Hydrogen to Electricity Conversion: Innovations Explored
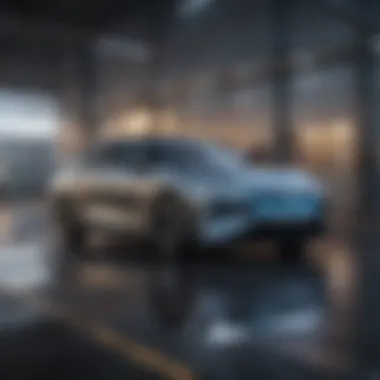
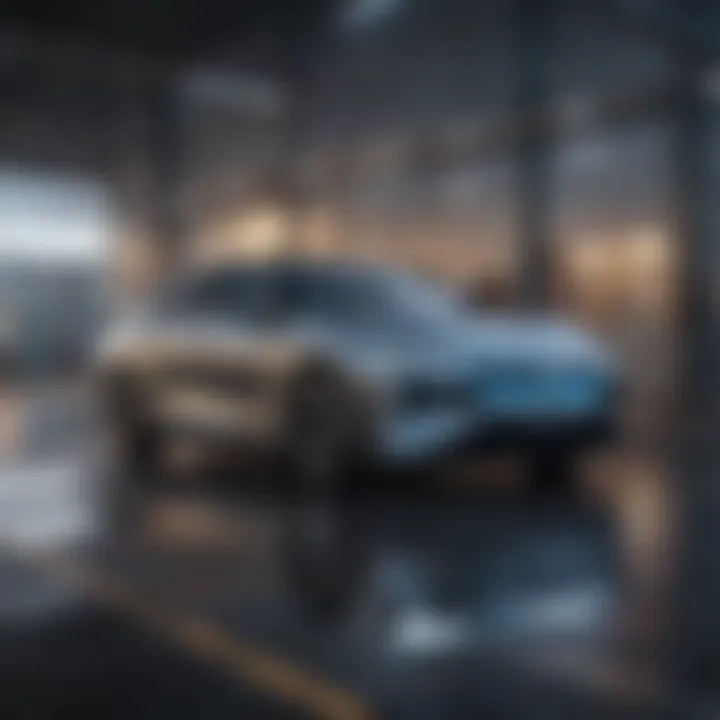
Intro
The shift toward renewable energy sources has accelerated in recent years. Hydrogen has emerged as an important player in this field. The conversion of hydrogen into electricity offers a unique solution to address global energy demands while contributing to environmental sustainability. Understanding how hydrogen can be transformed into electrical energy is crucial as it leads to cleaner alternatives to traditional fossil fuels. This article seeks to explore the mechanisms, methodologies, and implications of this conversion process.
Hydrogen can be produced using various methods, including electrolysis and steam methane reforming. However, the focus here will be predominantly on the advanced techniques employed to convert hydrogen into electricity. Fuel cells, as one prominent technology, provide a pathway through which this conversion can occur efficiently. Fuel cells combine hydrogen and oxygen to produce electricity, with only water as a byproduct, reducing harmful emissions significantly.
In this article, we will delve deeper into the specific conversion techniques, examine their efficiency rates, and discuss the overall benefits associated with hydrogen energy systems. We will also address the current research landscape and future direction of hydrogen technology and its role in achieving global sustainability goals.
The following sections will outline key findings, explore the associated methodologies, and analyze their implications for various stakeholders—including policymakers, researchers, and industry practitioners.
Understanding Hydrogen as an Energy Source
The exploration of hydrogen as an energy source is both timely and significant. Hydrogen is viewed as a clean alternative to traditional fossil fuels. Its potential to reduce greenhouse gas emissions is central to discussions about sustainable energy solutions. Additionally, the increasing urgency for energy diversification makes understanding hydrogen's benefits more crucial.
Hydrogen stands out due to its unique properties. It is the lightest element and can store energy effectively. When used to produce electricity, it generates only water vapor as a byproduct. This characteristic aligns hydrogen with global sustainability goals. Moreover, the adaptability of hydrogen across various sectors strengthens its role in a future energy landscape.
