Industrial Hydrogen Production: Key Processes and Sustainability
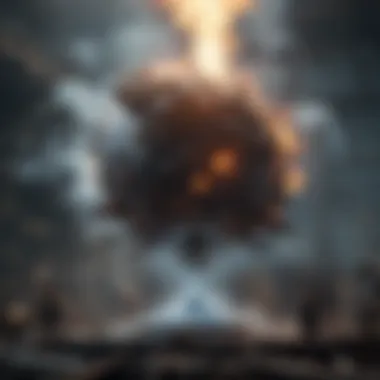
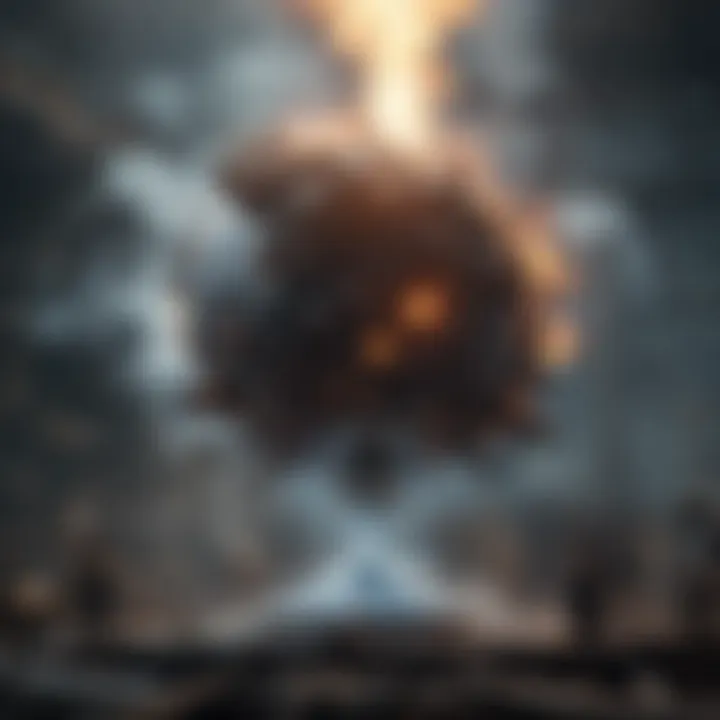
Intro
Industrial hydrogen production is far from a new concept; however, its importance has surged in recent years. As the world shifts towards more sustainable energy sources, hydrogen stands out as a versatile energy carrier. It finds applications not just in energy but also extends to various industries ranging from automotive to chemical synthesis. The ongoing evolution of hydrogen production processes is crucial in this landscape, addressing both the demand for cleaner energy solutions and the imperative of reducing greenhouse gas emissions.
This article aims to provide an in-depth exploration of the methods of hydrogen production, emphasizing the technological advancements and challenges accompanying each technique. We'll delve into processes like steam methane reforming, electrolysis, and biomass gasification, each carrying its unique attributes and implications for sustainability.
Research Highlights
Key Findings
- Diverse Hydrogen Production Methods: The article showcases multiple hydrogen production methods, illustrating the efficiency and carbon footprint of each. For instance, while steam methane reforming is prevalent, it is essential to consider the environmental costs associated with its carbon emissions. Conversely, electrolysis, often powered by renewable energy, presents a cleaner alternative.
- Emerging Technologies: New technologies are making headlines in the hydrogen sector. For example, advances in membrane technology for electrolysis are significant as they enhance efficiency and lower operational costs.
- Role of Hydrogen in Energy Transition: There's a growing recognition of hydrogen's role in global energy systems. Its capacity to act as a clean energy carrier is particularly vital in applications like fuel cells, which power vehicles and even backup energy systems.
Implications and Applications
The wide-reaching implications of hydrogen production extend into various sectors:
- Chemical Industry: Hydrogen is a key input for producing ammonia, a foundational chemical for fertilizers. Hence, its sustainable production can significantly lower the overall carbon footprint of agriculture.
- Transportation: Hydrogen fuel cells promise greener alternatives for vehicles, especially heavy-duty transport where battery technology may lag in performance.
- Energy Storage: Hydrogen holds potential as a method for storing excess renewable energy, particularly from wind and solar. This could help balance supply and demand in energy systems.
"The shift to hydrogen solutions is not merely a trend; it’s a fundamental change in how we approach energy production and consumption."
Methodology Overview
Research Design
The analysis in this article relies on a comprehensive literature review and current practices in the industry. By evaluating research papers, case studies, and technological reports, we compile a holistic view of the present landscape in hydrogen production and its future trajectory.
Experimental Procedures
The procedures followed for examining various methods include the following:
- Data Sourcing: Collecting qualitative and quantitative data from reliable sources, including academic papers and industry reports.
- Comparative Analysis: We compare the sustainability, efficiency, and applicability of different hydrogen production methods.
- Case Studies: Incorporating real-world examples helps to showcase how different technologies are applied in varied industrial contexts.
