Exploring the Molecular Biology of the Cell
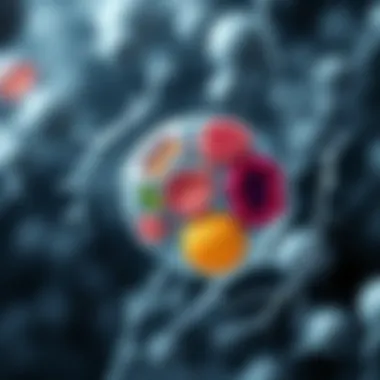
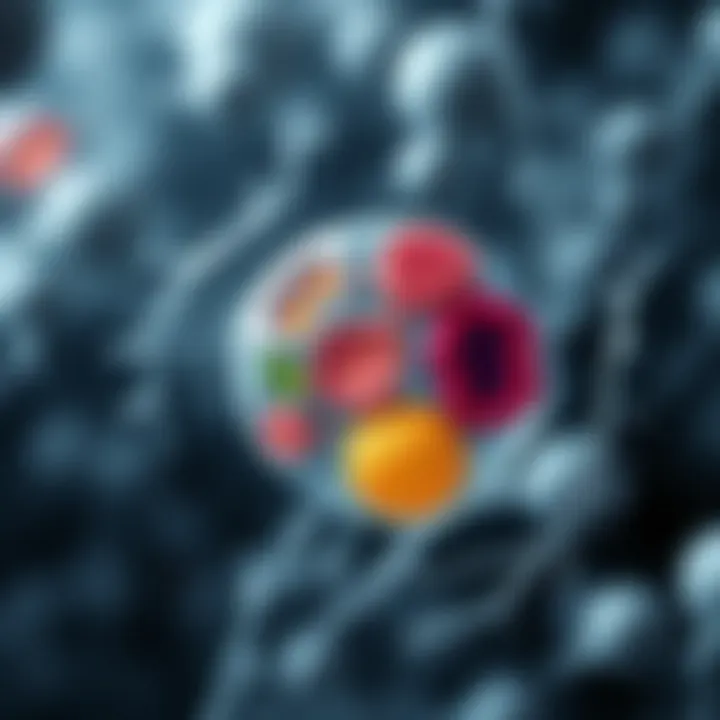
Intro
The field of molecular biology is a cornerstone of modern science, providing insights into the very essence of life itself. When we peel back the layers surrounding cellular function, a world of intricate details and interactions awaits us, echoing the complexity that defines biological systems. From tiny nucleotides to vast networks of proteins, every component plays a unique role in orchestrating life.
In this exploration of the molecular biology of the cell, we delve deep into the key structures, signaling pathways, and mechanisms that govern cellular operations. By uncovering such elements, we not only enhance our understanding but also address current challenges faced in biological research and therapeutic developments.
It’s like unraveling a complicated puzzle; each piece contributes to a larger picture that informs our grasp of health, disease, and everything in between.
Prelims to Molecular Biology
Molecular biology stands as a cornerstone in understanding the intricate mechanisms of life at a cellular level. This field not only emphasizes the basic components of life, like DNA, RNA, and proteins, but also explores how these molecules interact to foster cellular processes critical for existence. Our lives, from the humble heartbeats to the complex functioning of our brains, all hinge on molecular activities, making the understanding of molecular biology essential.
When diving into the depths of molecular biology, one quickly realizes it’s not just a niche area but a crucial segment that holds immense implications in various domains, including genetics, medicine, and biotechnology. The unraveling of this field provides insights that can lead to breakthroughs in treating diseases, understanding genetic disorders, and contributing to advancements in technology.
In this article, we aim to break down the components and processes involved in molecular biology. By examining the cellular architecture, the genetic underpinnings, and the signaling pathways, we offer a comprehensive exploration that reflects the profound interplay between structure and function. The knowledge garnered here will not only enhance your understanding but also equip you with the necessary insights to engage with ongoing discussions and research.
Defining Molecular Biology
Molecular biology can be succinctly defined as the study of biological processes at the molecular level. This discipline reflects a deeper inquiry into the interactions between various biological molecules. At its core, molecular biology melds the principles of biochemistry, genetics, and cell biology, creating a tapestry of knowledge that explains how life operates on a minute scale.