In evaluating hydrogen as an energy source, several aspects must be considered. These include its production methods, efficiency, storage, and transportation concerns. Each of these elements contributes to the viability of hydrogen in the energy market. Thus, an in-depth understanding is fundamental for stakeholders across research, industry, and policy realms.
Chemical Properties of Hydrogen
Hydrogen's chemical properties play a pivotal role in its application as a source of energy. It is highly flammable, allowing for easy combustion in reaction processes. However, its low boiling point presents challenges for storage and distribution. Additionally, hydrogen forms various compounds with other elements, influencing its production methods. Understanding these properties is essential for developing technologies that leverage hydrogen effectively.
Hydrogen Production Methods
The methods of producing hydrogen are diverse and reflect different technological advancements. Key production methods include steam methane reforming, electrolysis, and biomass gasification. Each method presents its advantages and limitations in terms of efficiency, sustainability, and carbon footprint.
Steam Methane Reforming
Steam methane reforming (SMR) is the most common method for hydrogen production. It involves reacting methane from natural gas with steam, resulting in hydrogen and carbon dioxide. One key characteristic of SMR is its high efficiency, making it a cost-effective choice for large-scale hydrogen production. However, the process emits significant carbon dioxide, raising environmental concerns. Thus, while SMR is prevalent, its sustainability impacts remain a critical topic for consideration.
Electrolysis
Electrolysis utilizes electricity to split water into hydrogen and oxygen. This process is increasingly gaining attention due to its potential for using renewable energy sources. A key feature is the ability to produce hydrogen without carbon emissions when powered by wind or solar energy. Despite its advantages, electrolysis currently faces challenges in terms of energy efficiency and cost. These factors must be addressed to make it a more viable option for mass hydrogen production.
Biomass Gasification
Biomass gasification converts organic materials into hydrogen, carbon monoxide, and methane. The process involves heating biomass in a low-oxygen environment. One significant aspect of biomass gasification is its dependence on renewable materials, presenting a more sustainable option. This method not only produces hydrogen but also aids in waste management. However, the scale of production and technical complexities can hinder its widespread adoption. Understanding these dynamics is important for assessing hydrogen's role in future energy systems.
Conversion Technologies of Hydrogen to Electricity
The conversion of hydrogen into electricity is a crucial aspect when considering sustainable energy solutions. This topic encompasses various methods and technologies, each with its unique benefits and challenges. Understanding these technologies can aid in developing efficient energy systems that utilize hydrogen as a clean fuel.
In recent years, technological advancements have made hydrogen conversion methods increasingly viable and attractive. The benefits associated with these methods include high energy efficiency, the potential for grid stability, and versatility in application. Fuel cells and electrochemical conversion methods are at the forefront of this technology.
Technological infrastructure needs to evolve to support widespread hydrogen adoption. This includes enhancing existing energy frameworks and integrating hydrogen into diverse sectors. The efficiency and performance of these conversion technologies can greatly influence their long-term viability.
Fuel Cells: Overview and Mechanism
Fuel cells are devices that convert chemical energy directly into electrical energy. They function by facilitating electrochemical reactions. The simplicity and effectiveness of fuel cells make them prominent in discussions about hydrogen energy. One notable characteristic is their ability to operate at varying scales, from small portable units to large stationary applications.