This foundational research lays the groundwork for a deeper understanding of the dynamic role hydrogen production plays in our interconnected world, addressing both current energy needs and futures that prioritize sustainability.
Prolusion to Industrial Hydrogen Production
Understanding industrial hydrogen production is vital in the landscape of modern energy. Hydrogen is not just a simple element; it stands as a pillar in the transition towards cleaner energy systems. As industries increasingly turn towards sustainable practices, emphasizing hydrogen's role becomes paramount.
Historical Context
The journey of hydrogen as a significant player in industrial processes dates back to the early 19th century, when scientists like Henry Cavendish first identified it. Its full potential, however, remained largely untapped until the mid-20th century when the demand for ammonia in fertilizers climbed. This paved the way for steam methane reforming, which has remained a dominant production method for decades. A shift gradually started occurring as the ecological consequences of fossil fuel reliance became clear in the latter half of the century. The need for a transition to cleaner alternatives was not just apparent but urgent, influencing technologies and policies.
Historically, hydrogen primarily derived from natural gas. Its accessibility and relative low cost fueled its wide-scale adoption in various sectors from petrochemicals to refining. The 21st century, marked by aggressive climate goals, sparked renewed interest in hydrogen production methodologies, focusing on lowering carbon emissions and enhancing sustainability. Cost-efficiency and resource availability shaped the development of these technologies, leading to more innovative practices like electrolysis and biomass gasification, providing not just alternatives but also complementary methods.
Current Relevance
Presently, hydrogen production bears more weight than ever due to its potential in addressing climate change and energy security. Countries are increasingly making commitments toward net-zero emissions, enabling hydrogen to emerge as a clean energy carrier. This importance can't be overstated; hydrogen is perceived as a solution to decarbonize various challenging sectors, including heavy industry and transportation.
Key players in the energy market are pivoting to Hydrogen not only for its versatile applicability but also as a means of enhancing energy storage. Energy systems integrating renewable sources like wind and solar face storage challenges, and hydrogen offers a bridge to secure these energy flows. Additionally, the global market for hydrogen is projected to grow, driven by both the sheer necessity of carbon neutrality and technological advancements.
The implications extend to government policies too. Incentives for green hydrogen production are shaping a new economic landscape, influencing market prices and the dynamics of hydrogen supply chains. Investments in infrastructure to support hydrogen economics are not mere trends; they are essentials for future energy security as well.
"The transition to a hydrogen economy is not just essential for energy diversification but also crucial in meeting global climate targets."
Overview of Hydrogen as a Commodity
Hydrogen holds a pivotal place in the current energy landscape, emerging not only as a fuel but as a critical commodity in various industrial applications. Its versatility ranges across numerous sectors, such as chemical production, energy storage, and transportation. With a growing emphasis on sustainability, understanding hydrogen's role as a commodity becomes increasingly relevant. This section enunciates the dynamics of hydrogen’s market, examining its significance and underpinning factors that influence its production and consumption.
Market Dynamics
The hydrogen market is a complex web of interactions driven by supply and demand, technological advancements, and geopolitical factors. Over recent years, this market has witnessed a notable shift towards greener production methodologies. Factors that render hydrogen essential include:
- Energy Transition: As countries pivot to lower carbon systems, hydrogen is viewed as a bridge among conventional and renewable energy sources. Its ability to store and transport energy positions it as an adaptable solution in integration with renewable technologies.
- Industrial Demand: Beyond its energy applications, hydrogen finds utility in vibrant sectors such as the chemical industry, where it acts as a fundamental feedstock for processes like ammonia synthesis and petroleum refining.
- Regulatory Frameworks: Politicians and regulatory bodies are increasingly implementing policies to encourage low-emission technologies. This shift creates additional demand for hydrogen from industries aiming to meet stringent environmental targets.
Given these dynamics, the hydrogen market is not static; it is likely to evolve rapidly, driven by both technological innovation and global initiatives aimed at decarbonization.
Price Fluctuations
Hydrogen pricing, much like other commodities, is subject to fluctuations influenced by several interconnected elements. Understanding these price dynamics can help stakeholders make informed decisions regarding production and investment in hydrogen technologies. Below are key points to consider:
- Production Methodology: The cost of hydrogen largely depends on how it is produced. For instance, hydrogen derived from steam methane reforming tends to be more economical than that produced via electrolysis, which employs electricity to split water. However, as renewable energy prices continue to decline, the cost of green hydrogen from electrolysis is becoming increasingly competitive.
- Raw Material Prices: The cost of input materials also plays a significant role. For example, natural gas prices directly impact hydrogen produced from fossil fuels. A spike in these prices can influence the overall cost of hydrogen generation.
- Market Speculation: Just like any other commodity markets, speculation can drive prices up or down based on perceptions about future supply and demand. External events, such as geopolitical tensions or natural disasters, can also sway market sentiments, leading to significant price volatility nearly overnight.
Above all, the interplay of these elements leads to a landscape where professionals involved in hydrogen production must navigate both opportunities and risks, balancing the pursuit of sustainability with economic feasibility.
