Exploring Hydrogen: The Element Shaping Our Future
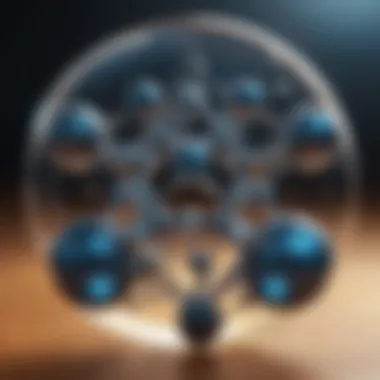
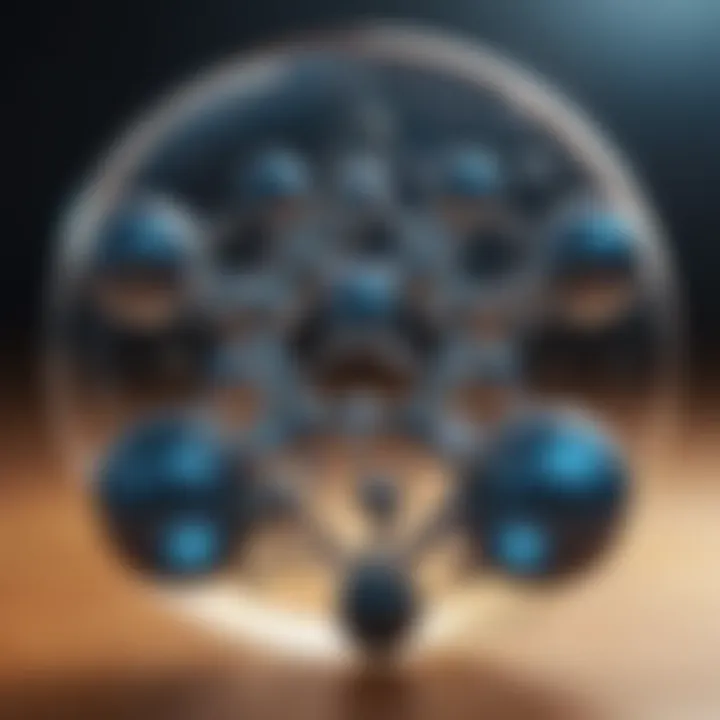
Intro
Hydrogen, the simplest and most abundant element in the universe, plays a significant role in the ongoing global shift toward sustainable energy solutions. This article explores various facets of hydrogen, encompassing its fundamental properties, methods of production, and diverse applications. It also analyzes hydrogen's potential in fostering a carbon-neutral future while outlining the complexities and challenges involved in its adoption.
In recent years, discussions surrounding hydrogen energy have intensified, as stakeholders in various sectors recognize its potential in addressing climate change and transforming energy systems. The following sections provide a detailed examination of hydrogen, shedding light on its importance in contemporary energy dialogues.
Research Highlights
Key Findings
- Elemental Properties: Hydrogen is unique due to its low atomic weight and high energy content per unit mass. It can exist in various forms, including molecular hydrogen () and ionic states.
- Production Techniques: Methods for producing hydrogen include electrolysis, steam methane reforming, and biomass gasification. Each method has distinct advantages and limitations impacting the overall sustainability of hydrogen.
- Applications in Energy Sector: Hydrogen serves as a versatile energy carrier, essential for fuel cells, industrial processes, and transportation systems. Its role can significantly reduce dependency on fossil fuels.
Implications and Applications
The implications of integrating hydrogen into the energy market are profound. Hydrogen's potential lies in its ability to act as a clean, carbon-neutral alternative. For instance:
- In transportation, shifting from gasoline to hydrogen fuel cells can reduce greenhouse gas emissions.
- In industry, hydrogen can replace carbon-intensive processes, leading to lower overall emissions.
Furthermore, scaling up hydrogen production must align with renewable energy sources to maximize environmental benefits.
Methodology Overview
Research Design
This exploration employs a multi-faceted approach. It integrates insights from scientific literature and empirical data to comprehensively evaluate hydrogen's role in energy transitions.
Experimental Procedures
- Reviewing recent studies on hydrogen production and application.
- Analyzing data from successful hydrogen projects around the globe.
- Assessing policy frameworks influencing hydrogen development in various countries.
