Exploring Invitrogen Oligos in Molecular Biology
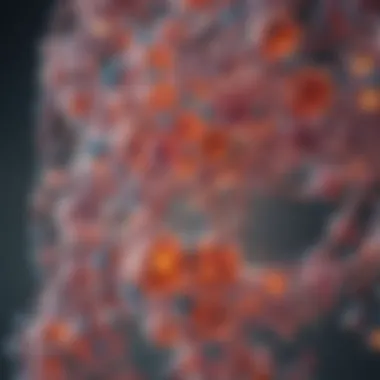
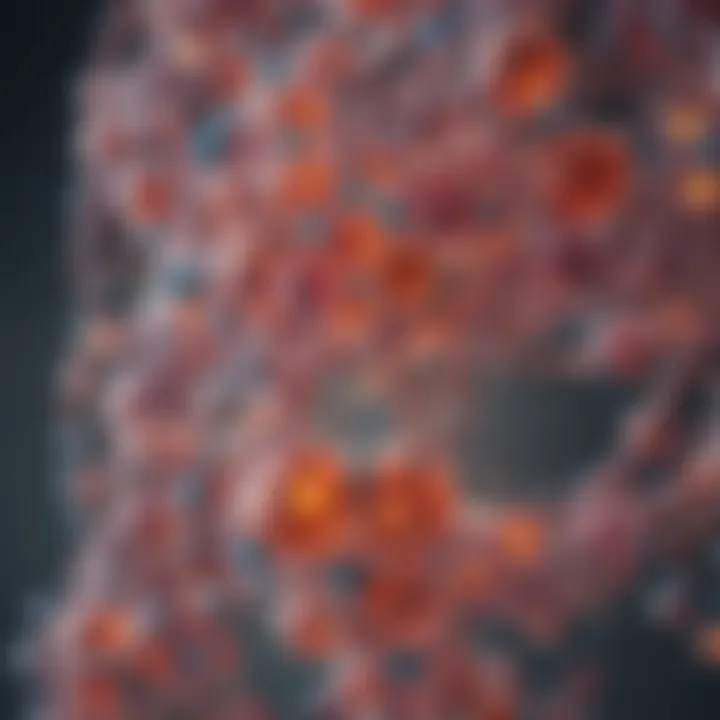
Intro
In the realm of molecular biology, the use of oligonucleotides plays a pivotal role in shaping research methodologies and experimental outcomes. Invitrogen oligos are a prominent choice for many researchers due to their high quality and reliability. This section aims to provide a foundational understanding of how these oligos function in vitro, emphasizing their synthesis, design, and practical applications in laboratory settings. Research highlights the multifaceted benefits of utilizing Invitrogen oligos, making them indispensable in a variety of experimental contexts.
Research Highlights
Key Findings
Investigating the functionality of Invitrogen oligos reveals crucial insights into their applications. These oligos are designed for versatility, allowing them to serve as primers, probes, or even in RNA interference assays. Their synthesis is based on solid-phase chemistry, which ensures a high degree of purity. This purity is vital for experiments requiring specific binding interactions. Studies indicate that the optimization of oligo design directly correlates with experimental success, leading to more accurate results.
Implications and Applications
The implications of using Invitrogen oligos in research are expansive. They enhance both the specificity and sensitivity of various assays, including quantitative PCR and sequencing. Moreover, these oligos are essential in the field of gene editing; for instance, in CRISPR applications, where precision is critical. The utilization of these oligos thus promotes advancements in genetic understanding and manipulation, which is essential for both academic investigations and therapeutic developments.
"The precision of oligonucleotide design is a foundational element in the success of molecular assays, underscoring the importance of products like Invitrogen oligos in scientific research."
Methodology Overview
Research Design
When conducting research utilizing Invitrogen oligos, it is imperative to have a well-structured research design. This includes defining the objectives, formulating hypotheses, and selecting appropriate methodologies that align with the goals of the study. Considerations must be made regarding the specific oligo applications, whether for amplification, detection, or modification of nucleic acids.