Methods of Hydrogen Production
Hydrogen production methods are at the heart of the ongoing energy transition. Understanding these methods is crucial for assessing their practical applications and sustainability. Each technique has its unique set of benefits and challenges, which influence how industries adopt hydrogen as a fuel source. As the world shifts towards cleaner energy solutions, the methods employed to produce hydrogen will play an essential role in shaping future energy landscapes.
Steam Methane Reforming (SMR)
Process Overview
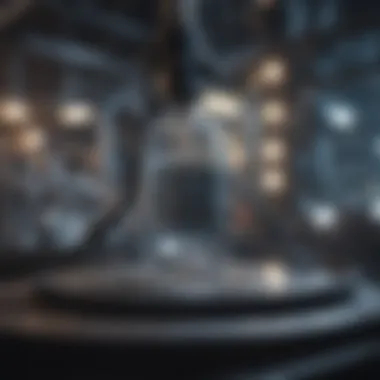
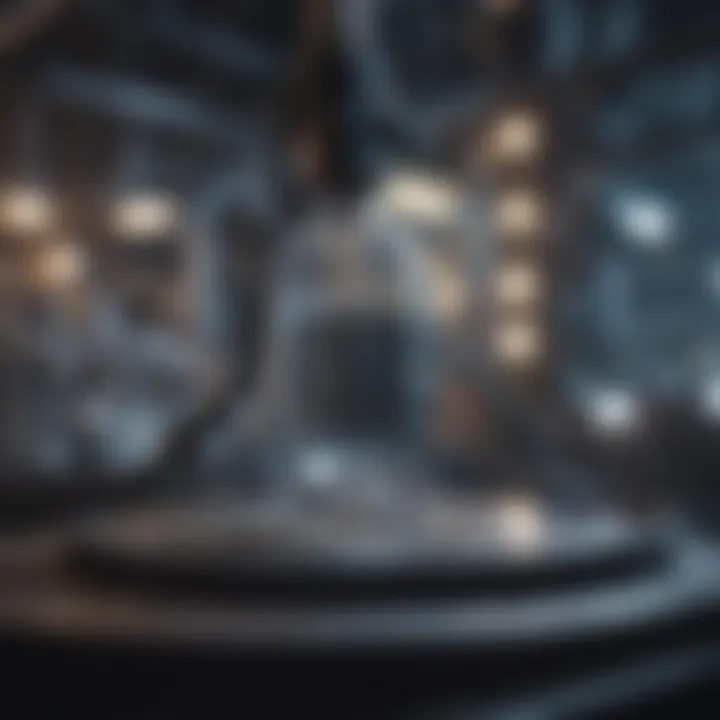
The Steam Methane Reforming process is the most widely used method for producing hydrogen. SMR involves reacting methane with steam under high temperature and pressure, producing hydrogen, carbon monoxide, and some carbon dioxide. The key characteristic of this process is its efficiency in converting hydrocarbons into hydrogen, making it a dependent choice for many hydrogen producers.
One unique aspect of SMR is its adaptability to existing natural gas infrastructures. As a result, it's seen as a practical and cost-effective method. However, the process emits significant amounts of carbon dioxide, raising concerns about environmental sustainability.
Advantages and Disadvantages
The advantages of SMR are rooted in its commercial viability and widespread application. It utilizes readily available natural gas as feedstock and can produce large volumes of hydrogen at relatively low costs.
On the flip side, the environmental costs cannot be ignored. The process contributes to greenhouse gas emissions, which is a significant drawback in the current climate-conscious landscape. Moreover, as regulations tighten around carbon emissions, the reliance on SMR may expose industries to future liabilities.
Future Perspectives
In terms of future perspectives, SMR is facing pressure to adapt to rising sustainability demands. Innovations, such as integrating carbon capture and storage technologies, may help mitigate some of the environmental impacts. However, this will require significant investments and may involve additional complexities in operations.
The shift towards greener hydrogen production methods is likely to lead to increased research and development in this area, presenting both challenges and opportunities for existing SMR facilities.
Electrolysis of Water
Types of Electrolysis
Electrolysis involves splitting water into hydrogen and oxygen using an electric current. There are several types of electrolysis, including alkaline electrolysis, proton exchange membrane (PEM) electrolysis, and solid oxide electrolysis. Each type has its own efficacy and application scenarios.
For instance, PEM electrolysis offers advantageous characteristics such as high purity hydrogen production and suitability for variable loads, making it an attractive option when paired with renewable energy sources.
Efficiency and Cost Factors
Efficiency and cost are critical considerations for electrolysis. The energy efficiency of the process can vary significantly based on the technology used and the purity of the water. Generally, circumstances like high electricity costs or limited access to renewable energy can hinder the economic attractiveness of electrolysis.
Technology improvements and scale-up will be vital in addressing these efficiency concerns while also driving down operational costs.