Ultimately, understanding these methodologies offers insight into how best to harness hydrogen's attributes effectively.
As we continue, the article will delve deeper into hydrogen's properties, production methods, applications, and its broader implications for our future energy landscape.
Prelims to Hydrogen
Hydrogen is frequently recognized as a potential cornerstone in the future of energy. This section aims to articulate the significance of hydrogen within the broader context of energy transition and environmental sustainability. As the simplest and most abundant element in the universe, hydrogen presents unique advantages and challenges. Its practicality as an alternative energy source is bolstered by its versatility. Moreover, as we face mounting concerns regarding climate change and pollution, the pursuit of cleaner energy solutions becomes increasingly urgent.
Understanding hydrogen's role is essential not just for academics and industries, but also for policymakers. Its various applications span from industrial processes to storing renewable energy, making it a topic worthy of thorough exploration.
Definition and Overview
Hydrogen, represented by the symbol H, is a colorless and odorless gas at room temperature. It exists naturally as a diatomic molecule (H₂), which means two hydrogen atoms bond together. The element's atomic number is one, designating it as the foremost element in the periodic table. As a lightweight element, it possesses a low density, lending itself to numerous applications. Hydrogen is often characterized by its high energy content per mass. When burned or utilized in fuel cells, it produces water as a byproduct, making it a clean energy option.
One of hydrogen's remarkable properties is its ability to serve as a carrier of energy rather than simply being an energy source. It can be produced from various feedstocks, including fossil fuels and renewable sources, further diversifying its potential applications.
Historical Context
The narrative of hydrogen dates back to the 18th century when scientists such as Henry Cavendish first described the gas. In these early explorations, the flammability of hydrogen was noted, sparking interest in its potential applications. By the 19th century, hydrogen played a role in the development of the internal combustion engine. Over time, advancements in electrolysis brought hydrogen to the forefront as a candidate for clean energy solutions.
In the latter half of the 20th century, the energy crises heightened interest in hydrogen as an alternative fuel. Researchers began to see hydrogen's potential beyond merely being a byproduct. Today, ongoing discussions revolve around the hydrogen economy and its implications for global energy systems. The historical evolution showcases not just scientific inquiry but also the societal shift towards cleaner energy in response to environmental challenges.
Hydrogen's journey reflects humanity's quest for sustainable solutions amidst growing ecological concerns.
This section establishes a foundation for understanding hydrogen's relevance in contemporary discussions on energy. With its properties, history, and ongoing advancements, hydrogen emerges as a crucial player in the transition towards a sustainable future.
Chemical Properties of Hydrogen
The chemical properties of hydrogen are critical in understanding its behavior and applications in various fields. Hydrogen is a unique element, being the lightest and most abundant in the universe. Its position on the periodic table, as the first element, sets a foundation for a multitude of reactions and interactions with other elements. Understanding these properties is essential in harnessing hydrogen effectively for energy solutions and technological advancements.
Atomic Structure
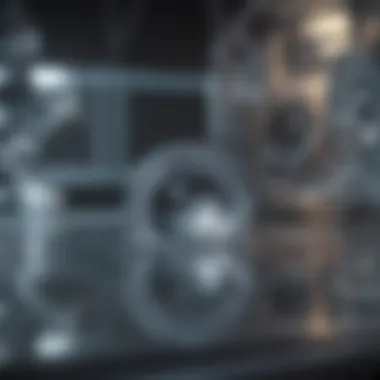
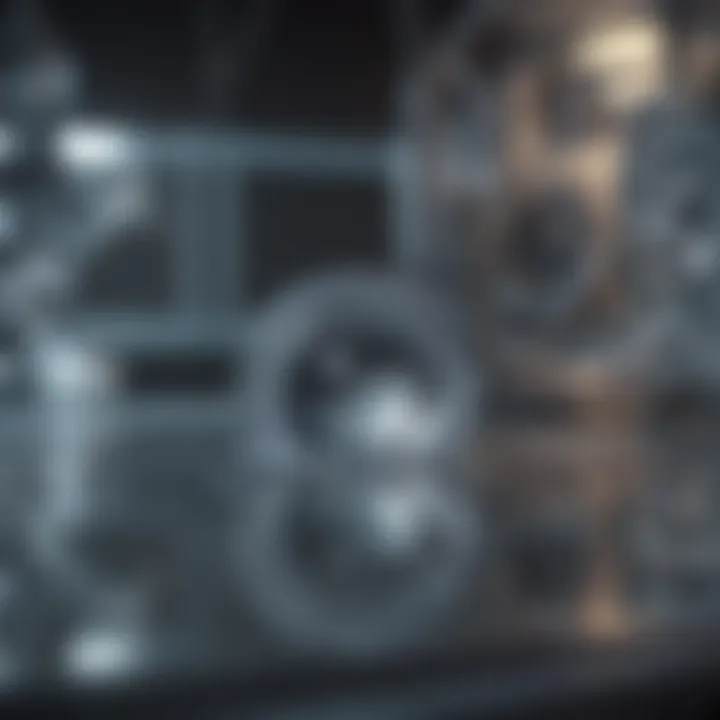
Hydrogen's atomic structure consists of a single proton in its nucleus and one electron in its outer shell. This simple arrangement gives hydrogen an atomic number of 1, making it the most fundamental element. The simplicity of this structure allows hydrogen to exist in various forms, primarily as molecular hydrogen (H₂), where two hydrogen atoms bond together. This feature makes hydrogen highly versatile in reactions, especially in combustion and synthesis processes.