Experimental Procedures
The experimental procedures involve several critical steps, including:
- Design and Order: Researchers must design oligonucleotides that fit their experiment's specific needs. This often involves the use of software tools for optimal sequence selection, addressing melting temperature, and specificity issues.
- Synthesis: Invitrogen provides a streamlined process for oligo synthesis, ensuring high yield and quality. Most orders are processed quickly, making them readily available for research applications.
- Validation: After obtaining the oligos, researchers conduct validation studies to confirm performance. This often involves running control experiments to establish baselines for comparison.
In summary, the careful integration of Invitrogen oligos in research methodologies significantly impacts experimental outcomes. Their applications in molecular biology extend across numerous fields, enhancing the quality and reliability of data derived from various biological inquiries.
Intro to Invitrogen Oligos
The utilization of oligonucleotides, specifically those synthesized by Invitrogen, plays a crucial role in the realms of molecular biology and genetic research. Understanding the unique characteristics and applications of these oligos is essential for any researcher or practitioner in the field. By delving into this introductory section, we will uncover the fundamental components that define Invitrogen oligos and the myriad ways they can enhance research efficacy.
Definition and Overview
Invitrogen oligos refer to short strands of nucleic acids, specifically DNA or RNA, that are synthesized for various research applications. These nucleotides carry sequences that are designed to be complementary to specific target nucleic acids. The synthesis of these oligos is a precise process that allows for customization according to the requirements of particular experiments. Oligos can vary dramatically in length, sequence, and modifications, making them versatile tools for numerous applications within molecular biology.
Amongst the features that define Invitrigen oligos are their Intended use in polymerase chain reaction (PCR) protocols, gene expression analyses, and the burgeoning realm of gene editing technologies such as CRISPR.
Importance in Molecular Biology
The impact of Invitrogen oligos cannot be overstated. They serve as foundational elements in various molecular and cellular biology techniques. The pertinence of oligos lies primarily in their ability to bind specifically to target sequences, enabling precise modification, analysis, and amplification of genetic material. This specificity can significantly enhance the accuracy of experiments.
Their applications transcend basic biological research. For instance, in clinical settings, Invitrogen oligos facilitate the development of diagnostic assays, therapies, and genetic testing. This versatility demonstrates not only their importance in experimental design but also their potential to drive innovation in diagnostics and treatment strategies.
Synthesis of Invitrogen Oligos
The synthesis of Invitrogen oligos is an essential aspect of their utility in molecular biology. Synthesizing oligonucleotides allows researchers to create specific sequences tailored for diverse experimental applications. This process is critical because the properties and functionality of the oligos depend heavily on their chemical structure and purity. It influences how effectively the oligos perform in procedures like polymerase chain reactions, gene cloning, and other analyses.
In this section, we will discuss the chemical synthesis process, followed by the quality control measures employed to ensure that the oligos meet the high standards required for scientific research.
Chemical Synthesis Process
The chemical synthesis of oligonucleotides generally employs solid-phase synthesis techniques. This approach allows for the automated production of oligos, leading to high-throughput capabilities. The synthesis starts with attaching a nucleoside to a solid support. During the process, various chemical reagents are added to elongate the oligonucleotide chain in a stepwise manner, creating sequences from a predetermined design.
