Exploring Innovations in Power Electronics
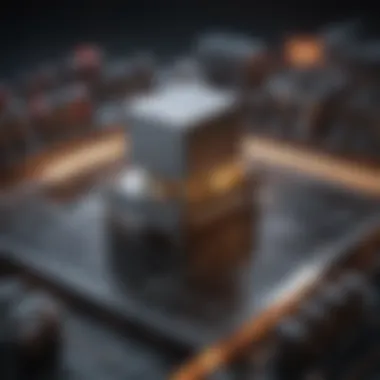
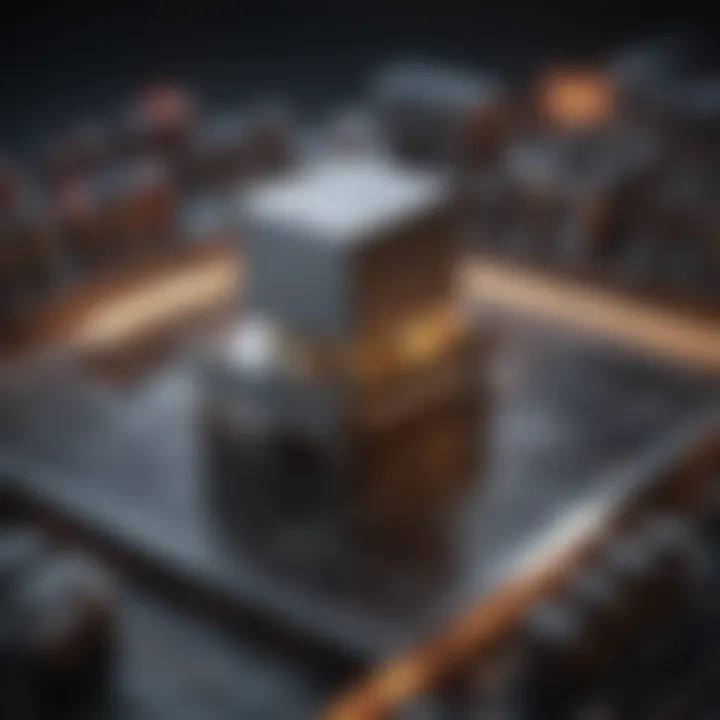
Intro
Power electronics represents a critical framework in contemporary technology, enacting significant changes across diverse sectors. By controlling and converting electrical power efficiently, power electronics underpins advancements in renewable energy, electric vehicles, and smart grids. As the world emphasizes energy efficiency and sustainability, power electronics becomes central to these discussions. This article aims to dissect the fundamental ideas, components, and ramifications of power electronics, providing an extensive analysis on how these innovations shape our technological landscape.
Research Highlights
Key Findings
In the study of power electronics, several vital findings emerge:
- Efficiency Improvements: Modern power semiconductor devices have shown remarkable efficiency. Gallium nitride (GaN) and silicon carbide (SiC) enable superior performance compared to traditional silicon-based devices.
- Control Strategies: Advanced control techniques such as model predictive control (MPC) and adaptive control are enhancing the precision and reliability of power converter applications.
- Integration with Renewable Sources: Power electronics play a pivotal role in integrating wind and solar energy sources into power grids, facilitating on-demand electricity generation.
Power electronics not only aids in energy conversion but also assists in global efforts towards decarbonization by optimizing the performance of renewable energy sources.
Implications and Applications
The implications of these findings are broad, impacting sectors ranging from industrial automation to consumer electronics. Key applications include:
- Electric Vehicles: Power electronics enables the smooth operation of electric drivetrains, enhancing performance and energy management.
- Renewable Energy Systems: Efficient power converters are essential for harnessing solar and wind energy, ensuring that this energy is usable and stable.
- Data Centers: Data centers utilize power management techniques to improve energy consumption, impacting overall operational efficiency.
Methodology Overview
Research Design
To examine the landscape of power electronics, this article employs a mixed-methods approach combining theoretical analysis with case studies and anecdotal evidence from various industries. This design allows for a comprehensive understanding of both the theoretical foundations and practical implications.