Types of Fuel Cells
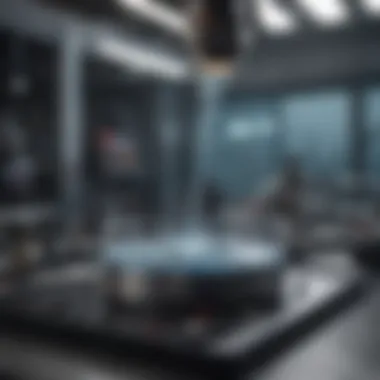
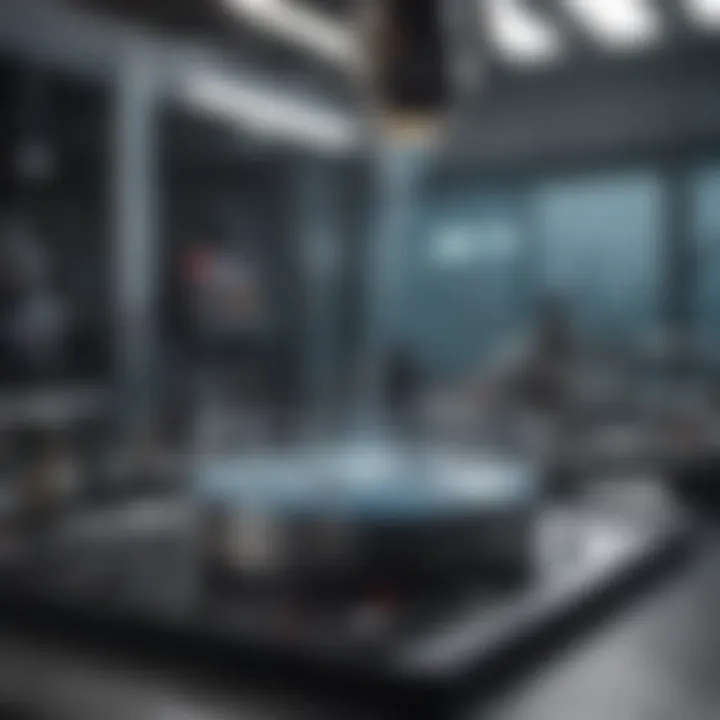
There are multiple types of fuel cells, each with distinct characteristics and applications. Proton Exchange Membrane Fuel Cells (PEMFCs), Solid Oxide Fuel Cells (SOFCs), and Alkaline Fuel Cells (AFCs) represent major fuel cell technologies. PEMFCs are often favored due to their compact size, quick start-up time, and lower operating temperature. This makes them suitable for applications such as transportation and portable power generation.
However, variations like SOFCs offer advantages in terms of efficiency and fuel flexibility, though they typically require higher temperatures and longer start-up periods. The selection of fuel cell type greatly impacts the performance and suitability for specific applications.
Operation of Proton Exchange Membrane Fuel Cells
The operation of Proton Exchange Membrane Fuel Cells involves the use of a solid polymer membrane as an electrolyte. Hydrogen ions pass through this membrane while electrons flow through an external circuit, generating electricity. This mechanism is effective and efficient, making PEMFCs particularly attractive for vehicular applications.
Their compact design and quick responses to varying loads provide considerable advantages in dynamic settings. Nonetheless, they can be sensitive to impurities in hydrogen and moisture levels.
Advantages and Limitations of Fuel Cells
Fuel cells present several advantages, including low emissions, high efficiency, and versatile applications. They can also provide power with minimal noise. However, limitations exist. The cost of fuel cell systems, including components like membranes and catalysts, often remains high. Additionally, challenges in hydrogen storage and infrastructure development can impede broader adoption.
Electrochemical Hydrogen Conversion
Electrochemical hydrogen conversion represents another method for generating electricity from hydrogen. This process encompasses various technologies, notably electrolyzers and fuel cells. Understanding the differences between these systems is vital for evaluating their respective roles in energy applications.
Electrolyzers vs. Fuel Cells
Electrolyzers are used to produce hydrogen by splitting water molecules into hydrogen and oxygen through electrolysis. In contrast, fuel cells convert hydrogen back into electricity. Both systems are valuable but serve different functions in the hydrogen economy.
The key characteristic of electrolyzers lies in their ability to produce hydrogen from renewable energy sources, which can be stored for later use. This enables energy storage solutions, adding flexibility to energy supply. However, the overall efficiency of electrolyzers is often lower than that of fuel cells.
Overall Efficiency Considerations
Efficiency is an important factor in comparing hydrogen conversion technologies. When considering the entire hydrogen lifecycle, from production to conversion, the efficiency of both electrolyzers and fuel cells can impact overall energy system performance. The optimization of these technologies is a continuous area of research.
Factors such as energy input and operational conditions dictate how efficiently each system operates. Therefore, improving the overall efficiency of hydrogen conversion technologies is critical for their future viability in the energy market.
Recent Advancements in Electrochemical Methods
Recent developments in electrochemical methods show promise for enhancing the capabilities of hydrogen conversion. Innovations in materials science, such as advancements in catalysts, can lead to more efficient processes. Ongoing research into systems that operate at lower temperatures and pressures also contributes to the field.