Understanding the definitions and nuances of molecular biology requires a grasp of several essential concepts:
- DNA and RNA: Molecules that store and transmit genetic information.
- Proteins: The workhorses of the cell that perform a myriad of functions, from acting as enzymes to providing structural support.
- Gene Expression: The process by which information from a gene is used to synthesize a functional product, typically a protein.
These elements interlink, forming the backbone of cellular processes. When dissecting each part, one can appreciate how mutations, regulatory mechanisms, and molecular interactions drive the overall biological phenomena observed in living organisms.
Historical Context
To truly grasp the significance of molecular biology today, it’s illuminating to reflect on its historical roots. The evolution of this field is marked by landmark discoveries that paved the way for our contemporary understanding.
- Early Discoveries: In the early 20th century, scientists such as Gregor Mendel initiated inquiries into hereditary traits, which laid the groundwork for genetics. His pea plant experiments hinted at the underlying principles passed through generations.
- The Watson-Crick Model: The elucidation of the double-helix structure of DNA by James Watson and Francis Crick in 1953 was a seismic shift. This discovery not only described the structural basis of genetic information but also ignited a flurry of research into how genetic material is replicated and expressed.
- Biotechnology Boom: The late 20th century bore witness to an explosion in biotechnical advancements, including recombinant DNA technology and the Human Genome Project, which sequenced the entire human DNA.
Each of these milestones signifies monumental progress in understanding molecular biology, leading to the sophisticated techniques and applications we see today. The journey from basic principles to complex applications in medicine and technology showcases the remarkable growth and relevance of this field in the modern scientific landscape.
"Molecular biology is not merely a scientific discipline; it's a lens through which we explore the complexities of life, unraveling the mysteries of biology one molecule at a time."
This overview sets the stage for deeper exploration into specific cellular structures and functions, and how these contribute to the broader canvas of life itself.
Cell Structure and Function
In molecular biology, understanding the structure and function of cells is crucial. Cells are the basic units of life, and each cell's architecture plays a significant role in its function. By piecing together knowledge about cell structure, we can make sense of how cells operate, how they communicate with one another, and the various processes they perform to sustain life. This knowledge is key in fields like genetics, medicine, and bioengineering, where insights into cellular behavior can lead to breakthroughs in treating diseases or developing new technologies.
The Cell Membrane
The cell membrane, often referred to as the gatekeeper, serves as the boundary that separates a cell from its external environment. It plays a pivotal role not only in defining cell shape but also in regulating what enters and exits the cell.
Composition of Lipids and Proteins
The cell membrane is primarily composed of lipids and proteins, forming a bilayer. This lipid bilayer consists mainly of phospholipids, which have hydrophilic (water-attracting) heads and hydrophobic (water-repelling) tails. The arrangements of these lipids create a semi-permeable barrier that allows certain molecules to pass while keeping others out. This characteristic is essential for maintaining the internal environment of the cell.
Moreover, proteins embedded within this bilayer act as channels, receptors, and enzymes. For instance, integral proteins span the membrane, facilitating the transport of substances across it. This unique feature plays a significant role in signaling pathways and cellular communication, allowing cells to respond to their environment.
Advantages and Disadvantages: The flexibility of the lipid bilayer is a boon as it permits structural integrity while also allowing for dynamic changes and adaptations to external stimuli. However, this same characteristic can also be a double-edged sword, as the membrane can become more permeable in certain pathological conditions.
Membrane Transport Mechanisms
Transport mechanisms are fundamental to cellular function, underpinning how substances move across the membrane. These mechanisms can be categorized into passive transport, active transport, and facilitated diffusion.
Passive transport does not require energy and relies on concentration gradients, such as the movement of oxygen into cells and carbon dioxide out. Active transport, on the other hand, involves energy expenditure to move substances against their concentration gradient. An example is the sodium-potassium pump, which is critical for maintaining cellular ion balance.
The unique features of these transport systems allow the cell to control its internal environment meticulously. They ensure essential nutrients enter while waste products exit, maintaining homeostasis.
Advantages and Disadvantages: While passive transport is energy-efficient, it may not be sufficient for all cellular needs. Active transport mechanisms, however, can be energy-intensive and, if dysfunctional, can lead to various diseases.
Organelles and Their Functions
Organelles are specialized structures within a cell, each performing distinct functions that contribute to the cell's overall health and efficiency. Their presence allows for compartmentalization of processes, enabling cells to perform various functions simultaneously.