Experimental Procedures
Experiments highlighted in this article include real-world applications of power electronics systems in various scenarios. Analysis involves:
- Simulation Studies: Leveraging software tools to model power electronic circuits and predict performance outcomes under different conditions.
- Field Tests: Engaging with industry partners to evaluate the performance of newly developed power electronic devices in situ.
- Literature Review: Gathering insights from existing research and case studies to understand trends and challenges faced in the field.
This methodology provides a well-rounded view of current practices and innovations in power electronics, setting the stage for further exploration in subsequent sections.
Prologue to Power Electronics
The field of power electronics plays a crucial role in various technological advancements today. It enhances the efficiency of energy systems, integrating innovations that support the growing demand for sustainable solutions. This section provides an overview of power electronics, laying the groundwork for a detailed exploration of its innovations and applications.
Power electronics focuses on converting electrical power from one form to another, managing energy in ways crucial for both consumer and industrial sectors. The innovations in this area have directly influenced improvements in energy efficiency, reliability, and performance of electrical systems. It is important to understand how these components and processes function and how they impact modern technology and daily life.
Definition of Power Electronics
Power electronics is the study and application of electronic circuits to control and convert electrical power. This field integrates various disciplines, including electrical engineering, material science, and control systems. Its primary objective involves the management of power flows within electronic devices, transforming electrical energy to fit the demands of the specific applications or systems.
Notably, power electronics incorporates several key processes, such as rectification, inverter operation, and power factor correction. As a result, its applications extend from consumer electronics to large industrial machines, illustrating its extensive relevance. In addition to traditional uses, recent innovations have led to implementations in renewable energy systems, electric vehicles, and smart grid technologies.
Historical Development
The origins of power electronics can be traced back to discoveries in semiconductor materials and the development of electronic devices in the mid-20th century. The evolution began with the introduction of silicon as a semiconductor material, enabling the creation of diodes and transistors. These early developments provided the foundation for the modern power electronics we rely on today.
Over the years, advancements in materials and manufacturing processes have led to the production of more efficient power conversion devices. The invention of the thyristor in the 1950s marked a significant milestone, as it allowed for control of high power levels. The 1970s and 1980s saw further innovations, including the advent of insulated gate bipolar transistors (IGBT), which facilitated greater efficiency and performance in industrial applications.
As technology progressed, the focus on energy efficiency began to rise. This led to the integration of power electronics in renewable energy sources, such as wind and solar energy systems. The evolution continues today, reflecting the increasing importance of sustainability and smart technologies.
Core Components of Power Electronics
In the realm of power electronics, the core components play a pivotal role in determining the performance, efficiency, and reliability of power systems. These components contribute decisively to the overall functionality, making their understanding essential for anyone interested in the field. Each component type fulfills specific functions and brings unique benefits and considerations to various applications.
Power Semiconductor Devices
Power semiconductor devices form the backbone of modern power electronics. They are vital for controlling and converting electric power, catering to growing demands for efficiency and reliability across numerous applications.
Diodes
Diodes are fundamental components in power electronic circuits. They allow current to flow in one direction while blocking it in the opposite direction. A key characteristic of diodes is their ability to handle high voltage and current levels, which makes them indispensable for applications such as rectification.
The most common type of diode used in power electronics is the silicon diode. These are popular due to their simplicity and robustness. A unique feature of silicon diodes is their low forward voltage drop, which enhances efficiency by reducing energy loss during operation.
However, the main disadvantage of diodes lies in their inability to switch off quickly. This characteristic limits their applications in high-frequency circuits where rapid switching is required.