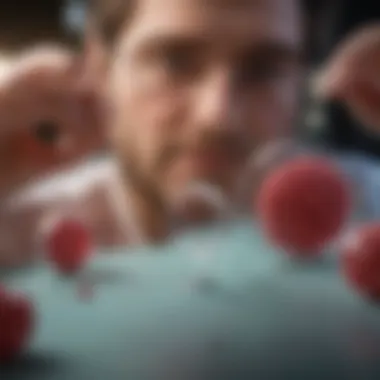
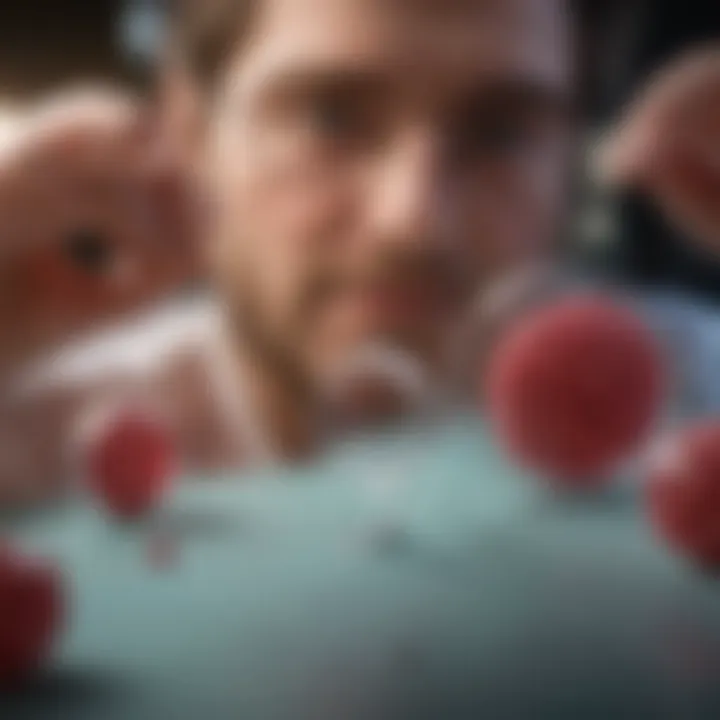
Key factors in this process include the choice of nucleosides, coupling efficiency, and protection strategies for reactive groups. Optimizing these factors directly contributes to the yield and purity of the final product. Ultimately, successful chemical synthesis can result in high-quality oligonucleotides ready for diverse applications in-vitro.
Quality Control Measures
Quality control measures are fundamental in the synthesis of Invitrogen oligos to ensure that the resulting products meet stringent criteria for use in scientific research. There are three primary quality control measures: purity assessment, size verification, and functional analysis. Each of these contribute uniquely to the evaluation process.
Purity Assessment
Purity assessment is crucial to determine the percentage of the desired oligo relative to any impurities that may interfere with experimental outcomes. A typical characteristic of purity assessment is its reliance on techniques such as high-performance liquid chromatography (HPLC) and mass spectrometry. These methods provide quantitative data regarding the purity level of synthesized oligonucleotides.
Purity assessment is a beneficial choice for this article because it directly influences experimental reliability. The unique aspect of this method is that it can detect minute impurities that may not be visible to conventional techniques. The advantages of purity assessment are clear, as higher purity often correlates with better performance in applications such as PCR and cloning.
Size Verification
Size verification ensures that the synthesized oligos match the intended length specified during design. A significant characteristic of size verification is the use of capillary electrophoresis or gel electrophoresis, which enable precise measurement of oligonucleotide lengths. This contributes to validating experimental setups, where specific sizes are critical.
Size verification is important for this article as it ensures that every oligo is appropriate for its intended use. The unique feature of size verification is its ability to distinguish between oligos of similar lengths, which minimizes the risk of experimental errors. The advantages include enhanced accuracy in experimental outcomes and a reduced likelihood of non-specific bindings.
Functional Analysis
Functional analysis assesses the biological activity of the synthesized oligos in relevant assays. This is often done through techniques such as hybridization studies and downstream applications. A key characteristic of functional analysis is its role in evaluating whether the oligos perform as expected within biological systems.
This analysis is a beneficial element for this article because it provides insight into the effectiveness of the oligos in practical scenarios. The unique aspect of functional analysis lies in its application to various workflows, from basic research to clinical outputs. The advantages include confirming that the oligos not only exist at the correct sequence and length but also function effectively in their respective roles.