New technologies in battery energy storage can work synergistically with hydrogen systems, boosting efficiency and performance. These advancements are crucial as the energy landscape continues to evolve.
The future of hydrogen conversion technologies hinges on ongoing research and development, which seeks to improve efficiency, reduce costs, and broaden applications.
Performance Metrics of Hydrogen Energy Systems
The evaluation of performance metrics in hydrogen energy systems is crucial for determining their viability and effectiveness in the current energy landscape. As the world shifts towards cleaner energy solutions, optimizing hydrogen conversion into electricity becomes more essential. Performance metrics include energy density, efficiency, and cost-effectiveness, all of which contribute to the overall success of hydrogen as an alternative energy source.
Energy Density and Efficiency
Energy density refers to the amount of energy stored per unit volume or mass. In hydrogen systems, this characteristic plays a significant role. Hydrogen offers a high energy density compared to conventional fuels. This quality means that hydrogen can potentially store more energy in a smaller volume. However, efficiency in converting that stored energy into usable electricity is equally important.
Hydrogen's energy efficiency can vary based on the conversion method employed. Fuel cells, for instance, can achieve high conversion efficiencies but are influenced by the operating conditions. The cumulative efficiency, when integrating production and conversion technologies, also greatly impacts the value proposition of hydrogen energy systems.
Cost-Effectiveness Analysis
Capital Investment Requirements
Capital investment is a key factor in assessing the feasibility of hydrogen energy projects. Initial costs can be substantial due to the infrastructure needed for production, storage, and distribution. The initial investment often determines the speed at which hydrogen technologies can gain traction in the market. A significant characteristic of capital investment in this field is the potential for economies of scale. As production ramps up, costs may decrease, making hydrogen more accessible.
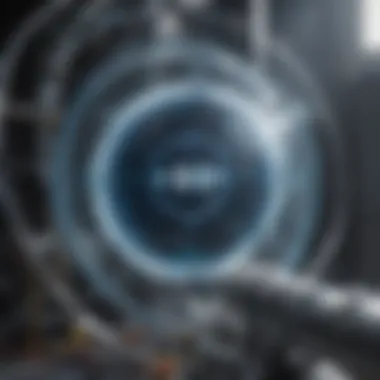
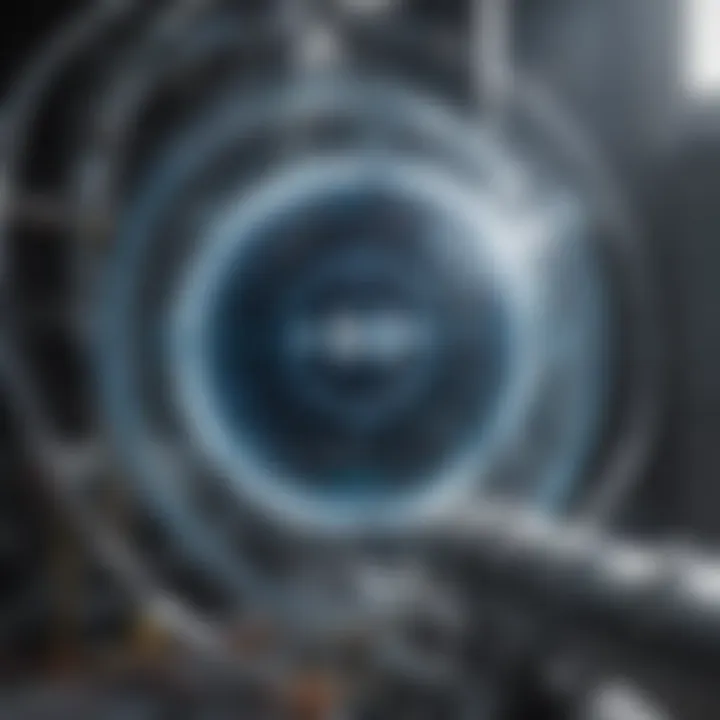
Unique features include the need for specialized equipment and facilities, which can be challenging for new entrants. Nonetheless, early investments can lead to long-term benefits, such as energy independence and supply stability. Given the global shift towards decarbonization, this aspect is increasingly regarded as a vital component for future energy planning.