Mitochondria
Mitochondria often called the powerhouses of the cell, are responsible for generating ATP, the primary energy carrier. They perform cellular respiration, a process essential for converting biochemical energy from nutrients into adenosine triphosphate.
A unique feature of mitochondria is their double-membrane structure, which includes an inner membrane that houses proteins for ATP production. Additionally, they have their own DNA, hinting at a symbiotic evolutionary history, suggesting once they were free-living prokaryotes.
Advantages and Disadvantages: The capacity of mitochondria to adapt their function based on energy demand is remarkable. However, dysfunction in mitochondrial processes can lead to a range of metabolic diseases and contribute to aging.
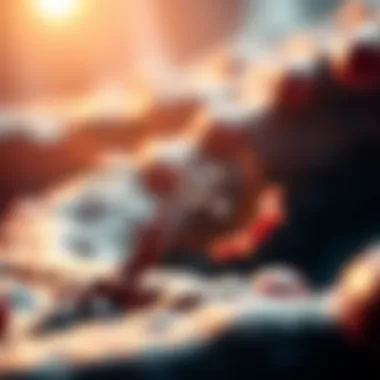
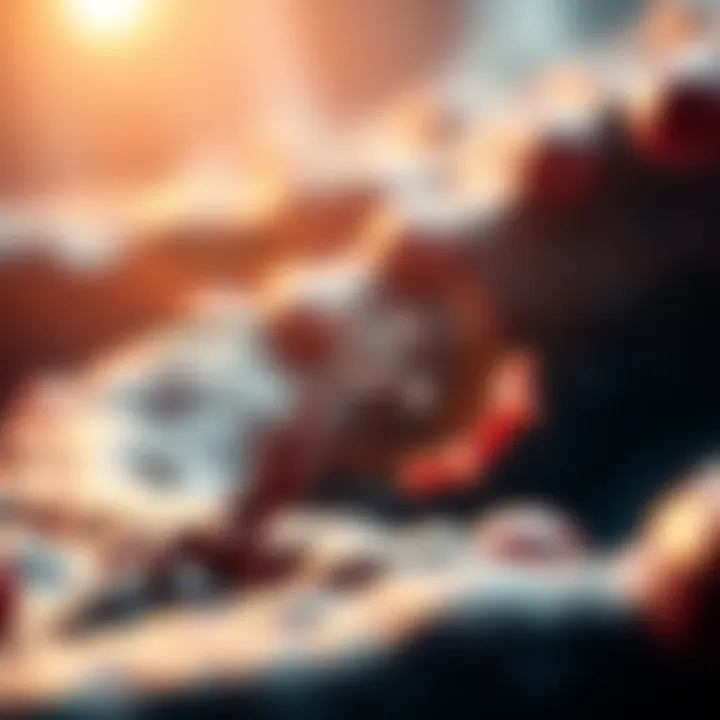
Nucleus
The nucleus is often recognized as the control center of the cell, housing the genetic material. It is encased by a double membrane, known as the nuclear envelope, which protects the DNA while also regulating material exchange through nuclear pores.
One of the noteworthy features of the nucleus is its nucleolus, where ribosomal RNA is synthesized. This nucleus-nucleolus relationship is critical for protein synthesis, as ribosomes are essential for translating genetic information into functional proteins.
Advantages and Disadvantages: While the nucleus serves as a protective hub for genetic material, it also presents challenges in regulating protein synthesis and maintaining DNA integrity, important factors that correlate with various diseases.
Endoplasmic Reticulum
The endoplasmic reticulum (ER) consists of a network of membranes that play roles in synthesizing proteins and lipids. There are two distinct types of ER: rough ER, which is studded with ribosomes, and smooth ER, which is involved in lipid synthesis.
The presence of ribosomes on the rough ER allows for the immediate translation of mRNA into proteins destined for secretion or membrane insertion. In contrast, smooth ER functions in lipid metabolism and detoxification processes.
Advantages and Disadvantages: The compartmentalization of functions within the ER enhances efficiency, yet the complexity can lead to disruptions in cellular processes if there are issues with quality control or folding of proteins.
Golgi Apparatus
The Golgi apparatus is crucial for processing and packaging proteins and lipids produced in the endoplasmic reticulum. It sorts these molecules and adds carbohydrate and phosphate groups in a process called glycosylation, which influences their function and destination.