In theoretical discussions, it is essential to note that the hydrogen atom can form isotopes, namely deuterium and tritium. Deuterium contains one neutron, and tritium incorporates two. These isotopes have significant implications in nuclear reactions and various scientific research fields.
Reactivity and Bonding
Hydrogen's reactivity is notable, primarily due to its electron configuration, which creates a tendency to engage in chemical bonding. It can form covalent bonds by sharing electrons and ionic bonds by transferring electrons to other elements, usually non-metals. When combined with oxygen, for instance, hydrogen forms water—a crucial compound for life.
Several factors affect hydrogen's reactivity. The conditions under which reactions occur, such as temperature and pressure, greatly influence the reaction rates. In addition, the presence of catalysts can lower activation energy, making reactions occur more readily. For example, in the process of steam methane reforming, hydrogen is produced efficiently with the help of catalysts, demonstrating hydrogen's industrial potential.
Understanding hydrogen's reactivity is also vital in fuel cell technology, where controlled reactions between hydrogen and oxygen generate electricity. This clean energy technology exemplifies the benefits of hydrogen as a fuel source, showcasing its potential in the transition towards a sustainable energy future.
Hydrogen's unique reactivity and the ability to form various compounds underline its potential in diverse applications, from energy production to industrial processes.
Overall, the chemical properties of hydrogen, rooted in its simple atomic structure yet complex behavior, highlight its significance in energy storage and future technological advancements. These characteristics not only illustrate hydrogen's role in the current energy landscape but also its potential in shaping a sustainable future.
Production Methods of Hydrogen
Understanding the production methods of hydrogen is essential for grasping its role in the energy landscape. Hydrogen is increasingly seen as a clean alternative to fossil fuels, and its production methods dictate its environmental impact, feasibility, and overall contribution to energy systems. Each method has distinct characteristics, advantages, and limitations. This section explores the three primary methods: steam methane reforming, electrolysis of water, and biological hydrogen production.
Steam Methane Reforming
Steam methane reforming (SMR) is currently the dominant method for hydrogen production. It involves reacting natural gas with steam in the presence of a catalyst. The process yields hydrogen and carbon monoxide, which can be further processed to extract more hydrogen, while carbon dioxide is released as a byproduct.
The benefits of SMR lie in its efficiency and cost-effectiveness. Natural gas, as a feedstock, is abundant and relatively inexpensive. Furthermore, the technology for SMR is well-established, making it a reliable choice for large-scale hydrogen production. However, the considerations include the carbon emissions associated with the process. Although carbon capture and storage (CCS) technology can mitigate emissions, the overall sustainability of SMR is under scrutiny as the world shifts toward lower-carbon solutions.
"Steam methane reforming is a crucial link in the hydrogen supply chain, balancing efficiency and sustainability considerations."
Electrolysis of Water
Electrolysis of water is a promising method for producing hydrogen, particularly in the context of renewable energy. This process uses electricity to split water into hydrogen and oxygen. When the electricity is sourced from renewable technologies, such as wind or solar, the hydrogen produced is often referred to as "green hydrogen" due to its minimal environmental impact.