Operational Costs and Maintenance
In addition to capital investment, operational costs and maintenance also influence the overall economic feasibility of hydrogen systems. Operational costs include expenses related to energy consumption, personnel, and the upkeep of technology. Maintaining high-efficiency systems is essential, yet it can involve ongoing costs that companies must account for.
A notable characteristic of operational costs is the variability depending on the scale of operation. Larger systems might benefit from lower per-unit costs, yet require more sophisticated maintenance protocols. The balance between maintaining operational efficiency and managing costs is critical. While operational expenses can be significant, effective maintenance practices can enhance system reliability, ultimately leading to lower long-term costs.
The performance metrics of hydrogen energy systems are a pivotal area of focus as it influences both investment decisions and environmental outcomes in the shift towards sustainable energy solutions.
In summary, analyzing the performance metrics of hydrogen energy systems reveals essential insights into energy density, efficiency, capital investment requirements, and operational costs. Understanding these metrics allows researchers and professionals alike to make informed decisions as they explore the potential of hydrogen as a mainstream energy source.
Integrating Hydrogen Energy into Existing Systems
Integrating hydrogen energy into existing systems presents a pivotal opportunity in the transition towards clean energy solutions. As energy demands rise globally, the adoption of hydrogen can complement and enhance traditional energy frameworks. The integration helps to leverage the existing infrastructure, providing a pathway that does not require a complete overhaul of current systems. This is particularly critical as governments and organizations look for efficient methods to reduce greenhouse gas emissions while improving energy security.
Hydrogen Blending in Natural Gas Infrastructure
Hydrogen blending involves the introduction of hydrogen into existing natural gas pipelines. This process allows for a gradual shift toward a hydrogen-based economy without extensive modifications to current infrastructure. The key benefit of hydrogen blending lies in its ability to reduce carbon emissions from natural gas usage. By substituting a portion of natural gas with hydrogen, emissions can be diminished, promoting a cleaner energy solution.
However, there are technical considerations in this integration. Hydrogen has different characteristics compared to natural gas, like lower energy content per volume and the potential for embrittlement of pipeline materials. Therefore, engineering solutions and new standards must be established to ensure safe blending practices. This combination of hydrogen and natural gas can significantly contribute to energy diversification and sustainability goals.
Utilizing Hydrogen in Transportation
Hydrogen Fuel Cell Vehicles
Hydrogen Fuel Cell Vehicles (FCVs) represent a significant advance in clean transportation technology. These vehicles convert hydrogen into electricity through a fuel cell process, emitting only water vapor as a byproduct. The key characteristic of FCVs is their efficiency and range compared to battery electric vehicles. They can refuel quickly, similar to conventional gasoline vehicles, enabling longer travel distances. This makes them a sensible choice in regions lacking widespread charging infrastructure.
A unique feature of FCVs is their scalability. As advancements in hydrogen production and storage evolve, the performance and adoption of these vehicles could see substantial increases. The primary advantage of hydrogen fuel cell technology is its potential to contribute toward reducing urban air pollution and reliance on fossil fuels. However, the overall infrastructure for hydrogen fueling stations is still limited, which poses a significant challenge for widespread adoption.
Challenges in Infrastructure Development
The challenges in infrastructure development for hydrogen energy systems are notably intricate. Building the necessary infrastructure for hydrogen production, storage, and distribution requires substantial investment and time. The key characteristic of these challenges stems from the current lack of networked hydrogen refueling stations, which limits accessibility for potential users.
Despite these challenges, investing in hydrogen infrastructure presents both opportunities and benefits. For instance, it can create jobs and boost local economies during the construction and operational phases. Furthermore, expanding hydrogen infrastructure can enhance energy resilience, integrating renewable energy sources into existing systems. However, the upfront costs and regulatory hurdles must be thoroughly addressed to realize a successful transition.
This integration of hydrogen into existing systems exemplifies how innovation in energy can pave the way for a sustainable future.
In summary, leveraging hydrogen within current infrastructures not only promotes sustainability but also offers a multi-faceted approach to meeting energy needs effectively. The exploration of hydrogen blending and fuel cell vehicles further emphasizes its effectiveness, paving the way for a cleaner, more robust energy grid.