Integration with Renewable Energy
Integration with renewable energy sources is one of the standout features of the electrolysis process. It can act as a buffer for surplus energy, converting excess production into hydrogen for future use. This not only aids in energy storage but amplifies the feasibility of a decarbonized energy system.
However, the reliance on stable, affordable renewable energy sources is essential to make this integration practical and cost-effective.
Biomass Gasification
Process Description
Biomass gasification converts organic materials into hydrogen through thermal decomposition in a low-oxygen environment. The process aims to produce syngas, from which hydrogen can be separated. Its feedstock versatility makes it a notable contender among hydrogen production techniques.
By utilizing various organic materials, from agricultural waste to dedicated energy crops, this method displays considerable promise for sustainable practices—drawing attention in a world seeking to reduce waste.
Feedstocks and Sustainability
The sustainability aspect of biomass gasification revolves around its potential to utilize waste products effectively. Using biomass not only curtails the environmental footprint but also enables a circular economy where waste is repurposed.
However, challenges around sourcing and consistent quality of feedstocks linger. Sustainability success relies on ensuring that feedstock acquisition is environmentally sound and does not compete with food production.
Challenges in Implementation
Implementing biomass gasification poses its own hurdles. Technical challenges include managing the variability in feedstock and maintaining efficient operations. Regulatory considerations further complicate the landscape, as differentiated standards exist by region regarding biomass utilization.
Natural Gas Reforming
Conversion Technologies
Natural Gas Reforming is the overarching category that includes methods like SMR and autothermal reforming (ATR). Conversion technologies play a central role in how hydrogen can be efficiently extracted from natural gas.
The distinct advantage of these technologies is the scalability and existing infrastructure, which makes them appealing in the short run. However, as the environmental stakes rise, reliance on these methods may become more complicated.
Impact on Global Warming
The impact of natural gas reforming practices on global warming is critical to assess. Efficient operations can still lead to substantial carbon dioxide emissions. The move towards cleaner methods is essential as energy policies shift toward climate action, making it pressingly important to consider lower-emission alternatives.
Regulatory Considerations
Regulatory considerations are becoming especially necessary as environmental standards tighten globally. Expect pressure on industries involved in natural gas reforming as stakeholders—including consumers—demand more transparency and accountability. Regulatory frameworks are evolving, and responsiveness will be key for companies operating in this arena.
Thermochemical Water Splitting
Process Mechanism
Thermochemical water splitting employs chemical reactions driven by heat to produce hydrogen. Though still largely experimental, the mechanism holds promise for efficient hydrogen production without the need for electrolysis.
The unique selling point here is potentially lower energy requirements, which may become a significant advantage in the future as technological advances are made.
Efficiency Metrics
Efficiency metrics for thermochemical processes are still indicative of potential rather than established practices. Understanding these metrics requires context, as many technologies in their infancy often have variable outputs. Continued experimentation will be required to refine this technology for broader application.
Commercialization Outlook
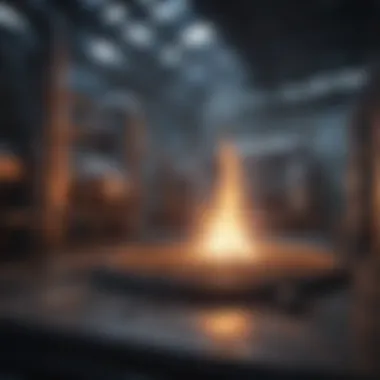
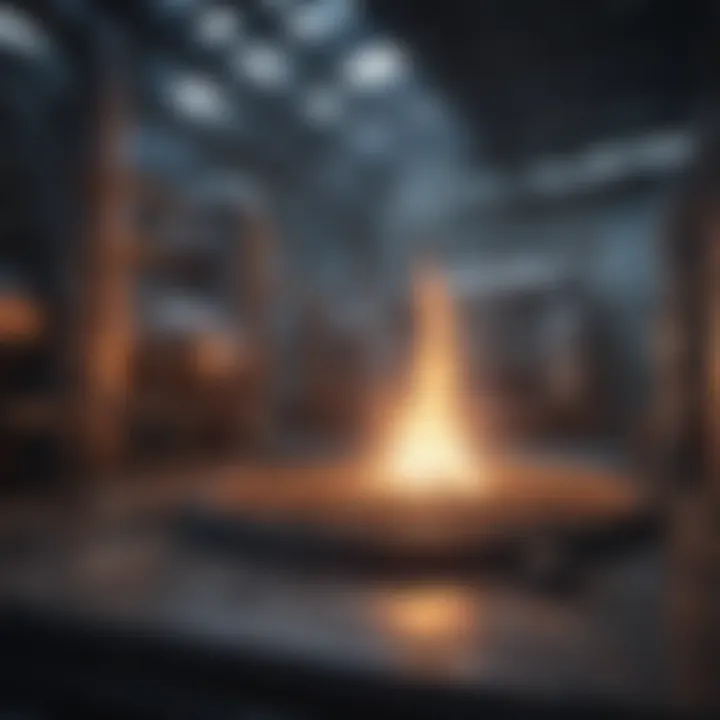
Commercialization outlook depends heavily on success in lab settings transitioning to practical applications. Collaborative efforts in research and development will be paramount in understanding whether thermochemical splitting becomes a viable alternative for large-scale hydrogen production.