The importance of electrolysis lies in its potential to create a sustainable hydrogen economy. Unlike SMR, electrolysis does not emit carbon dioxide when powered by renewable energy. However, the challenges are notable. The efficiency of current electrolysis methods can be improved, and the cost of electricity plays a significant role in the overall economics of hydrogen production via this method. Continuous innovations are needed to enhance efficiency and reduce costs.
Biological Hydrogen Production
Biological hydrogen production is another avenue receiving attention. This method involves using microorganisms like bacteria or algae to produce hydrogen through biological processes such as fermentation or photosynthesis.
One of the primary benefits of this method is its ability to utilize waste materials, potentially leading to a circular economy approach. Additionally, it operates at ambient temperatures, which can reduce energy consumption compared to thermal methods.
However, while biologically derived hydrogen represents a sustainable approach, the current considerations include scalability and yield. The commercial viability of this method is still in early stages. Research is ongoing to develop more efficient strains of microorganisms and optimize the production processes.
Hydrogen in Energy Storage
Energy storage is a critical aspect of modern energy systems, particularly in the context of renewable energy. Hydrogen offers unique advantages that make it an attractive option for storing energy. As society moves towards greener alternatives, understanding hydrogen's role in energy storage becomes essential.
Role in Renewable Energy Systems
Hydrogen serves as a vital bridge between renewable energy generation and consumption. When environmental conditions are favorable, excess energy from sources such as solar panels and wind turbines can be converted into hydrogen through electrolysis. This process efficiently captures energy that would otherwise be wasted. The hydrogen produced can then be stored and utilized when energy demand exceeds production.
Benefits of integrating hydrogen into renewable energy systems include:
- Scalability: Hydrogen systems can be scaled up to meet large demands, addressing extensive energy needs.
- Flexibility: It provides versatility, allowing excess renewable energy to be stored and used later.
- Sustainability: Hydrogen’s potential to be produced via electrolysis aligns with carbon-neutral goals as the energy source can be renewable.
This role not only enhances grid stability but also encourages investment in renewable technologies.
Storage Technologies
Hydrogen can be stored using various techniques, each with its own merits and drawbacks. The choice of storage technology significantly affects efficiency, safety, and cost. Some common methods include:
- Compressed Hydrogen: This method involves compressing hydrogen gas and storing it in high-pressure tanks. It is a well-established technology and is simple to implement, however, it requires significant energy to maintain high pressure.
- Liquid Hydrogen: By cooling hydrogen to extremely low temperatures, it can be stored in liquid form. This method achieves higher energy density but demands considerable energy and specialized insulation to maintain low temperatures.
- Metal Hydrides: Certain metals can absorb hydrogen gas and form metal hydrides. These storage systems are compact and efficient but may have materials challenges and recovery issues.
- Underground Storage: Large quantities of hydrogen can be stored in underground caverns. This method is cost-effective for substantial reserves but relies on geologic formations suitable for storage.
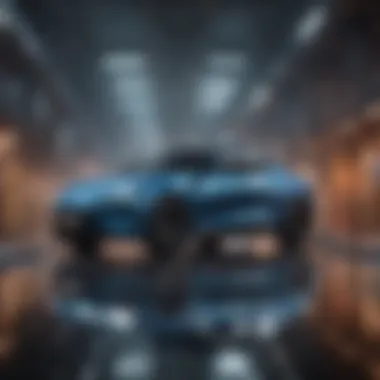
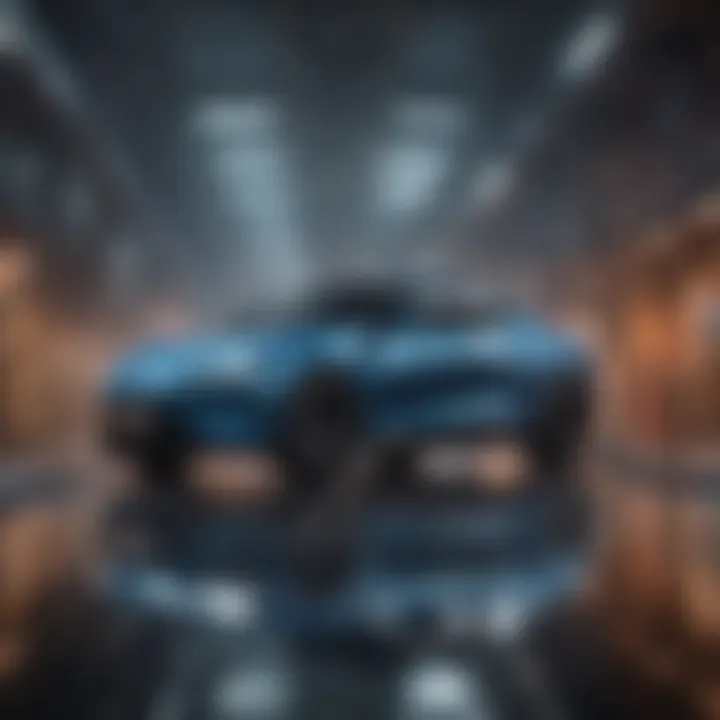
Understanding these storage technologies enables a clearer picture of how hydrogen can complement existing energy infrastructures and facilitate the transition to a more sustainable future.