Design Principles of Oligonucleotides
The design principles of oligonucleotides, particularly those produced by Invitrogen, play a crucial role in the efficacy and specificity of molecular biology applications. Understanding how to design these sequences is essential for researchers, as it affects the success of experiments and the reliability of data generated. The factors include sequence design considerations as well as the software tools that can assist in creating optimal oligonucleotide sequences.
Sequence Design Considerations
Specificity
Specificity is a vital characteristic of oligonucleotide design, aimed at ensuring that the oligos bind exclusively to their target sequence without associating with non-target sequences. This is important for experiments such as PCR, where mispriming can lead to false positives. A high degree of specificity enhances experimental accuracy, making it a beneficial choice when designing oligonucleotides.
The unique feature of specificity is its relation to the melting temperature (Tm) of the oligo, which should be matched to the target sequence conditions. The advantages of high specificity include reduced background noise and higher signal-to-noise ratios, ensuring clearer results in experiments.
GC Content
GC content refers to the percentage of guanine (G) and cytosine (C) nucleotides in an oligonucleotide sequence. This aspect is critical as GC pairs form three hydrogen bonds compared to the two hydrogen bonds formed by adenine (A) and thymine (T) pairs. Therefore, oligonucleotides with higher GC content tend to have increased stability and melting temperatures, which is beneficial for many in vitro applications.
A key consideration when optimizing GC content is finding a balance that provides stability without compromising specificity. While high GC content can be advantageous, excessive GC content may lead to unwanted secondary structures. Thus, careful consideration is necessary in the context of the specific application.
Secondary Structures
Secondary structures refer to the configurations that oligonucleotides can adopt, such as hairpins or loops, due to intramolecular bonding. The presence of these structures can significantly impact the performance of oligonucleotides in various applications like PCR and sequencing. A major concern is that secondary structures can hinder binding efficiency, affecting the overall results of an experiment.
The key characteristic of secondary structures is their dynamic nature, which means they can change based on different conditions, such as temperature and ion concentration. While secondary structures can sometimes be advantageous by enhancing specific interactions or creating stability, generally avoiding them is recommended to enhance the oligos' performance in practical applications.
Software Tools for Design
Designing effective oligonucleotides requires not only a firm grasp of fundamental principles but also access to specialized software tools. These tools provide algorithms and resources that can aid in selecting optimal sequences, calculating Tm, analyzing secondary structures, and ensuring specificity. Some popular software options include Primer3, OligoCalc, and Integrated DNA Technologies’ (IDT) Oligo Analyzer.
Utilizing these tools can greatly facilitate the design process. Users can input desired parameters and receive recommendations for sequences that fit specific criteria. Additionally, many of these platforms offer simulation features to predict how designed oligos will behave in various conditions, adding an extra layer of reliability to the designs.
Applications of Invitrogen Oligos
The applications of Invitrogen oligos represent a critical dimension in molecular biology. These oligonucleotides facilitate various experimental techniques, enhancing precision and effectiveness in research. Their versatility spans a wide range of applications, including polymerase chain reaction (PCR), gene expression analysis, cloning, and gene editing. As such, understanding their applications is paramount for researchers aiming to innovate within their fields.
