading to faster maintenance and improved safety in various applications.
- Data Interpretation: AI algorithms can derive meaningful insights from complex strain data, supporting engineers in decision-making processes.
- Adaptive Systems: Machine learning can enhance system capabilities by adapting to observed behaviors, improving efficiency in strain monitoring.
Implementing these technologies will also involve considerations related to data security and privacy. Protecting sensitive information will be essential as more devices connect within smart networks. The long-term impact of these integrations could redefine how industries approach structural health monitoring and predictive maintenance.
Miniaturization and Smart Materials
The trend towards miniaturization in electronics presents a significant opportunity in the field of wireless strain gauges. Smaller sensors can be employed in areas previously deemed inaccessible or impractical, expanding the possible applications.
- Flexible Designs: Innovations in smart materials, such as piezoelectric or shape memory alloys, pave the way for more versatile strain gauges. These materials can conform to irregular surfaces, making them ideal for various structural applications.
- Cost Efficiency: As components become smaller, production costs can decrease as well. This reduction can lead to wider adoption across different sectors.
Furthermore, the push for sustainability in design aligns with the development of smart materials. Their ability to respond to environmental changes can lead to more responsive and eco-friendly systems. Research should explore the boundaries of material science to develop high-performance gauges that support these objectives.
"The future of wireless strain gauges will be shaped by advances in AI and the development of innovative materials. As we move forward, understanding these aspects will be crucial for maximizing their potential."
In summary, focusing on integrating advanced technologies like AI and machine learning, while pushing for miniaturization and smart materials, is vital for the future of wireless strain gauge research. The merging of these factors holds substantial promise to enhance the functionality and applicability of strain gauges, ultimately influencing various industries.
Finale
In the realm of measurement technology, wireless strain gauges represent a significant advancement over traditional methods. This article has elucidated the fundamental principles, applications, and innovations associated with these devices, enabling a better grasp of their operational dynamics and importance in various sectors.
Summary of Key Insights
Wireless strain gauges are notable for their ability to eliminate physical wiring, thereby enhancing installation flexibility and reducing maintenance burdens. The integration of advanced materials and communication protocols contributes to their efficiency and reliability. Key insights include:
- Advantages of Wireless Design: The removal of wires reduces installation complexity and risk of mechanical failure.
- Wide Range of Applications: From structural health monitoring to aerospace, the versatility of wireless strain gauges is evident across industries.
- Research Contributions: Insights from academic studies and collaborative projects highlight the expanding role of wireless strain gauges in innovative solution development.
- Future Directions: Ongoing advancements in artificial intelligence and materials science are set to further enhance the capabilities of these devices.
The Role of Wireless Strain Gauges in Modern Science and Engineering
Wireless strain gauges are not merely functional tools; they are pivotal in advancing contemporary engineering practices and scientific research. Their contribution can be summarized as follows:
- Enhanced Data Collection: These gauges enable real-time monitoring of structural integrity, facilitating timely interventions that can prevent catastrophic failures.
- Integration with Smart Technologies: The potential for integrating wireless strain gauges with IoT devices and machine learning algorithms presents a frontier for predictive analytics in engineering.
- Support for Sustainability: In sustainable engineering efforts, these gauges aid in developing environmentally friendly structures by monitoring stress and strain effectively.
"The application of wireless strain gauges can fundamentally transform monitoring strategies in engineering, allowing for more efficient and responsive systems."
In summary, wireless strain gauges are more than a technological upgrade; they embody a shift towards more adaptable, efficient, and anticipatory engineering practices that will shape the future landscape of various industries. Recognizing their significance will help foster continued innovation and research in this essential field.