Keeping a pulse on innovations in hydrogen production methods will be crucial. As new technologies emerge, the energy landscape may shift dramatically, making it essential to remain adaptive and informed.
Applications of Hydrogen in Industry
The significance of hydrogen in various industrial applications cannot be overstated. It plays a vital role in numerous sectors, most notably in chemical production, energy storage, and transportation. As industries seek greener, more sustainable solutions, hydrogen emerges as a leading candidate for aiding the transition towards cleaner energy systems. This section delves into key applications of hydrogen, emphasizing its benefits and considerations for future development.
Hydrogen in the Chemical Industry
Ammonia Production
Ammonia production is one of the most prominent uses of industrial hydrogen. The Haber-Bosch process, which synthesizes ammonia from atmospheric nitrogen and hydrogen, is fundamental to modern agriculture. This reaction delivers an essential fertilizer that significantly boosts food production globally.
One key characteristic of ammonia production is its efficiency; it allows for large-scale synthesis that meets the ever-increasing demand for fertilizers. Moreover, this process benefits from established infrastructure, making it a robust option for industries. However, the environmental impact of ammonia production remains a concern due to carbon emissions produced during hydrogen generation from fossil fuels. The shift toward using renewable hydrogen for ammonia synthesis is a unique feature that holds the promise of making this process more sustainable. Understanding these nuances is crucial in evaluating ammonia production's advantages against its disadvantages in the greater context of hydrogen applications.
Hydrogenation Reactions
Hydrogenation reactions utilize hydrogen to convert unsaturated hydrocarbons into saturated compounds. This process is essential in the food industry for producing margarine and other oils. The ability to alter fat composition highlights the functionality of hydrogen in facilitating essential chemical reactions.
A key advantage of hydrogenation is its ability to improve product stability and shelf life, making it a favored choice in food products. Moreover, these reactions can be tailored according to specific needs, providing versatility. While the unique feature of this application lies in modifying physical properties, there are considerations regarding trans fats and health implications, which can affect consumer choice and industry practices.
Synthetic Fuels
Synthetic fuels produced from hydrogen are another critical application. Hydrogen can combine with carbon sources to create hydrocarbons, offering a cleaner alternative to conventional fossil fuels. The advantage of synthetic fuels is their compatibility with existing fuel infrastructure, allowing a smoother transition toward using hydrogen.
A standout feature of synthetic fuels is that they can be produced sustainably, especially if renewable resources are utilized. However, the cost associated with the production processes and the need for significant technological advancements present challenges. Thus, while promising, synthetic fuels must continuously evolve to address economic and environmental pressures.
Role of Hydrogen in Energy Storage
Hydrogen as a Fuel Cell
Fuel cells are at the forefront of clean energy storage and conversion technologies. By utilizing hydrogen, these electrochemical devices convert fuel into electricity with water as the only byproduct. This clean mechanism is a major advantage, especially in the face of worsening air quality and climate change.
One major characteristic of hydrogen fuel cells is their scalability, making them suitable for everything from small devices to large power systems. In the context of energy storage, they can store excess renewable energy, thereby enhancing grid stability. The unique feature of hydrogen as a fuel cell lies in its high energy density compared to traditional batteries, providing longer runtimes. However, challenges such as infrastructure development and hydrogen distribution deficiencies affect practical implementation.
Pumped Energy Systems
Pumped energy systems represent another innovative method of storing hydrogen energy. Essentially, they pump water uphill to a reservoir when excess energy is available. When energy demand peaks, water is released to generate electricity.
The standout attribute of pumped energy systems is their efficiency in energy management. By integrating hydrogen production into this framework, industries can effectively balance supply and demand. However, the limitations include geographical constraints and the significant initial investment required to establish such systems.
Thermal Energy Storage
Thermal energy storage using hydrogen involves storing heat generated through hydrogen combustion. This approach can stabilize energy supply, particularly in renewable energy systems by harnessing off-peak energy for later use.
A key feature of thermal energy storage is its potential to utilize existing technology, easing the transition for many industries. This method can balance energy loads efficiently, but it also faces challenges related to system integration and heat losses over time.
Transport Sector Applications
Fuel Cell Vehicles
Fuel cell vehicles (FCVs) represent one of the most promising applications of hydrogen in the transport sector. These vehicles rely on hydrogen fuel cells to generate power, offering an alternative to conventional internal combustion engines. The clean emissions of FCVs, mostly water vapor, present a compelling case for their adoption in reducing urban pollution.
The primary characteristic of FCVs is their reduced environmental footprint when compared to gasoline or diesel vehicles. However, the challenges in terms of hydrogen refueling infrastructure must be addressed for widespread adoption.