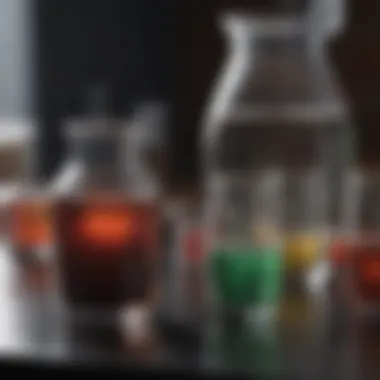
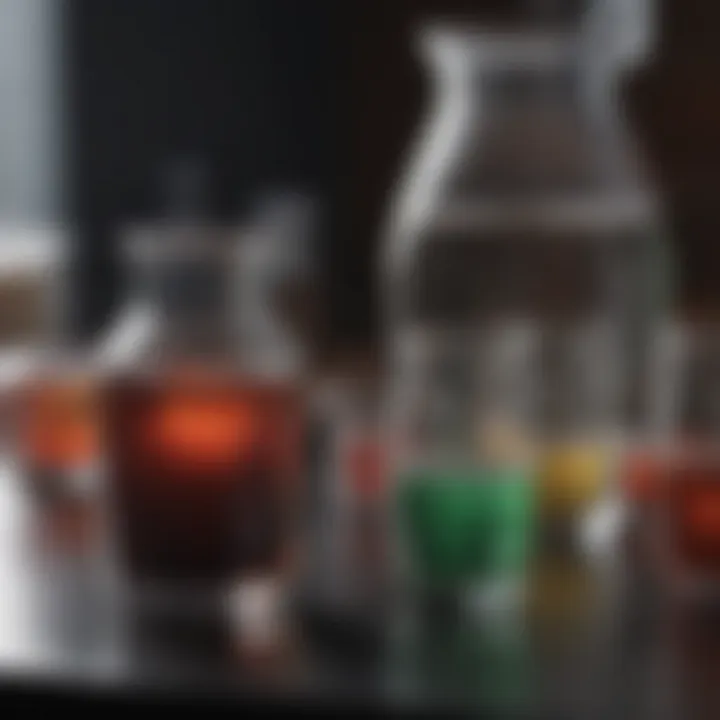
PCR and Amplification Techniques
Polymerase chain reaction (PCR) is a fundamental technique that amplifies specific DNA sequences. It requires precise primers, which are often designed and synthesized as Invitrogen oligos. The ability of these oligos to ensure specificity during amplification is crucial. A well-designed primer allows for efficient binding to target sequences, leading to exponential amplification. This technique is widely used in diagnostics and genetic research. The precision offered by Invitrogen oligos thus plays a significant role in the reliability of results in PCR applications.
Gene Expression Analysis
Gene expression analysis is essential for understanding cellular function and regulation. This analysis often employs specific oligos to probe and quantify the expression levels of genes.
qPCR
Quantitative polymerase chain reaction (qPCR) is a powerful technique that quantifies nucleic acids through fluorescence detection. This method is favored because it allows for real-time monitoring of the amplification process, offering a more accurate assessment of gene expression levels. qPCR involves the use of Invitrogen oligos as primers, which contribute significantly to the specificity and efficiency of amplification. The primary characteristic that sets qPCR apart is its ability to provide quantitative data, making it a popular choice for researchers needing precise measurements. However, qPCR requires careful optimization to avoid issues related to primer efficiency, which can skew results if not adequately addressed.
RT-PCR
Reverse transcription polymerase chain reaction (RT-PCR) is another method instrumental in gene expression analysis. It converts RNA into complementary DNA (cDNA), enabling the study of gene expression at the transcriptional level. The key benefit of RT-PCR lies in its capacity to detect and quantify RNA, which can be particularly valuable in studies assessing gene regulation under various conditions. The unique feature of RT-PCR is its ability to analyze transient gene expression, providing insights that static measurements cannot. However, this method may also face challenges related to the stability of RNA, which can impact results if not properly handled.
Cloning and Gene Editing
Cloning and gene editing are transformative applications in molecular biology. Both processes rely heavily on the use of oligonucleotides for various steps, from designing vectors to introducing changes in DNA sequences.
CRISPR Applications
The CRISPR technology has revolutionized gene editing by allowing targeted modifications within genomes. The design of CRISPR guide RNAs typically involves the synthesis of specific oligos, which direct the Cas9 protein to the intended DNA locus. This method's main advantage is its specificity and efficiency, which enable precise edits that can lead to significant advancements in genetic research. However, off-target effects remain a critical concern that researchers must address, as they can result in unintended genetic modifications.