Transistors
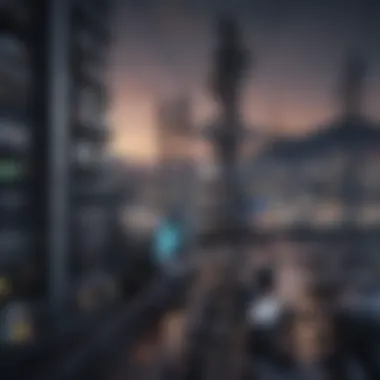
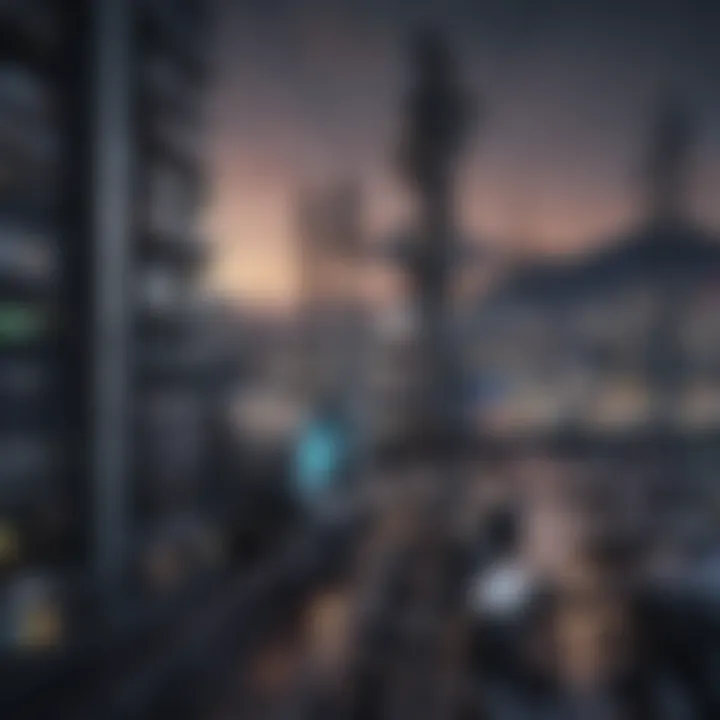
Transistors are essential for signal amplification and switching in power electronic systems. They can act as a switch or amplifier, making them incredibly versatile. A notable feature of transistors is their ability to control large amounts of current with a small input signal, which is essential for modern electronic devices.
Field Effect Transistors (FETs) and Bipolar Junction Transistors (BJTs) are commonly used in power applications. FETs, particularly, are advantageous due to their high efficiency and fast switching capabilities. However, they can be more costly compared to other options.
Despite their benefits, transistors have limitations such as power dissipation, which can affect thermal management in systems.
Thyristors
Thyristors are specialized semiconductor devices used in high-power applications. They are unique in their ability to control large amounts of current with a relatively small control signal. A significant characteristic of thyristors is their ability to handle high voltage levels while maintaining efficiency.
One unique feature of thyristors is their latching capability. Once triggered, they remain on until the current through them drops below a certain threshold, allowing for simple control in power electronics circuits. This feature is especially useful in applications like motor control and power regulators.
However, thyristors have disadvantages as well. Their inability to turn off quickly can be a limitation in high-speed switching applications.
Passive Components
Passive components play a supporting role in power electronics systems. They do not amplify or control power but are crucial for energy storage, filtering, and energy management. Understanding the contributions of these components is vital for effective circuit design.
Inductors
Inductors store energy in a magnetic field created by the electric current flowing through them. They are essential in applications such as energy storage and filtering. The key characteristic of inductors is their ability to resist changes in current. This makes them valuable in managing power fluctuations and ensuring stable operation in circuits.
One unique feature of inductors is their time delay characteristics, which can be advantageous for smoothing out voltage levels. However, they can be bulky and heavy, which can impact the design of portable devices.
Capacitors
Capacitors store electrical energy in an electric field. They are widely used in power electronics for energy storage, filtering, and voltage stabilization. A notable characteristic of capacitors is their ability to release energy quickly, which is beneficial for applications requiring rapid bursts of power.
Ceramic and electrolytic capacitors are common types found in power electronics. While ceramic capacitors are known for their reliability, electrolytic capacitors can store more energy but may have shorter lifespans.
The unique feature of capacitors is their frequency response, allowing them to filter out unwanted signals in power supply circuits. Nonetheless, they can be sensitive to voltage levels and might pose reliability issues, particularly in high-stress environments.