"Hydrogen's ability to store renewable energy offers a promising solution to balancing supply and demand in energy networks."
Hydrogen Fuel Cells
Hydrogen fuel cells represent a pivotal technology in the pursuit of clean energy solutions. They operate by converting chemical energy from hydrogen into electricity, with water and heat as the only byproducts. This characteristic makes them especially appealing for various applications, including transportation and stationary power generation. The growing need for sustainable energy sources has placed hydrogen fuel cells in the spotlight, making their development and implementation crucial for reducing greenhouse gas emissions and achieving a carbon-neutral future.
Principles of Operation
The operation of hydrogen fuel cells is based on electrochemical processes. The fundamental component of a fuel cell consists of an anode, a cathode, and an electrolyte membrane. When hydrogen gas is supplied to the anode, it undergoes a reaction that separates it into protons and electrons. The protons pass through the electrolyte membrane to the cathode, while the electrons are diverted through an external circuit, generating electric current. At the cathode, the protons combine with oxygen from the air and the incoming electrons to produce water and heat.
This process is efficient, often exceeding 60% efficiency in converting fuel into electricity, depending on the type of fuel cell. Additionally, when using renewable hydrogen produced through electrolysis, the environmental impact is significantly reduced. This method aligns with global energy goals, supporting a shift toward greener technology.
Applications of Fuel Cells
Hydrogen fuel cells have diverse applications that highlight their versatility and potential. Some major applications include:
- Transportation: Fuel cell electric vehicles (FCEVs) are becoming increasingly popular. Companies like Toyota and Hyundai have launched models such as the Toyota Mirai and Hyundai Nexo, which operate purely on hydrogen and emit only water vapor.
- Power Generation: Stationary fuel cells can serve as back-up power sources or primary electricity generators. Industries and data centers utilize fuel cells for their reliability and low emissions, contributing to energy security.
- Portable Power: Smaller fuel cells are used in devices like laptops and smartphones, providing energy in off-grid situations or emergencies.
- Industrial Processes: Hydrogen fuel cells find use in industries that require heat and power, including chemical manufacturing and steel production. These applications can enhance efficiency while decreasing reliance on fossil fuels.
"The integration of hydrogen fuel cells in various sectors signifies a shift towards a more sustainable energy paradigm."
Environmental Implications of Hydrogen Use
The environmental implications of hydrogen use are significant in the context of the ongoing transition towards sustainable energy solutions. As energy systems evolve, hydrogen emerges as a vital component capable of addressing carbon emissions and environmental degradation. It is crucial to understand both the potential benefits and the challenges associated with hydrogen to gauge its role in future energy systems.
Carbon Footprint of Hydrogen Production
Hydrogen can be produced through various methods, each with a different environmental impact. The carbon footprint often depends on the production technique used. For example, steam methane reforming, which is the most common method today, involves extracting hydrogen from natural gas. This process can emit substantial amounts of carbon dioxide, counteracting the environmental benefits of hydrogen as a clean fuel.
In contrast, hydrogen produced from renewable resources, often termed "green hydrogen," uses electrolysis powered by renewable energy sources such as wind or solar. This method can produce hydrogen with very minimal carbon emissions. The full lifecycle analysis of hydrogen production processes is essential to understand their sustainability. Factors such as energy consumption during production and transportation emissions must be considered.
- Steam Methane Reforming: High carbon emissions due to reliance on fossil fuels.