Gene Targeting
Gene targeting, another area within cloning and gene editing, utilizes Invitrogen oligos to create homology arms for genomic integration. This method is beneficial in creating knockout models or introducing specific mutations. The ability to precisely target specific genes offers researchers invaluable tools for studying gene function and interaction. Nevertheless, it requires sophisticated design and validation to ensure successful integration and expression.
"The applications of Invitrogen oligos are essential in enhancing molecular biology research and innovation."
In summary, the applications of Invitrogen oligos are foundational to the progress of molecular biology techniques. These oligos are indispensable tools that enhance the precision, specificity, and scalability of genetic research.
Technical Considerations for Oligo Usage
Understanding the technical considerations for oligo usage is essential for ensuring the success of various molecular biology applications. The precise handling of oligos can drastically influence experimental outcomes, such as specificity, efficiency, and overall reproducibility. Moreover, paying attention to the technical aspects can help mitigate common challenges, promoting more reliable results. Factors such as concentration, dilution, and optimization of reaction conditions play vital roles in utilizing Invitrogen oligos effectively.
Concentration and Dilution Factors
Concentration and dilution are primary factors that affect the performance of oligonucleotides in experiments. The proper oligo concentration is crucial as it can directly influence binding efficiency during processes such as PCR or gene expression analysis. If the concentration is too high, it may lead to non-specific binding, resulting in undesirable products. Conversely, a low concentration might not provide sufficient binding to achieve effective amplification.
In practice, researchers should carefully calculate the required oligo concentrations based on the specific requirements of their experimental protocols. Adjusting concentrations may require dilutions in buffer, which further emphasizes the importance of accurate measurement and preparation.
Optimization of Reaction Conditions
Temperature
Temperature is a critical factor in optimizing reaction conditions for oligos. It significantly affects the annealing process during PCR and other applications. The optimal temperature can dictate the specificity of the reaction, aiding in the successful binding of the oligo to the target sequence. It is commonly recommended to run gradient PCR to determine the suitable temperature for specific reactions. While a higher temperature may increase specificity, one should balance it against the need for sufficient duplex formation.
Buffer Composition
Buffer composition is another crucial aspect that influences the performance of oligos in molecular biology. The choice of buffer can impact the overall stability of the oligo-target complex and its interaction during the reaction. For example, Tris-buffered saline (TBS) is widely used due to its effectiveness in maintaining pH and minimizing ion-induced precipitation, which is especially beneficial for oligo interactions. However, the buffer composition must align with the desired reaction, as some compounds may interfere with oligo performance, leading to suboptimal results.
Impact of Secondary Structures
Secondary structures in oligonucleotides can greatly affect their functionality. These structures may form due to complementary sequences within the oligo, which can hinder binding to target sequences. It is essential to analyze the potential secondary structures before conducting experiments to preemptively address any challenges they may introduce. Tools for predicting secondary structures can be beneficial in minimizing these issues, ensuring a higher likelihood of successful reactions. Understanding and controlling the formation of secondary structures should be a priority for researchers working with Invitrogen oligos.
Common Challenges in Using Oligos
The use of Invitrogen oligos offers numerous advantages, yet researchers face distinct challenges when working with these tools. Addressing challenges is crucial for maximizing the efficacy of oligonucleotides in various applications. Understanding these issues can inform better practices and enhance the reliability of results in molecular biology. Key challenges include non-specific binding and degradation, both of which can significantly impact experimental outcomes.