Resistors
Resistors control current flow in electric circuits by providing a specific amount of resistance. Their role is essential in managing power levels and ensuring circuit integrity. Resistors are key for dividing voltages and limiting current, which can protect sensitive components from overheating or damage.
One of the main characteristics of resistors is their simple structure and wide availability. Thin film and wire-wound resistors are popular choices in power electronics. Thin film resistors offer precision, while wire-wound types handle higher power levels better.
However, a significant disadvantage is their tendency to generate heat, which must be managed carefully to prevent component failure.
Control Circuits
Control circuits are integral to the functioning of power electronics. They manage the operation of the power electronic components, leading to optimized performance and enhanced efficiency. Their design and implementation are critical as they influence how power is converted, regulated, and delivered across various applications.
Fundamental Operating Principles
The operating principles of power electronics form the foundation for understanding how various components interact to manage electrical energy. This section is crucial as it lays the groundwork for grasping how power conversions and switching techniques operate. Appreciating these principles is essential for advancing technologies in renewable energy, electric vehicles, and industrial automation.
Conversion Processes
AC to Conversion
AC to DC conversion, also known as rectification, is vital in power electronics. It allows alternating current (AC) from mains or other sources to be converted into direct current (DC) which many devices require. The most common characteristic of AC to DC conversion is the use of diodes in rectifier circuits. This conversion is beneficial since most electronic devices operate on DC, including laptops and smartphones.
A unique feature of AC to DC conversion is its ability to stabilize voltage levels. This results in decreased risk of voltage spikes damaging sensitive electronic devices. However, it is not without disadvantages; the process can lead to inefficiencies if not properly designed. Heat generation is often a concern which may necessitate additional thermal management solutions.
to AC Conversion
DC to AC conversion, known as inversion, is another crucial process in power electronics, particularly in renewable energy systems. This conversion allows for the energy generated by solar panels or battery systems to be utilized in homes or fed back into the grid, which operates on AC. The converter’s efficiency is a key aspect, and the technology employed often includes transistors and specialized control circuits.
DC to AC conversion is popular because it enables integration with existing AC infrastructure, making energy distribution easier. A distinctive feature of this process is the ability to adjust output frequency, which is important for applications like motor drives. Despite its advantages, DC to AC conversion can sometimes introduce harmonics that may affect overall power quality.
to Conversion
DC to DC conversion is essential in applications where voltage levels need to be changed without converting to AC. It is frequently used in power supplies for electronics. A fundamental aspect of conversion is the step-up and step-down capabilities through devices such as buck and boost converters.
This type of conversion is beneficial as it improves the range of voltage levels and optimizes energy usage in various applications. Among its unique features is the high efficiency it can achieve, often above 90% in well-designed systems. On the downside, depending on the design and components used, it can introduce complexities in circuit designs.
Switching Techniques
Switching techniques are central to the operation of power electronics. They dictate how power semiconductor devices manage the flow of electrical energy. Efficient switching can enhance system reliability and performance, crucial factors in applications like industrial automation and electric vehicles.