The unique feature of the Golgi apparatus is its stacked membrane-bound cisternae that allow it to modify cargo as they transit through it. The Golgi is often referred to as the shipping center of the cell, dispatching finished products to their intended locations.
Advantages and Disadvantages: Its ability to modify and sort proteins makes the Golgi apparatus essential for precise cellular function. However, errors in processing can lead to misdirected proteins and subsequent cellular dysfunctions.
Cytoskeleton Dynamics
The cytoskeleton provides structural support to the cell, while also playing integrative roles in transport and motility. It consists of three main components: microfilaments, microtubules, and intermediate filaments.
Microfilaments
Microfilaments are primarily composed of actin filaments, and they are crucial for maintaining cell shape and enabling movement. Their dynamic nature allows them to rapidly assemble and disassemble as needed, which is critical during cell division and motility.
Advantages and Disadvantages: This adaptability is a significant advantage in processes like wound healing, but can also lead to challenges, such as cancer metastasis where cells migrate inappropriately.
Microtubules
Microtubules are tube-like structures made from tubulin protein, and they are vital for various cellular functions like providing tracks for vesicle transport, maintaining the shape of the cell, and facilitating cellular division.
Advantages and Disadvantages: Their ability to grow and shrink rapidly is beneficial for quick cellular responses but may confuse regulatory mechanisms linked to cell division, potentially leading to diseases like cancer.
Intermediate Filaments
Intermediate filaments provide tensile strength to cells and help withstand mechanical stress. Composed of various proteins, their composition varies depending on the cell type.
Advantages and Disadvantages: The structural integrity they offer is critical for maintaining cellular organization, but their inflexible nature can sometimes hinder cell movement, making it a complex trade-off in cellular dynamics.
Genetic Basis of Molecular Biology
Molecular biology's fundamental cornerstone lies in understanding the genetic material that dictates not just the structure but also the function of living organisms. Essentially, this area of study highlights how genes serve as blueprints for the assembly of proteins, which in turn orchestrate a myriad of cellular activities. Knowing the genetic basis of molecular biology informs us on everything from basic cellular functions to complex biological systems.
The crux of molecular biology is in delineating how genes are expressed and regulated, as this directly influences every aspect of cell life. With the integration of molecular techniques and genomic data, this field is unfolding details that have great implications in medicine, biotechnology, and environmental science.
DNA Structure and Replication
In the realm of molecular biology, deoxyribonucleic acid (DNA) is like a revered manuscript containing the instructions for life. Its structure, characterized by the iconic double helix, encompasses a sequence of nucleotides that encodes genetic information. The double helix features complementary base pairing—adenine with thymine, and cytosine with guanine, creating a stable structure critical for accurate replication.
Replication itself is a high-stakes endeavor for the cell, tasked with ensuring that each daughter cell inherits a complete and unblemished copy of the genetic code. Errors during this process can lead to mutations, which might have serious repercussions, ranging from benign to life-threatening. A series of proteins coordinate this complicated mechanism, showcasing the intricacies involved in maintaining genetic fidelity.
Transcription and Translation
Transcription and translation are two fundamental processes that convert the genetic information held within DNA into functional proteins. During transcription, a segment of DNA is unraveled and used as a template to synthesize messenger RNA (mRNA). This process is like rewriting a recipe from an ancestral cookbook—ensuring that the essential ingredients (the genes) are maintained while adapting them for the modern kitchen (the cellular machinery).
RNA Polymerase Function
RNA polymerase plays a pivotal role in transcription. It is the enzyme responsible for catalyzing the formation of mRNA from the DNA template. One key characteristic of RNA polymerase is its remarkable ability to initiate transcription without a primer, which sets it apart from DNA polymerase.
Its unique feature lies in its multi-subunit structure, allowing it to regulate the transcription of various genes effectively. This adaptability makes it an essential component for cell responses to environmental changes, and its dysfunction can result in transcriptional errors leading to diseases.
Ribosome Structure and Role
Once mRNA is synthesized, it travels to the ribosome, the cellular factory for protein synthesis. Ribosomes themselves are composed of ribosomal RNA (rRNA) and proteins, forming two distinct subunits that collaborate to translate the mRNA sequence into a polypeptide chain. The ribosome’s key characteristic is its dual function: binding mRNA and facilitating the attachment of transfer RNA (tRNA), which carries the necessary amino acids for protein assembly.