Non-Specific Binding Issues
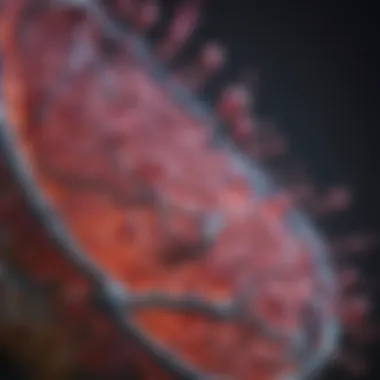
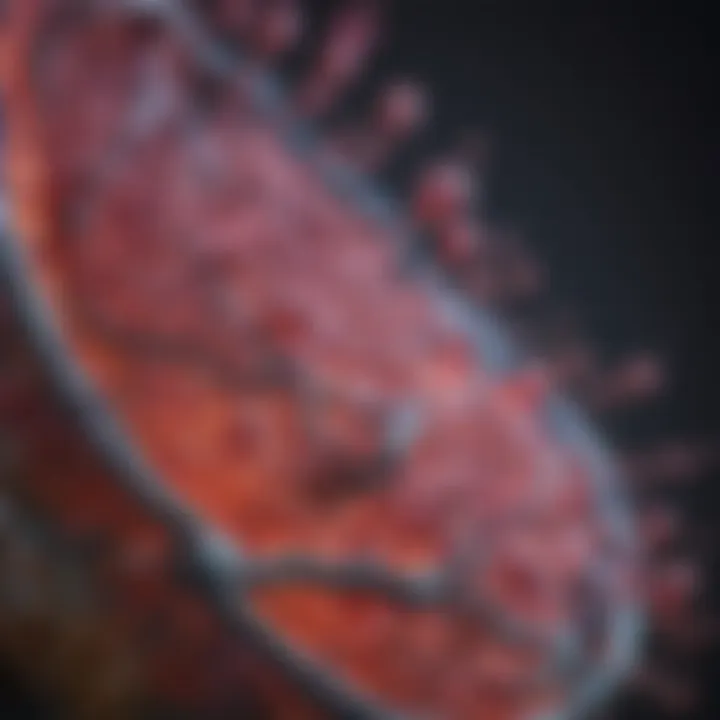
Non-specific binding refers to the unintended interaction between oligos and targets or other molecules in a reaction. This phenomenon can skew results, leading to false positives or ambiguous data. Addressing non-specific binding is critical for achieving accurate and reproducible results in experiments.
One contributing factor is the design of oligonucleotides. Oligos with insufficient specificity may anneal to similar sequences rather than the intended target. This scenario is exacerbated in reactions with complex mixtures, where oligos may hybridize with unintended sequences. To mitigate these issues, researchers should fine-tune oligo design, paying careful attention to sequence uniqueness.
Additionally, reaction conditions play a significant role. Factors such as temperature and salt concentration can influence binding characteristics. Researchers must optimize these conditions to reduce background noise and increase the signal-to-noise ratio in their assays. In practical terms, using high-fidelity DNA polymerases and enhancing reaction buffer compositions may further minimize this challenge.
Degradation and Stability
Degradation of oligos can have detrimental effects on research. Oligonucleotides are susceptible to hydrolysis and nucleolytic degradation, which can compromise the stability and integrity of experimental results. Two major aspects of degradation, chemical stability and biological degradation, require careful consideration.
Chemical Stability
Chemical stability refers to the oligos' ability to resist breakdown under specific conditions. Higher chemical stability helps protect the oligos from unwanted reactions that can lead to reduced functionality. A key characteristic of chemically stable oligos is their phosphorothioate modifications. This unique feature imparts resilience against exonuclease activity and makes them a popular choice for various applications.
The advantages of high chemical stability are clear. Researchers can store such oligos for extended periods without significant loss of activity. However, it is essential to monitor storage conditions and avoid prolonged exposure to extreme temperatures or pH concentrations.
Biological Degradation
Biological degradation pertains to the breakdown of oligonucleotides by biological agents such as enzymes. This is particularly relevant in in vitro applications where enzymes like nucleases can degrade sensitive oligos. A notable characteristic of biological degradation is its swift action. This can lead to rapid loss of oligo effectiveness during experiments.
To tackle this challenge, professionals often explore methods to enhance the resistance of oligos to biological degradation. Modifying the oligos, such as using locked nucleic acids or introducing modifications at key positions, may enhance their stability against enzymatic activity. However, it's vital to balance modifications with potential effects on oligonucleotide functionality.