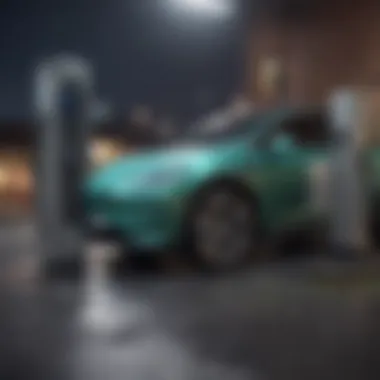
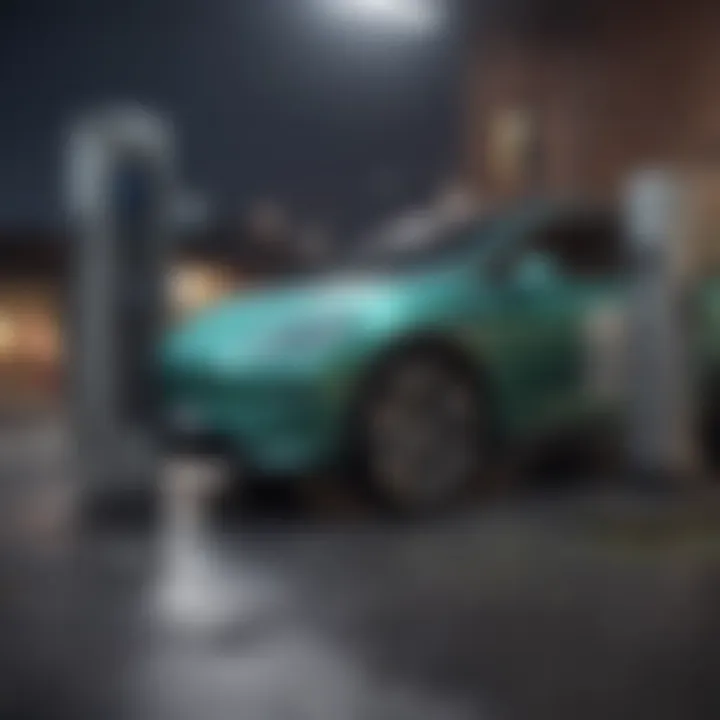
Common switching techniques include pulse width modulation (PWM) and linear control, each with its advantages and considerations. For instance, PWM provides better efficiency and lower heat generation compared to linear control. Understanding these techniques is essential for optimizing energy conversion systems and ensuring they meet the demands of modern technology.
The mastery of fundamental operating principles in power electronics influences efficiency, reliability, and innovation across various industries.
Applications of Power Electronics
Power electronics plays a crucial role in modern technology, influencing various sectors like renewable energy, electric vehicles, and industrial automation. This section emphasizes its significance in enhancing energy efficiency and sustainability. By understanding diverse applications, stakeholders can strategically implement power electronic systems for better performance and reliability.
Renewable Energy Systems
Solar Inverters
Solar inverters are essential devices in solar energy systems. They convert direct current (DC) generated by solar panels into alternating current (AC), which is used in homes and businesses. The key characteristic of solar inverters is their ability to maximize the amount of power drawn from solar panels. This adaptability makes them a popular choice for integrating renewable energy into existing grids.
A unique feature of solar inverters is their ability to perform maximum power point tracking (MPPT). This technology adjusts the electrical operating point of the modules to ensure optimal output. The advantages of solar inverters include improved energy yield and lower grid dependence. However, they can also present challenges, such as potential efficiency losses during conversion.
Wind Energy Converters
Wind energy converters, also known as wind turbines, capture kinetic energy from wind and convert it into mechanical power, which is then transformed into electricity. A crucial aspect of wind energy converters is their variable-speed operation. This allows them to efficiently harness energy from varying wind speeds, contributing significantly to the electrical grid's stability.
The unique feature of wind energy converters is their direct-drive systems, which eliminate the need for a gearbox. This design simplifies maintenance and enhances reliability. The advantages of this approach include reduced mechanical wear and overall efficiency. However, installation costs can be high, impacting their feasibility in certain locations.
Electric Vehicles
Power Management
Power management in electric vehicles (EVs) focuses on optimizing energy use for performance and efficiency. This involves coordinating energy distribution among various components, such as motors, batteries, and ancillary systems. A key characteristic of power management is its role in prolonging battery life and ensuring effective energy recuperation during braking.
The unique feature of advanced power management systems is their predictive algorithms. These algorithms forecast energy needs based on driving conditions, enhancing overall vehicle efficiency. The advantages include better range and improved driving experience. However, developing sophisticated algorithms can entail significant research and development.
Charging Infrastructure
Charging infrastructure for electric vehicles is critical for promoting widespread adoption. It encompasses the network of charging stations that supply energy to EVs. A vital characteristic of charging infrastructure is its ability to facilitate rapid charging. This efficiency significantly reduces downtime for EV drivers, promoting convenience and accessibility.
The unique aspect of modern charging infrastructure is its integration with smart grid technology. This integration allows for better energy management and load balancing. The advantages include increased reliability and reduced energy costs. However, the high initial investment for infrastructure development can be a barrier in some regions.