- Electrolysis: Lower carbon emissions, dependent on the energy source used.
Potential for Green Hydrogen
Green hydrogen has gained attention as a promising solution to reduce emissions in multiple sectors. It has the potential to decarbonize industries that are difficult to electrify, such as steel and concrete production. It also serves as a clean fuel for transportation and energy storage.
Investments in green hydrogen can spearhead a transition towards more sustainable economic frameworks. Furthermore, when produced using renewable energy, it can ensure energy security while mitigating the reliance on fossil fuels.
The global push towards hydrogen adoption also underlines the importance of collaboration between governments, industries, and research institutions. This collaboration will be essential to develop infrastructure necessary for widespread green hydrogen use and to establish favorable policies promoting innovation and investment in this area.
"As the world pivots towards sustainability, green hydrogen stands out as a viable alternative to fossil fuels, supporting environmental goals and paving the way for a carbon-neutral future."
In summary, while hydrogen production and use carry environmental implications, the shift towards green hydrogen showcases a pathway towards significant reduction in carbon emissions. Navigating the complexities and challenges in hydrogen production can unlock pathways to sustainable energy systems.
Challenges Facing the Hydrogen Economy
The hydrogen economy is often hailed as a crucial component in the quest for sustainable energy solutions. Despite its potential, several challenges hinder its widespread adoption. An understanding of these challenges is essential to appreciate the complexities surrounding hydrogen as a clean energy source.
Economic Barriers
Economic barriers play a significant role in the slow progress of hydrogen technology. Currently, the costs associated with hydrogen production are high, particularly for green hydrogen generated through renewable resources. For example, electrolyzer technology needed for water electrolysis can require substantial investment. The capital setup for production facilities can be prohibitive for many companies.
Moreover, hydrogen's price competitiveness is under scrutiny. Natural gas remains cheaper in many cases, making hydrogen less appealing for energy producers and consumers. As a result, unless hydrogen becomes significantly more economical, it is likely to face continuous resistance from traditional energy industries.
Infrastructure Shortcomings
Infrastructure for hydrogen distribution and storage is another key barrier. The current pipeline and storage system is predominantly designed for natural gas and lacks the necessary adaptations for hydrogen. The physical properties of hydrogen, such as its lower density and high diffusivity, require specialized materials and technologies that are not widely available.
Many regions lack refueling stations for hydrogen vehicles. Without a robust infrastructure, consumer adoption remains limited. Investments into new infrastructure will be crucial. Governments and private investors alike must recognize the need for dedicated hydrogen facilities, including pipelines, refueling stations, and storage systems, to encourage hydrogen use.
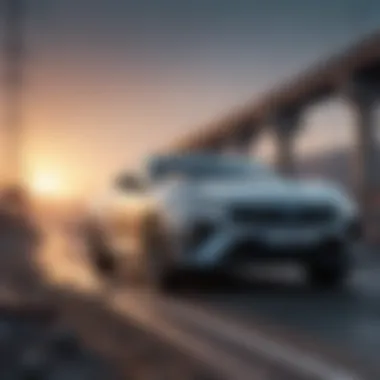
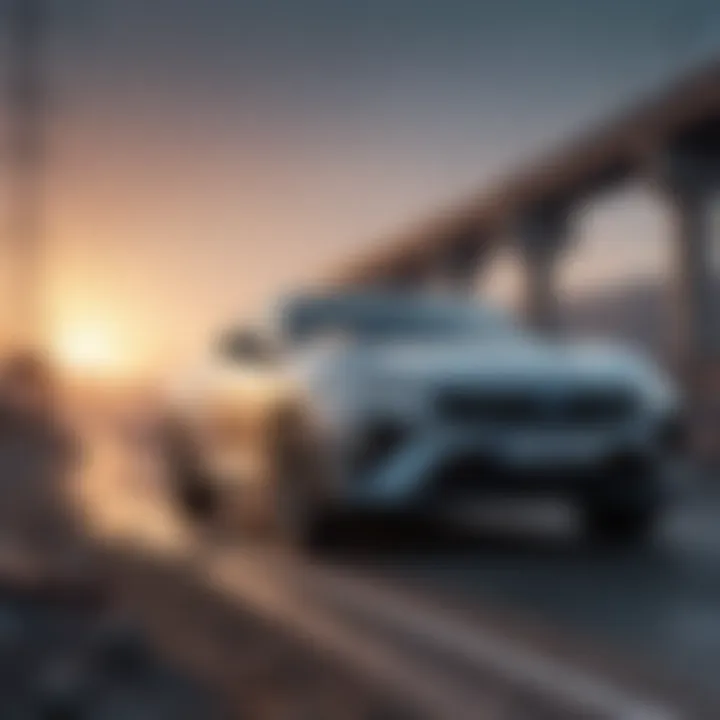
Regulatory and Policy Issues
Regulatory landscapes often pose additional challenges. The hydrogen industry requires clear and cohesive policies to guide its development and implementation. Fragmented regulations across regions can create confusion and hinder investment. Many stakeholders seeking to contribute to the hydrogen economy face uncertainty regarding safety standards, environmental regulations, and incentives for adoption.