Its unique feature is that ribosomes can operate on several mRNA strands simultaneously, a process known as polyribosome formation, which amplifies protein production. However, the potential downside is that errors during translation can lead to dysfunctional proteins that have negative consequences for cellular function, highlighting the need for finely-tuned regulatory mechanisms.
Protein Synthesis and Folding
Protein synthesis is the final act in the orchestration of molecular biology. Once polypeptides are formed, they need proper folding to become functional proteins. Folding is inherently complex and can be influenced by various factors including the cellular environment and the amino acid sequence. When proteins fold mishap, aggregates can form, which might contribute to various diseases, like Alzheimer’s or Parkinson’s. Understanding how proteins achieve their functional conformations not only informs biological sciences but also aids in developing therapeutic interventions.
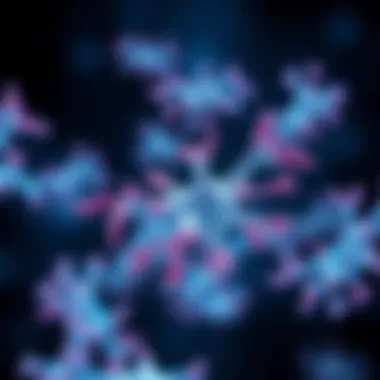
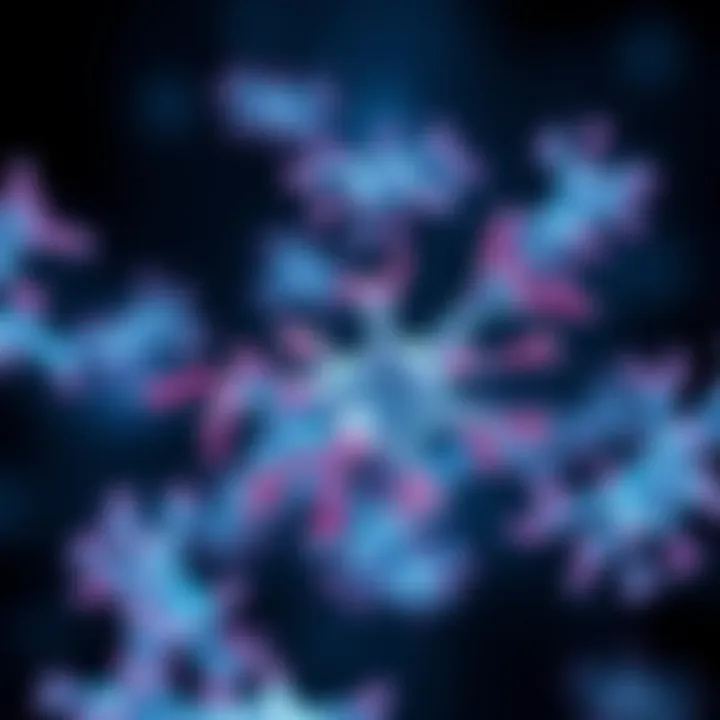
Like a well-tuned orchestra, the elements of protein synthesis—from transcription to translation and beyond—work in harmony to uphold life's processes, making the genetic basis of molecular biology an indispensable framework for unraveling the science of life itself.
Cell Signaling Pathways
Cell signaling pathways are the intricate communication networks that govern numerous cellular processes, from growth to immune responses. This section delves into the various types of signaling mechanisms cells employ and the role receptors play in these processes. Understanding these pathways is crucial, as they lay the foundation for how cells convey information, react to their environment, and maintain homeostasis. Without these signaling mechanisms, life as we know it wouldn’t hold together.
Types of Cell Signaling
This subsection discusses the three primary types of cell signaling: autocrine, paracrine, and endocrine. Each plays a unique role in cellular communication, impacting everything from tissue growth to hormone regulation. Let's break these down:
Autocrine
Autocrine signaling is when a cell releases signals that bind to receptors on its own surface, effectively telling itself to respond in a specific way. A key characteristic of autocrine signaling is its self-sufficiency; it's like a cheerleader encouraging her own team. This type is a beneficial choice in the context of tumor growth, where cancer cells often rely on autocrine signals to proliferate without dependence on external signals.