Industrial Automation
Industrial automation involves the use of control systems for operating equipment in industries, such as machinery, processes, and information systems. Power electronics is integrated into automation frameworks to optimize processes. This results in improved efficiency and precision in manufacturing.
Power electronics enhances automation by enabling real-time control over machinery. This capability allows for adjustments based on varying operational demands. The benefits of this automation include reduced labor costs and increased productivity. However, the complexity of integrating power electronics systems can pose challenges for manufacturers.
Recent Innovations in Power Electronics
The field of power electronics is experiencing rapid developments. Innovations in this area primarily aim to enhance efficiency, reliability, and functionality of electronic systems. Recent innovations are pivotal as they pave the way for advancements across various sectors including renewable energy, electric vehicles, and industrial automation. This section explores two key innovation categories: Wide Bandgap Semiconductors and Advanced Control Techniques. Each provides unique advantages that are reshaping the landscape of power electronics.
Wide Bandgap Semiconductors
Wide bandgap semiconductors, such as silicon carbide (SiC) and gallium nitride (GaN), are revolutionizing power electronics. These materials have higher energy thresholds compared to traditional silicon. Their ability to operate at higher voltages and temperatures makes them ideal for applications requiring efficiency and high power density.
Some benefits of wide bandgap semiconductors include:
- Higher Efficiency: They reduce energy losses in switching operations.
- Improved Thermal Management: Their thermal conductivity allows for better heat dissipation, which is critical for high-performance systems.
- Compact Design: Smaller form factors help in reducing the overall size of power electronic devices.
Considering these elements, wide bandgap semiconductors contribute significantly to the advancement of power electronics in various applications, leading to more sustainable and energy-efficient systems.
Advanced Control Techniques
Control techniques play a crucial role in maximizing the performance of power electronics. Two notable methods are Model Predictive Control and Adaptive Control. Each presents distinct characteristics that contribute to improving the operation of power electronic systems.
Model Predictive Control
Model Predictive Control (MPC) is a control strategy that uses a model of the system to predict future behavior. In power electronics, it optimizes output in real time. One key characteristic of MPC is its ability to handle multi-variable control and constraints effectively. This flexibility makes it a popular choice for complex systems requiring optimal performance.
The unique features of MPC include:
- The ability to anticipate future events and plan accordingly.
- Support for multivariable systems, allowing for better management of interactions among various components.
MPC’s primary advantage is its capability of optimizing the control performance continuously. However, it may demand significant computational resources, potentially complicating its implementation in less sophisticated setups. Despite this, its precision and efficiency make it an appealing choice for various applications.
Adaptive Control
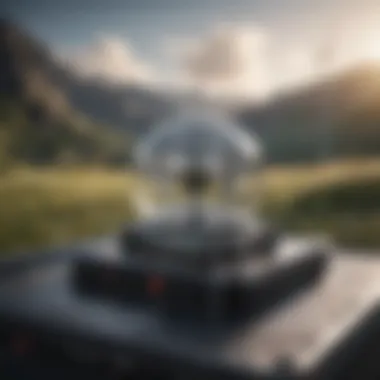
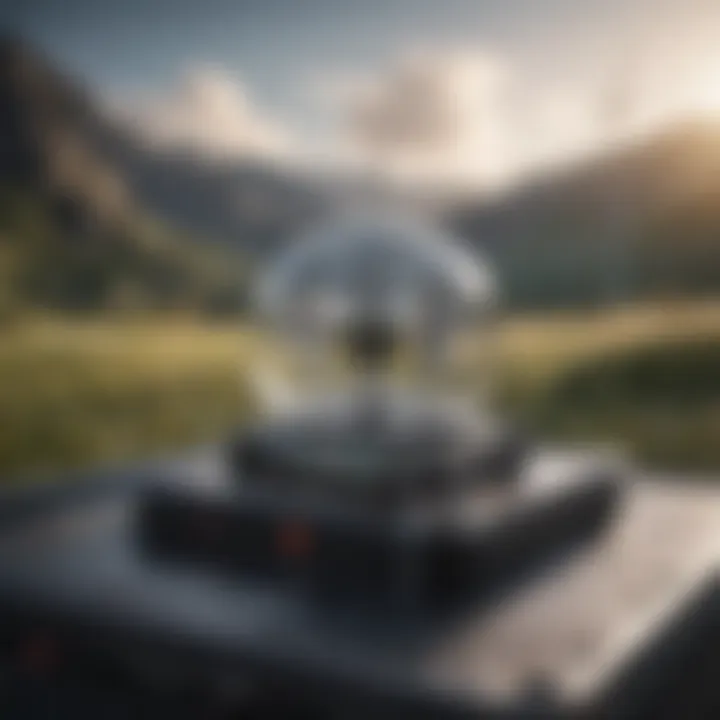
Adaptive Control is another innovative technique that adjusts the control parameters automatically based on real-time data. This is particularly useful in power electronics as it adapts to changing loads and system dynamics. A key characteristic of Adaptive Control is its ability to improve system performance over time as it learns from the operational conditions.