Environmental Implications of Hydrogen Use
The exploration of hydrogen as an energy source brings important environmental considerations to the forefront. Hydrogen has potential to reduce carbon emissions significantly when utilized in energy systems. Assessing its impact requires examining carbon footprints and the sustainability of production methods. It is essential to understand how hydrogen can function within the broader context of environmental sustainability.
Carbon Footprint Analysis
The carbon footprint of hydrogen production greatly depends on the method utilized. For instance, steam methane reforming, a common method today, often leads to increased carbon emissions due to the use of fossil fuels. On the other hand, when hydrogen is produced through electrolysis using renewable energy, the carbon footprint can be dramatically reduced. This contrast highlights why methods of hydrogen production must be evaluated critically.
The choice of production method directly influences the environmental benefits hydrogen can provide and is paramount for its future adoption.
Key Characteristics of Carbon Footprint
- Production Method: Different methods yield varying emissions.
- Energy Source for Electrolysis: Using solar or wind energy minimizes carbon footprint.
- Integration with Existing Infrastructure: Influences overall efficacy in emission reduction.
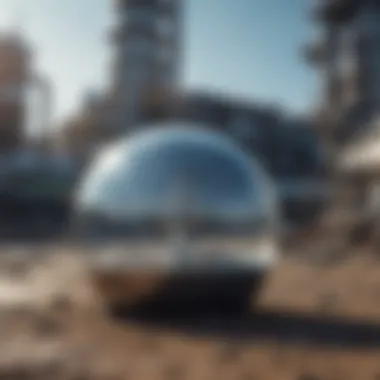
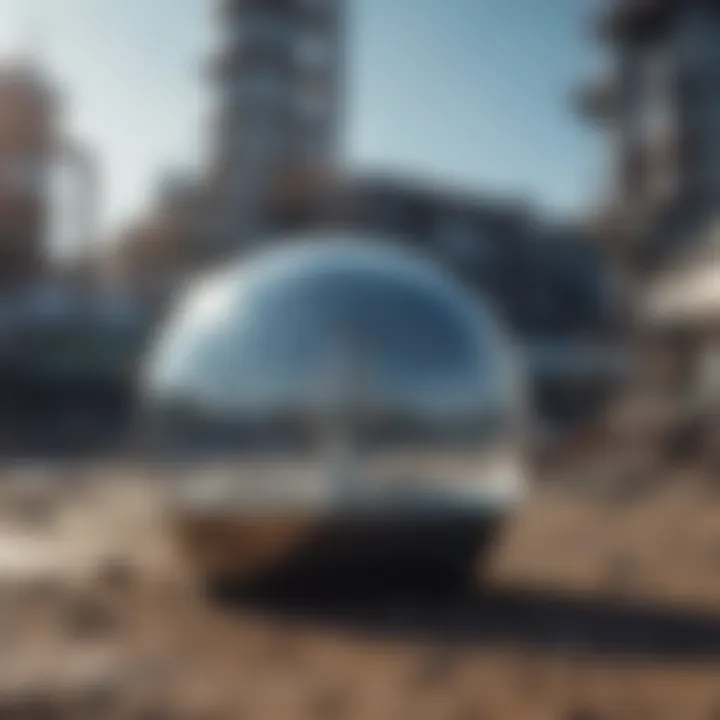
In summary, hydrogen has potential to minimize environmental impact if clean production methods are prioritized.
Sustainability of Hydrogen Production
Sustainability in hydrogen production is vital for its long-term viability as an energy source. This involves understanding the renewable sources employed and their emission profiles. Sustainable hydrogen reduces reliance on fossil fuels and supports green initiative goals.
Assessing Renewable Sources
Understanding renewable sources is key. Solar energy, wind energy, and hydropower can all be harnessed for electrolysis, a method that divides water into hydrogen and oxygen. These sources are considered beneficial in producing clean hydrogen. Key characteristics of these sources include:
- Abundance: Available globally and not regionally restricted.
- Low Emissions: They do not produce greenhouse gases during energy generation.
- Long-term Viability: Can support hydrogen production without depletion.
The unique feature of using renewable sources in hydrogen production is the enhanced reduction of greenhouse gas emissions, making it a popular choice for sustainable energy projects.