Unique Features and Considerations:
- A significant advantage of this signaling is speed; the response time is typically rapid since it acts on the same cell.
- However, the disadvantage lies in the fact that overreliance can lead to unregulated cell growth, as seen in certain cancers.
Paracrine
Paracrine signaling occurs when a cell communicates with nearby cells by releasing signaling molecules into the local environment. This is akin to a neighbor calling across the fence—it's a direct but limited communication. The beauty of paracrine signaling lies in its ability to create a localized response, which is essential for coordinating activities in tissues, such as during wound healing.
Key Characteristics and Insights:
- An important characteristic is specificity; signals only affect nearby cells which helps prevent systemic reactions that could be detrimental.
- The disadvantage, however, is the ease of interference; factors like distance can limit the effectiveness of these signals, especially in larger tissues.
Endocrine
Endocrine signaling involves hormones released into the bloodstream, where they travel to distant sites in the body. This method of signaling is quite like dispatching a letter over a long distance. Hormones like insulin and adrenaline are prime examples of this type of signaling. The key characteristic here is the broad reach, as these signals can affect cells throughout the body.
Unique Features and Pros/Cons:
- The significant advantage is that the action can be profound on multiple systems at once, allowing for a coordinated response to events like stress.
- However, the time needed for these hormones to circulate can be quite lengthy, making immediate responses difficult.
Receptors and Their Mechanisms
Receptors are essential components in signal transduction; they act as gateways for the signals to be relayed within the cell. Various receptor types exist, and the mechanism through which they operate can significantly influence the cellular response. These receptors can either be on the cell surface or within the cell interiors and are finely tuned to respond to only specific signaling molecules.
In summary, cell signaling pathways are vital for maintaining the delicate balance of cellular activities. Each type of signaling—autocrine, paracrine, and endocrine—serves distinct purposes, with their own set of advantages and limitations. The effectiveness of these pathways is heavily dependent on the receptors involved, which makes the understanding of these mechanisms crucial for numerous fields, including medicine and biotechnology.
"The dance of communication at the cellular level can dictate the rhythm of life itself. Understanding these pathways is not just science; it is the essence of life."
In depth exploration into cell signaling can yield insights into how to manipulate these pathways for therapeutic purposes and generate new treatment strategies.
Techniques in Molecular Biology
The area of Techniques in Molecular Biology is indispensable for any comprehensive exploration of the discipline. These methodologies serve as vital tools that enable researchers to manipulate, analyze, and visualize biomolecules, facilitating advancements across various sectors including healthcare, environmental science, and genetic research. In this section, we will delve into three fundamental techniques which have become the backbone of molecular biology: Polymerase Chain Reaction (PCR), Gel Electrophoresis, and DNA Sequencing Methods. Each of these methods not only showcases the complexity and sophistication of modern biology but also the dynamic impact they have in practical applications.
Polymerase Chain Reaction (PCR)
Polymerase Chain Reaction, often referred to as PCR, is a revolutionary technique that has transformed genetic analysis. This method allows for the amplification of a single or a few copies of DNA across several orders of magnitude, generating thousands to millions of copies of a particular DNA sequence. The significance of PCR lies in its versatility; it is employed in various applications from medical diagnostics to forensic science.
Key Steps in PCR:
- Denaturation: This involves heating the reaction mixture to separate the DNA strands.
- Annealing: The temperature is lowered to allow primers to bind to the target DNA sequence.
- Extension: The temperature is adjusted again for DNA polymerase to synthesize new DNA strands, starting from the primers.
This technique is not just a lab curiosity; it has crucial implications for infectious disease diagnosis, paternity testing, and research into genetic disorders. The precision of PCR turns it into a double-edged sword too, as even tiny contaminants can lead to erroneous results. So, maintaining strict procedural discipline is a must.
Gel Electrophoresis
Gel Electrophoresis is a method used to separate mixtures of DNA, RNA, or proteins according to their size. By applying an electric current to a gel matrix, molecules move through the gel at different rates, allowing scientists to distinguish between them. This separation is critical for analyzing genetic material, visualizing gene fragments, and validating PCR results.
Why Gel Electrophoresis Matters:
- Visualization: It provides a tangible representation of nucleic acids and proteins, critical for analysis.
- Purification: Unwanted fragments can be removed, ensuring purity of the sample for downstream applications.