Furthermore, policy frameworks must adapt to accommodate emerging technologies. While governments in several countries announce ambitious hydrogen strategies, these plans need to be backed by practical regulations to support the industry effectively. Ultimately, a lack of coherent regulations can stall innovation and impede progress in this pivotal sector.
"To realize the potential of hydrogen, a multifaceted approach addressing economic, infrastructural, and regulatory challenges is essential."
Global Perspectives on Hydrogen Adoption
The adoption of hydrogen as an energy source is a topic of significant global interest. Countries are increasingly recognizing hydrogen's potential to address energy needs, mitigate climate change, and stimulate economic growth. Its versatility allows it to be integrated into various sectors, such as transportation, industry, and power generation. As nations strive to meet their climate goals, the strategic deployment of hydrogen can serve as a keystone for sustainable development.
One major benefit of hydrogen is its capability for storage and transport, making it a critical element in balancing intermittent renewable energy sources like solar and wind. By harnessing hydrogen, countries can not only diversify their energy mix but also enhance energy security. The comparative ease of adapting existing infrastructures to hydrogen can facilitate its rapid integration into current energy systems. Moreover, hydrogen can aid in decarbonizing industries that are traditionally hard to electrify, such as steel production and heavy freight.
However, the journey towards hydrogen adoption is complex and entails addressing several considerations. These include economic factors, regulatory frameworks, and technological advancements. Understanding how different nations approach hydrogen is essential for disseminating best practices and fostering collaborative efforts.
Case Studies from Leading Countries
Leading countries are at the forefront of hydrogen adoption, each pursuing unique strategies to integrate hydrogen into their energy ecosystems.
- Germany: Germany is investing heavily in hydrogen technology as part of its " Strategy". The government aims to produce green hydrogen through electrolysis powered by renewable sources. This initiative positions Germany as a leader in the European hydrogen market and focuses on developing a robust hydrogen infrastructure.
- Japan: Japan has made substantial investments in hydrogen since the 2011 Fukushima disaster. The country's focus on hydrogen as a way to diversify its energy portfolio is reflected in its "Basic Hydrogen Strategy". Incentives for hydrogen fuel cells and infrastructure development are key components of this strategy.
- South Korea: South Korea is implementing a national hydrogen roadmap that aims to increase hydrogen's role in transportation and energy. The government plans to manufacture hydrogen vehicles and develop associated refueling stations.
These case studies illustrate the diverse pathways countries can take to harness hydrogen's potential, reflecting their specific energy needs and policy priorities.
International Cooperation and Initiatives
International cooperation is essential for advancing hydrogen adoption globally. Joint initiatives can facilitate knowledge sharing and accelerate the development of hydrogen technologies. A few noteworthy endeavours include:
- The Hydrogen Council: A global initiative aimed at promoting hydrogen as a key player in the energy transition. It brings together companies from various sectors to collaborate on strategic projects and initiatives.
- Mission Innovation: An international initiative to accelerate public and private clean energy innovation. Hydrogen has been identified as a key focus area for research and development across member countries.
- Hydrogen Energy Ministerial Conference: This gathering of government officials focuses on sharing knowledge and experiences in promoting hydrogen technologies. The conference aims to create synergies and increase global awareness about hydrogen's potential.
International collaboration not only strengthens hydrogen’s market presence but also promotes research and development, laying the groundwork for a global hydrogen economy.