In summary, understanding and addressing the common challenges of using oligos significantly enhance their utility and effectiveness in molecular biology research.
The Future of Oligonucleotide Technologies
The field of oligonucleotide technologies is rapidly evolving. This section discusses its relevance in molecular biology and the anticipated future developments. Oligonucleotides play a central role in various applications, making the innovations in this domain particularly significant. The advancements may enhance their efficacy in research and allow researchers to tackle complex biological questions more effectively.
Emerging Trends in Oligo Design
The design of oligonucleotides is becoming increasingly sophisticated. Several emerging trends are shaping the future of oligo design. One notable trend is customizability. Researchers can create oligonucleotides tailored specifically for their experiments. This is essential for applications like gene editing and diagnostics.
Another trend is the increased use of software for predictive modeling. Advanced algorithms and machine learning technologies are being employed to predict the behavior of oligonucleotides in biological systems. This helps in optimizing their design for better specificity and reduced off-target effects.
Additionally, the focus on eco-friendly synthesis methods is gaining traction. Sustainable practices in the synthesis of oligos can minimize the environmental impact. The trend also reflects a growing awareness of corporate responsibility in the scientific community.
Innovations in Synthesis Techniques
Synthesis techniques for oligonucleotides are also experiencing significant innovations. New methodologies are being developed to enhance the speed and efficiency of synthesis. For instance, solid-phase synthesis remains a common approach but is increasingly being substituted with more efficient strategies, like microfluidics.
Microfluidics allow for better control of reagent flow and improved reaction conditions. This can lead to higher yields and purity of the synthesized oligonucleotides. Moreover, new chemical modifications are being explored to create oligos with novel properties. These modifications can improve stability and binding affinity, thus expanding their potential applications.
Furthermore, there is an increasing focus on large-scale synthesis. As research demands grow, the ability to synthesize oligonucleotides in bulk is crucial. Companies are investing in automated platforms to streamline processes and ensure quality.
In summary, the future of oligonucleotide technologies seems promising. With ongoing advancements in design and synthesis, researchers can expect more robust and efficient tools for various molecular biology applications.
Culmination
The conclusion of this article emphasizes the significance of Invitrogen oligos in the landscape of molecular biology research. It is crucial to summarize the key points discussed throughout the article as these aspects are tied to the understanding and practical applications of oligonucleotides in a laboratory setting.
The Importance of Invitrogen Oligos cannot be overstated. Their versatility in various applications, including PCR, gene expression analysis, and cloning, speaks to their essential role in advancing research. Additionally, attention to the design principles and synthesis techniques enhances their utility, ensuring that researchers obtain high-quality oligos tailored for specific experiments.
Summary of Key Points
- Invitrogen oligos are fundamental in modern molecular biology, supporting a variety of applications, such as PCR, gene expression studies, and gene editing.
- The synthesis process and quality control measures ensure the integrity and effectiveness of these oligonucleotides.
- Design principles play a vital role in maximizing specificity and minimizing non-specific binding, which are crucial for successful experiments.
- Understanding technical considerations, such as dilution factors and reaction conditions, can significantly improve experimental outcomes.
- Common challenges exist in the use of oligos, including stability and degradation, which require careful management.
Implications for Future Research
The implications of utilizing Invitrogen oligos extend well into the future of research. As new applications emerge, the demand for improved synthesis techniques and innovative design strategies is anticipated to grow. Researchers must remain informed about advancements in oligonucleotide technology, which may lead to improved methodologies.
Potential future directions could include:
- Development of oligos with enhanced stability, reducing degradation during experiments.
- Exploration of novel applications in personalized medicine and synthetic biology, expanding the scope of oligonucleotide use.
- Continued improvement in computer-assisted design tools to facilitate more efficient and accurate oligonucleotide crafting.