- Comparative Studies: It allows for the comparison of different samples, vital in genetic research and diagnostics.
One must keep in mind the ethical and safety considerations related to handling biological materials and the interpretation of results. Misinterpretation can lead to significant downstream misconceptions, particularly in clinical settings.
DNA Sequencing Methods
DNA sequencing has advanced leaps and bounds since the first Sanger method was developed. With the arrival of next-generation sequencing technologies, the landscape of genomics has seen explosive growth. Today, sequencing methods allow researchers to determine the exact sequence of nucleotides in a DNA molecule, which is pivotal for genomics, personalized medicine, and evolutionary biology.
Prominent Sequencing Techniques:
- Sanger Sequencing: While traditional, it still remains relevant for small-scale projects.
- Next-Generation Sequencing (NGS): High throughput and massively parallel, ideal for whole-genome sequencing.
- Third-Generation Sequencing: Such as Nanopore sequencing, allows reading long DNA strands directly, increasing read lengths considerably.
Piggybacking on these advancements, applications range from identifying genetic mutations to cancer research and the exploration of microbial diversity. However, technologies do come with a cost. The data output is immense and requires advanced analytical skills and tools to process and understand, blurring the line between biological research and data science.
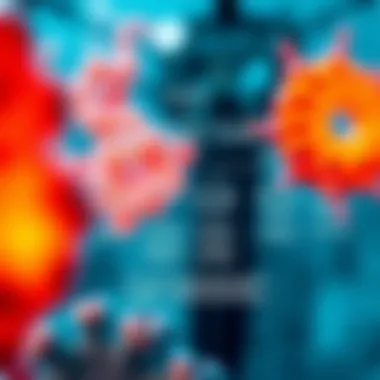
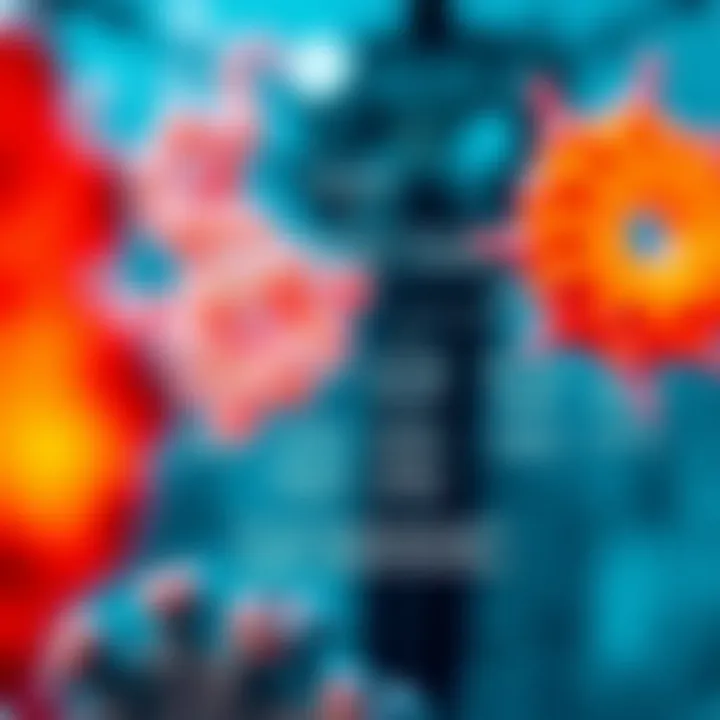
"The techniques covered are not just mere methodologies, but the pulse of molecular biology, driving discoveries that shape our understanding of life itself."
Through an understanding of these techniques, we gain a clearer picture of their importance in unraveling cellular complexities. As scientists push the boundaries of our current knowledge, the role that these methods play will only grow, shaping the future of not just molecular biology, but medicine and global health as well.
The Role of Molecular Biology in Medicine
Molecular biology has swiftly become a cornerstone in medical research and treatment. Its influence stretches across various medical fields, allowing for precise manipulations of cellular and molecular processes. This section delves into key areas where molecular biology is significantly shaping medical practices, offering not just a glimpse into its capabilities but also addressing its pivotal role in modern healthcare.
Molecular biology intersects with medicine in a myriad of ways, essentially unlocking the secrets of biological processes that have profound implications for diagnosing and treating diseases. The benefits are apparent: improved outcomes, targeted therapies, and even the potential for preventative measures that were mere aspirations a few decades ago.