Lifecycle Emissions Considerations
Analyzing lifecycle emissions during hydrogen production is crucial. This examines emissions from the entire process, from production through to usage. Key characteristics of lifecycle emissions considerations include:
- Total Emission Scope: This includes direct and indirect emissions involved in the process.
- End-Use Emissions: Evaluating emissions during hydrogen combustion or use in fuel cells.
- Sourcing and Processing: Impacts from sourcing materials for electrolysis or fuel cells matter significantly.
The unique aspect of lifecycle emissions is its comprehensive view of hydrogen’s impact. While hydrogen production might appear clean, the entirety of its sourcing and use can contribute to emissions. This duality represents an advantage as it enables better regulation and optimization of hydrogen systems.
Future Trends in Hydrogen Conversion Technologies
The future trends in hydrogen conversion technologies are crucial in shaping the landscape of renewable energy. As societies become more dependent on sustainable solutions, understanding these trends can enhance efficiency and economic viability. Researchers and industries are actively exploring how to optimize hydrogen energy systems for a greener future. These advancements could significantly affect energy storage capabilities, particularly in addressing peak demand and intermittent resources.
Emerging Research Directions
Nanotechnology Applications
Nanotechnology applications are revolutionizing hydrogen conversion processes. This field focuses on manipulating matter on an atomic and molecular scale, leading to innovative solutions in efficient hydrogen production and storage. A key characteristic of nanotechnology in this context is its ability to improve the surface area contact in chemical reactions, thus enhancing catalytic performance. This is particularly beneficial in fuel cells where highly efficient reactions are necessary.
The unique feature of nanotechnology is the use of nanomaterials, which can lead to lighter and more efficient systems. These materials often display superior electrical conductivity and thermal stability compared to traditional substances. Moreover, their smaller size allows for better absorption of hydrogen, increasing the overall efficiency. However, there are also significant concerns regarding the stability and long-term reliability of these materials in practical applications.
Smart Grid Integration
Smart grid integration represents a transformative approach for hydrogen energy systems. This concept refers to the incorporation of digital technology into the electrical grid, allowing for better management of electricity supply and demand. A prominent characteristic of smart grids is their ability to enable two-way communication between the utility and consumers. This flexibility is crucial for optimizing the use of hydrogen produced from renewable sources such as solar or wind energy.
The unique feature of smart grid integration is its capacity to balance generation and consumption in real-time. By facilitating the storage of excess renewable energy in the form of hydrogen, it can help stabilize energy production amidst fluctuations in renewable sources. Additionally, this technology can enhance the resilience of the energy infrastructure. On the downside, implementing smart grid technologies can be expensive and complex, requiring significant infrastructure investments.
Policy and Economic Factors Influencing Hydrogen Development
Government Incentives
Government incentives play a vital role in accelerating hydrogen development. These incentives may come in the form of tax credits, subsidies, or research grants aimed at reducing the initial costs associated with hydrogen technologies. A key characteristic of these incentives is their potential to lower the financial barriers that companies face when entering hydrogen markets.
The unique feature of government incentives is that they can foster innovation, encouraging the private sector to invest in research and development. Consequently, this can lead to breakthroughs that enhance the efficiency and reduce the costs of hydrogen technologies. However, a reliance on government support can create challenges. For instance, if funding is reduced or discontinued, projects may struggle to sustain themselves, threatening the stability of the industry.
Investment Trends in Hydrogen Technologies
Investment trends in hydrogen technologies reflect a growing recognition of its potential. With increased awareness of climate change, private and institutional investors are focusing more on sustainable energy solutions. A significant characteristic of this trend is the surge in partnerships between energy companies and research institutions. These collaborations can accelerate the development and commercialization of hydrogen technologies.
The unique feature of these investment trends is the diverse array of funding sources available today. Venture capital firms, multinational corporations, and even governmental bodies are now actively participating in hydrogen projects. However, it is essential to acknowledge that the investment landscape can be volatile, driven by competing interests and market fluctuations. This volatility may pose risks to long-term hydrogen initiatives.
"Understanding future trends in hydrogen conversion not only illuminates the path towards a sustainable energy future but also highlights the critical role of continuous innovation and strategic investment."
Through examining these aspects, we gain insight into the evolution of hydrogen technologies and their potential to transform the energy landscape in the years to come.