Hydrogen-Powered Trains
Hydrogen-powered trains are gaining traction as a sustainable alternative to diesel trains. These trains utilize hydrogen fuel cells for propulsion, striking a balance between operational efficiency and environmental consciousness. The key aspect of hydrogen-powered trains is their ability to operate without producing direct emissions, addressing critical transportation emissions issues in railways.
Unique features include the potential for retrofitting existing diesel trains to hydrogen, offering compatibility with current infrastructure. Nevertheless, this approach must contend with the availability of hydrogen and the associated costs, which can deter quick implementation.
Shipping Innovations
The shipping industry is exploring hydrogen as a means to reduce its carbon footprint significantly. Innovations such as hydrogen-powered vessels are in the development phase, aimed at addressing the heavy emissions associated with maritime transport.
A standout quality of shipping innovations lies in their potential to significantly cut greenhouse gas emissions while maintaining shipping efficiency. The use of hydrogen in shipping holds unique prospects for cleaner oceans and environments. Yet, achieving this transition faces notable obstacles, mainly concerning infrastructure and safety regulations.
Overall, hydrogen's versatility across various industrial applications showcases its potential as a key player in the future of energy. Its contributions to the chemical industry, energy storage, and transportation are significant, but addressing associated challenges is vital for unlocking its full potential.
Challenges Facing Hydrogen Production
The realm of industrial hydrogen production holds the promise of a cleaner, more sustainable future. However, it is not without its share of hurdles. As we explore the numerous advantages hydrogen offers, we must also tackle the critical challenges that could hinder its widespread adoption. Addressing these obstacles is not merely an academic exercise; it’s a pressing priority for industries, policymakers, and researchers alike. Understanding the economic viability, technological barriers, and infrastructural limitations can guide the development of more effective strategies to harness hydrogen's potential as a clean energy source.
Economic Viability
When we talk about economic viability, it’s important to grasp the subtleties involved in the production of hydrogen. The cost of production varies significantly depending on the method employed. For instance, steam methane reforming often appears more affordable initially due to established infrastructure; however, its long-term environmental costs can be staggering. In comparison, electrolysis, while greener, requires high-energy input, which in turn drives up costs.
- Key factors influencing economic viability include:
- Production Costs: These vary by methods—how much energy goes in versus how much hydrogen comes out?
- Market Demand: A rise in demand for hydrogen, especially green hydrogen, can lead to economies of scale, lowering costs.
- Government Policies: Subsidies and incentives greatly affect economic dynamics.
This economic dance is multifaceted, often requiring industry-wide collaboration to ensure hydrogen remains competitive against other energy sources.
Technological Barriers
As technology continues to burgeon, the hydrogen sector frequently encounters persistent technological barriers. Despite the advances made in hydrogen production, the efficiencies achieved aren't necessarily enough to tip the scales in its favor. Complications arise in different areas:
- Energy Efficiency: For instance, in electrolysis, the conversion efficiency from electricity to hydrogen remains an uphill battle, with efficiency rates often hovering around 70-80%.
- Durability of Equipment: The robustness of electrolyzers can be a sticking point; their operational lifespan often comes under scrutiny, particularly in harsh environments.
Overcoming these technological hurdles is not an insurmountable task, but it demands innovative approaches, extensive research, and perhaps a bit of trial and error.
Infrastructure Limitations
Infrastructure issues represent a significant challenge in hydrogen production and distribution. While the groundwork for traditional energy systems is well-established, hydrogen infrastructure is still developing. Unless we put in some serious effort, it’s a case of catching up—fast.
The following aspects are crucial when considering infrastructure limitations:
- Transport and Storage: Hydrogen's low density makes it tricky to store and transport. For instance, it may require liquefaction or high-pressure gas systems that are costly.
- Distribution Networks: Current natural gas pipelines can’t simply be repurposed for hydrogen without significant modifications, raising questions about how to effectively distribute the produced hydrogen.
“Creating a seamless hydrogen economy is as much about innovation in infrastructure as it is about advancements in production technology.”