The benefits of Adaptive Control include:
- Enhanced system responsiveness to disturbances and changes in operating conditions.
- Continuous improvement in performance through real-time adjustments.
One consideration with Adaptive Control is its complexity and the need for careful tuning. The system must be set up to ensure stability while adapting to new conditions. Overall, Adaptive Control offers significant advantages that align well with the dynamic nature of modern power electronic systems.
The future of power electronics relies on innovations like wide bandgap semiconductors and advanced control methods to enhance performance and efficiency.
In summary, recent innovations in power electronics focus on materials and control strategies that support higher efficiency and adaptability. Understanding these developments is essential for anyone engaged in the field, as they directly impact the design and application of power electronic systems.
Challenges in Power Electronics
The field of power electronics faces several challenges that can significantly affect its development and application. Addressing these issues is crucial for improving performance and ensuring reliability in various applications. In this section, we will delve into two major challenges: thermal management and reliability issues. Each challenge holds its own complexities but also offers opportunities for innovation and technical advancements.
Thermal Management
Thermal management is a critical concern in power electronics systems as heat generation can impact both efficiency and longevity. Power electronic devices, such as transistors and diodes, convert electrical energy and inevitably produce heat in the process. Excessive heat can lead to overheating, which can cause failure or degrade performance. This circumstance creates a need for effective heat dissipation methods to maintain optimal operation.
One of the main strategies to combat thermal issues involves the use of advanced cooling techniques. These methods include air cooling, liquid cooling, and the implementation of heat sinks. Each technique has its own set of advantages and potential drawbacks. For example, liquid cooling tends to provide superior heat removal for high-power applications but can introduce complexity in design and maintenance.
Moreover, materials science plays a role in enhancing thermal management solutions. High thermal conductivity materials, such as graphene, are gaining attention as they can effectively transfer heat away from power devices. As research in this area progresses, the incorporation of these new materials could prove critical for the development of more efficient power electronic systems.
"Effective thermal management extends the lifespan of power electronic devices and improves overall system reliability."
Reliability Issues
Reliability is another major challenge that impacts power electronic systems. Components are often subject to a variety of stressors, including thermal cycles, electric fields, and physical strain. These factors contribute to wear and tear, potentially leading to premature failures.
One specific area of concern is the degradation of semiconductor materials due to high temperatures and electrical stresses. This degradation can manifest as shorter lifespans for devices and increased maintenance costs. Ensuring that components withstand such stresses without degradation requires thorough testing and robust design principles.
Research into improving the reliability of power electronic systems focuses on developing more robust materials and fault-tolerant designs. One promising approach is the use of redundancy in critical components. With backup systems in place, even if one component fails, the system as a whole can continue functioning.
Furthermore, predictive maintenance models that incorporate data analytics can play a vital role in reliability management. By analyzing performance data, potential failures can be predicted, allowing for timely intervention. This not only prevents unexpected downtime but also enhances the overall trustworthiness of power electronic systems.