Overall, understanding global perspectives on hydrogen adoption is critical. Such insights guide practical applications and innovative solutions needed to overcome challenges and seize opportunities in the hydrogen landscape.
Future Trends in Hydrogen Technology
The importance of future trends in hydrogen technology cannot be overstated, as they hold the key to unlocking massive potential for clean energy solutions. As the global community shifts towards sustainability, hydrogen is increasingly recognized as a crucial element in that transition. This section will explore innovations in production and emerging applications in various sectors, revealing how hydrogen technology can reshape our energy landscape.
Innovations in Hydrogen Production
In recent years, innovations in hydrogen production have gained substantial momentum. Traditional methods like steam methane reforming are transitioning towards more sustainable practices such as water electrolysis. Advanced electrolyzers capitalize on renewable energy sources, significantly reducing greenhouse gas emissions. The following are notable innovations:
- Proton Exchange Membrane (PEM) Electrolysis: This technology allows for efficient electrolysis even at small scale installations. PEM electrolyzers are compact, offer high purity hydrogen output, and respond rapidly to fluctuating energy supply.
- High-Temperature Electrolysis (HTE): This technique utilizes heat sources, such as nuclear energy, to enhance the efficiency of hydrogen production. The coupling of heat and electricity enables higher efficiency rates which could alter our approach to energy generation.
- Biological Hydrogen Production: Research continues into microbial methods that utilize bacteria and algae to convert organic matter into hydrogen. Such processes represent an untapped frontier, potentially leading to low-cost, sustainable hydrogen without high energy inputs.
These innovations not only streamline hydrogen production but also enhance its viability as a mainstream energy carrier. The financial implications are significant as cost-effective production may lead to larger adoption rates, influencing energy markets worldwide.
Emerging Applications in Transportation and Industry
The versatility of hydrogen fuels numerous potential applications in both transportation and industrial sectors. As concerns over fossil fuel reliance and carbon emissions grow, the following applications stand out:
- Fuel Cell Electric Vehicles (FCEVs): Companies such as Toyota and Hyundai are already producing commercial fuel cell vehicles. Zero-emission and long-range capabilities make these vehicles an appealing alternative to conventional electric vehicles.
- Hydrogen-Powered Trains and Buses: In countries like Germany and Japan, initiatives to power public transport with hydrogen show promise. These modes of transportation can serve as models for future urban mobility, showcasing reduced emissions.
- Industrial Applications: Industries, particularly those with high-energy demands like steel and chemical production, are exploring hydrogen as a cleaner feedstock. This shift could notably decrease overall carbon footprints in manufacturing processes.
- Power-to-X Technologies: Hydrogen can be synthesized from excess renewable energy and subsequently converted into synthetic fuels or chemicals. This integration supports both energy storage and expands hydrogen's use beyond direct fuel applications.
The transition to hydrogen is not just about energy; it is about fostering sustainable growth across multiple sectors.
Concluding Remarks
The concluding section serves as a critical synthesis of the information presented throughout the article. It emphasizes the pressing significance of hydrogen as a pivotal element in the contemporary energy landscape. Hydrogen's potential as a sustainable energy carrier aligns with the global push towards carbon-neutral alternatives. Understanding its multifaceted nature is essential not only for researchers and policymakers but also for stakeholders across various industries.
Reassessment of Hydrogen's Role
In recent years, hydrogen's role has evolved. Once seen primarily as a niche element for specialized applications, it is now recognized as a key component in achieving energy independence. As nations strive to reduce greenhouse gas emissions, hydrogen offers an alternative that can bridge gaps where traditional renewables may falter, such as during periods of low solar or wind output. The versatility of hydrogen, being usable in fuel cells, internal combustion engines, and as an energy storage solution, necessitates a thorough reassessment of its place in the future energy mix.
Call for Continued Research and Development
Advancements in hydrogen technologies are essential. Investing in research can address existing challenges related to production efficiency, storage solutions, and economic viability. Continued innovation is required to reduce costs associated with hydrogen production and to develop robust infrastructure for distribution and usage.
"Without ongoing R&D efforts, hydrogen's potential to serve as a cornerstone of sustainable energy systems may remain unrealized."
It is imperative to foster collaborative efforts between governments, industries, and academic institutions. By leveraging their collective expertise and resources, we can uncover new methods and applications that will transform hydrogen from a concept into a palpable reality in our global energy future.