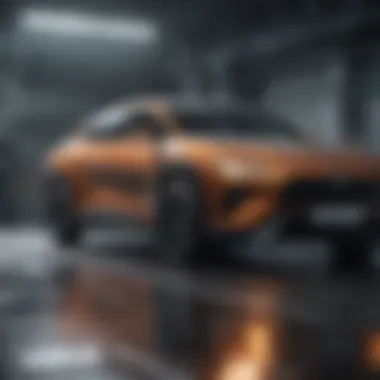
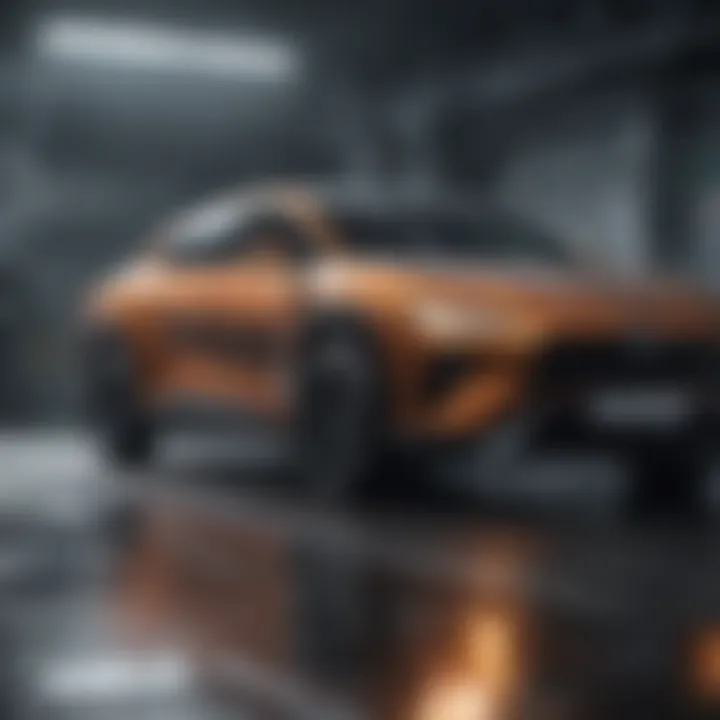
Each of these elements—economic viability, technological barriers, and infrastructure limitations—interplays like notes in a symphony. A harmonious balance could lead to the successful integration of hydrogen into our future energy systems. Concluding our review of challenges, it’s evident that while the road ahead is fraught with complexities, tackling these challenges head-on is essential for capturing the full potential of hydrogen as a sustainable energy source.
Environmental Considerations
In the quest for cleaner energy sources, environmental considerations surrounding industrial hydrogen production take center stage. The impact of hydrogen processes on air quality, water resources, and the overall ecosystem cannot be overlooked. As industries pivot from fossil fuels to alternatives, understanding these considerations is paramount for sustainable growth and regulatory compliance.
Carbon Emissions Impact
Firstly, the carbon emissions generated through various hydrogen production methods are a pivotal concern. Traditional production techniques, particularly steam methane reforming, release significant amounts of carbon dioxide into the atmosphere. For instance, reports suggest that SMR can emit around 9 to 12 tons of CO2 per ton of hydrogen produced. This stark reality drives home the need to develop cleaner alternatives that significantly reduce greenhouse gas emissions.
The shift towards sustainable production is critical. Innovations in methods like electrolysis—particularly when powered by renewable energy—present a clear path to minimizing the carbon footprint. However, it is essential to consider the entire energy lifecycle and the sources of electricity used in these processes.
"The choice of hydrogen production method can dictate future environmental outcomes. A careful analysis is needed to gauge the best suited approach for mitigating carbon emissions."
Sustainable Hydrogen Production
Sustainable hydrogen production embodies a proactive approach to addressing environmental challenges. Key aspects include focusing on renewable sources while minimizing ecological disruption during the production process. Implementing methods that both generate hydrogen and adhere to sustainability protocols can revolutionize industry practices.
Green Hydrogen Opportunities
Green hydrogen is produced through the electrolysis of water using renewable energy sources like wind and solar. Its standout feature is that it virtually emits no carbon during production, making it a mirror reflecting sustainability. This character trait of green hydrogen positions it as an attractive option for various applications, especially in industries looking to enhance their environmental credentials.
Advantages of green hydrogen include:
- Zero Carbon Emissions: The process does not produce any harmful pollutants.
- Renewable Integration: It can be produced in tandem with renewable energy, allowing for a decentralized energy system.
However, the disadvantages shouldn’t be ignored. The efficiency and current costs associated with electrolysis still pose challenges for widespread adoption. Greater investment in technology advancements is necessary to boost economic viability.
Life Cycle Assessment
Another crucial element is the Life Cycle Assessment (LCA) of hydrogen production methods. This assessment provides an in-depth analysis of environmental impacts from production through to consumption. The key characteristic of LCA is that it takes into account all phases of a product's life, enabling more precise sustainability evaluations.
LCA is advantageous because:
- Holistic Viewpoint: It considers upstream and downstream impacts, identifying hotspots for emissions and resource use.
- Guidance for Policymakers: Policymakers can make informed decisions on subsidies and regulations based on comprehensive data.
Conversely, LCA can be complex and resource-intensive, requiring significant expertise and data which may not always be readily available. The inaccuracies in data sourcing can also lead to conflicting conclusions, hence the quality of the LCA can greatly affect its findings.
In summary, refining environmental considerations in industrial hydrogen production helps lay the groundwork for a sustainable future. Incremental improvements and commitment to innovation will redefine the industrial landscape, aligning energy production with ecological and social responsibility.
Future of Industrial Hydrogen Production
The future of industrial hydrogen production holds significant promise, intertwining advancements in technology, evolving policies, and shaping market dynamics. Understanding this future is crucial, as it directly impacts not only the energy industry but also economic growth, environmental sustainability, and innovations across various sectors. Key elements to consider in this discussion include the drive for cleaner energy sources, the challenges posed by existing infrastructures, and the potential for hydrogen to become a cornerstone of the emerging low-carbon economy.