The Future of Power Electronics
The future of power electronics is pivotal as it aligns with sustainable energy initiatives and smarter technology integrations. As society shifts towards a more energy-efficient environment, power electronics will play a crucial role in enhancing the performance, reliability, and functionality of essential systems. This section will explore emerging trends and research directions that are shaping the landscape of power electronics.
Emerging Trends
Integration with IoT
The integration of power electronics with the Internet of Things (IoT) represents a significant advancement. IoT allows devices to communicate and interact effectively, leading to improved efficiency in energy management systems. A key characteristic of this integration is its ability to enable real-time monitoring and control of power circuits. This leads to a more responsive energy management framework, which can optimize energy usage and reduce waste.
One unique feature of the integration with IoT is the ability to gather data from various sources, which can then be analyzed to improve operational efficiency. For instance, smart appliances can adapt their performance based on live consumption data, resulting in better energy savings. However, challenges exist, particularly related to security and data privacy. Protecting sensitive information in connected systems becomes critical as these systems grow in complexity.
Smart Grids
Smart grids signify a transformative leap in power distribution and management. A distinctive aspect of smart grids is their ability to integrate distributed generation sources, such as solar panels and wind turbines. This flexibility in resource management adds resilience and sustainability to the grid. Moreover, smart grids facilitate better communication between energy suppliers and consumers, contributing to a more stable energy supply.
The unique feature of smart grids is the incorporation of advanced sensors and control technologies, which provide detailed information about energy flows. This enables operators to react swiftly to fluctuations in demand or generation. However, the deployment of smart grids is capital intensive and requires careful planning to ensure interoperability among different technologies.
Research Directions
Research in power electronics is heading towards innovative solutions that address current challenges and explore new frontiers. Topics of interest include the optimization of power conversion methods and enhancing thermal management strategies. Furthermore, there is a growing emphasis on the development of materials that can improve the efficiency and thermal performance of devices.
The convergence of disciplines, such as materials science and electrical engineering, will continue to fuel advancements in this field. Future studies may also explore the implications of artificial intelligence in power electronics design and management, offering new ways to enhance system performance and intelligence. As research progresses, collaboration across sectors will be essential to fully realize the potential of power electronics in an increasingly digital and sustainable world.
Ending
The conclusion encapsulates the essential aspects discussed throughout this article on power electronics. It provides a comprehensive overview of the pivotal role power electronics plays in various applications, from renewable energy systems to industrial automation. Through a meticulous examination of the innovations and challenges, the article highlights the transformative potential of power electronics technology.
Summary of Key Points
- Defining Power Electronics: The article begins with a clear definition of power electronics and its historical context, establishing a foundational understanding.
- Core Components: It details the crucial components such as power semiconductor devices, passive components, and control circuits, explaining their functions and significance in systems.
- Operating Principles: A discussion on conversion processes and switching techniques sheds light on how power electronics operates in real-world applications.
- Application Spectrum: The varied applications in renewable energy, electric vehicles, and industrial automation showcase the versatility of power electronics technology.
- Innovations and Challenges: The exploration of wide bandgap semiconductors and advanced control techniques illustrates recent breakthroughs, while challenges like thermal management and reliability are candidly acknowledged.
- Future Prospects: Emerging trends such as integration with IoT and smart grids are considered, indicating where research efforts may lead in the coming years.
This summary serves as a reminder of the article’s core themes, bringing focal awareness to how power electronics is embedded in the fabric of modern technology.
Implications for Future Research
Research in power electronics is increasingly important as the push towards energy efficiency intensifies. A few suggestions for future directions include:
- Integration of AI Techniques: Exploring the use of artificial intelligence to optimize control systems and improve efficiencies in power electronics applications.
- Enhanced Material Research: Investigating new materials for power devices that can improve performance, efficiency, and sustainability.
- Assessment of Environmental Impact: Understanding the lifecycle of power electronics products will be important for ensuring sustainable development.
- Scalability of Innovations: Research should focus on how to scale proven innovations for commercial applications without incurring excess costs.
Through these research directions, the ongoing evolution of power electronics can contribute significantly to meeting future energy demands and environmental challenges. As industries adapt to new technologies, the insights gained from continued research will be vital.