Innovations on the Horizon
Innovations in hydrogen production are sprouting from every corner of research and development. The landscape is continuously shifting toward more efficient and sustainable methods. Some notable advancements include:
- Improved Electrolysis Techniques: New methods, such as proton exchange membrane (PEM) electrolysis, are enhancing efficiency and reducing costs. The integration with renewable sources like solar or wind further optimizes production.
- Research in Biomass Conversion: There’s a growing interest in converting agricultural waste into hydrogen through thermochemical processes. This approach not only produces clean energy but also addresses waste management issues.
- Advancements in Thermochemical Water Splitting: This method utilizes concentrated solar power to drive chemical reactions that split water into hydrogen and oxygen. With improvements in solar technologies, this process might capture a larger share of hydrogen production in the future.
These innovations underscore a movement towards hydrogen production methods that are not just economically viable but also environmentally benign.
Policy and Regulatory Impacts
Government policies play a vital role in shaping the future of hydrogen production. As nations grapple with climate change objectives, regulations are being adapted to promote hydrogen as a clean alternative.
Considerations include:
- Incentives for Clean Technology Adoption: Many countries are already offering tax incentives or grants for businesses that invest in hydrogen production technologies. Such support can effectively lower the start-up costs associated with innovative hydrogen projects.
- Standardization of Hydrogen Infrastructure: Clear regulatory frameworks are necessary to establish safety standards and protocols for hydrogen transport and storage. With potential risks of hydrogen leaks, building trust and safety in storage systems is crucial.
- International Collaborations: Countries are beginning to engage in shared hydrogen projects promoting international best practices and consistent regulations. Collaborations like these can catalyze new markets and lead to greater hydrogen adaptability.
These regulatory developments ensure that hydrogen production does not occur in a vacuum but rather alongside global efforts for sustainability and economic growth.
Global Market Trends
A close look at market trends reveals the shifting landscape of hydrogen within global economies. Factors impacting these trends include:
- Rising Demand for Clean Energy: As industries and governments aim to achieve net-zero emissions, hydrogen is seen increasingly as a clean alternative for sectors hard to decarbonize.
- Investments in Green Hydrogen Projects: Financial flows into green hydrogen projects are increasing, influencing how energy markets are evolving. Companies are recognizing the critical role that hydrogen will play in future energy systems, leading to strategic investments.
- Integration with Other Markets: As hydrogen technology matures, its synergies with other sectors—like transportation and power generation—are becoming more evident. For instance, hydrogen fuel cells are gaining traction in vehicle markets, while hydrogen storage is seen as essential for stabilizing renewable energy sources.
As the market adapts to the realities of climate change, the holistic role of hydrogen production as a central player in both economic recovery and eco-friendliness cannot be understated.
In summary, the future of industrial hydrogen production is a tapestry woven with potential innovations, supportive policies, and evolving market dynamics. Understanding these elements is fundamental for stakeholders looking to navigate the clean energy transition.
End
Hydrogen production has emerged as a pivotal facet in the landscape of modern energy solutions. As the world grapples with the pressing challenge of climate change, the role of hydrogen becomes ever more significant. This conclusion ties together the threads woven throughout the article, highlighting the necessity for a cohesive understanding of hydrogen's production methods, applications, and sustainability initiatives.
Summary of Key Insights
Through the various sections explored in this article, several key insights have surfaced:
- Diverse Production Methods: Methods such as steam methane reforming, electrolysis, and biomass gasification each offer unique benefits and drawbacks. Understanding these processes is crucial for stakeholders aiming for economic viability and sustainability.
- Applications Across Industries: Hydrogen isn’t just a fuel or a raw material; it is utilized across numerous sectors including energy storage, transportation, and chemical manufacturing. This versatility underscores its potential as a clean energy vector in our transition to greener solutions.
- Sustainability Considerations: The future of hydrogen production must be aligned with sustainable practices. The development of green hydrogen, produced through renewable sources, offers a pathway toward minimizing carbon footprints in various industries.
As interest in industrial hydrogen surges, it is essential to stay informed about technological advancements and policy frameworks that could shape the industry. A comprehensive understanding not only benefits industry players but also informs public discourse on energy transition.
Final Thoughts on Future Directions
Looking ahead, several trends and considerations will shape the future of hydrogen production:
- Innovation in Technologies: Research and development will be vital in enhancing the efficiency of production processes. Breakthroughs in electrolysis and thermochemical cycles may pave the way for lower costs and higher output.
- Regulatory Frameworks: As different nations prioritize clean energy, supportive policy measures can significantly influence the pace of hydrogen adoption. Collaborations and standardizations will likely play a role in fostering an integrated hydrogen economy.
- Global Supply Chains: Developing infrastructure for hydrogen distribution is essential. Considerations of safety, transportation logistics, and storage methods will dictate how effectively hydrogen can be utilized in various locations.