"Molecular biology serves as the bridge between understanding the microcosm of cells and the macrocosm of human health."
Gene Therapy
Gene therapy represents one of the most promising applications of molecular biology in medicine, aiming to correct or replace defective genes responsible for disease development. The process can involve either directly delivering healthy copies of a gene or using gene-editing technologies like CRISPR-Cas9 to modify the faulty genes.
A prime example is the use of gene therapy in treating inherited disorders such as cystic fibrosis or muscular dystrophy. Adjustments at the molecular level can alleviate symptoms or potentially cure these conditions. A thorough understanding of gene function and regulation allows researchers to craft tailored approaches to specific genetic maladies.
Some considerations here include ethical concerns surrounding genetic manipulation and the long-term effects on individuals and populations. That's why discussions about safety, efficacy, and regulations are equally vital as the scientific advancements.
Cancer Molecular Biology
Cancer is not merely a disease of uncontrolled cell growth; it is fundamentally rooted in molecular errors. The field of cancer molecular biology aims to discern the molecular underpinnings that lead to cancer development, progression, and metastasis. By identifying specific genetic mutations, researchers are able to develop targeted treatments that inhibit the pathways that cancer cells exploit for their growth.
For example, therapies such as trastuzumab are designed to target specific proteins on cancer cells. These treatments display how a deep understanding of cellular processes can lead to breakthroughs that change the landscape of cancer treatment.
However, this approach also brings its own challenges. The genetic diversity among tumors means that what works well for one patient might not work for another, underscoring the necessity for personalized approaches in oncology and the ongoing research to further understand tumor biology.
Personalized Medicine
Personalized medicine, an area heavily reliant on molecular biology, involves tailoring medical treatment to the individual characteristics of each patient. This concept moves away from the one-size-fits-all model, allowing treatments to be calibrated based on a person's genetic makeup, lifestyle, and environment.
For instance, pharmacogenomics is an exemplary area where genetic profiles guide medication types and dosages, optimizing their effectiveness while minimizing adverse reactions. A common scenario involves testing patients for specific biomarkers before administering chemotherapy to make sure that they receive the most effective treatment for their cancer type.
This level of customization in healthcare does raise questions about data privacy and the ethical implications of genetic testing. As the field moves forward, careful thought must be given to how such information is handled, ensuring that patient rights are preserved along with their health.
As research in molecular biology progresses, the trajectory it sets for the future of medicine is not only exciting but also essential for improving health outcomes on a broader scale.
Interdisciplinary Connections
In the realm of scientific inquiry, the intersection of fields often leads to breakthroughs that push the boundaries of what we know. This is particularly true in molecular biology, where connections with other disciplines enrich research and application. The necessity for Interdisciplinary Connections arises from the multi-faceted nature of cellular processes. Essentially, understanding molecular biology isn't just about DNA, RNA, and proteins in isolation; it involves integrating knowledge from genetics, biochemistry, and bioinformatics. Such collaboration yields deeper insights and fosters innovation across various applications, from healthcare to biotechnology.
Molecular Biology and Genetics
Genetics is like the backbone of molecular biology. In fact, the two fields are inextricably linked. To grasp molecular biology, one must appreciate how genetic information is stored, expressed, and regulated within cells. Molecular biology dives deep into the mechanisms of gene expression — how genes are turned on and off, how they are replicated, and how they ultimately synthesize proteins.
- Gene Regulation: This aspect of genetics involves understanding promoters and enhancers. Regulatory elements determine when and where specific genes are expressed. Disruptions in these mechanisms frequently lead to diseases, further highlighting their importance in molecular biology.
- Genetic Engineering: The capacity to manipulate genes has revolutionized medicine. Techniques like CRISPR allow for precise editing, making it possible to target genetic disorders at their source.
Biochemistry's Influence on Molecular Biology
Biochemistry lays the groundwork for understanding the chemical underpinnings of biological processes. This discipline looks at the molecules that define life, catering to an insight that molecular biology builds upon. The interplay of enzymes, metabolic pathways, and energy production fuels cellular activity.
- Enzyme Function: Enzymes act as catalysts, speeding up chemical reactions in cells. Knowledge of enzyme kinetics and structure helps in designing drugs that can inhibit or enhance their activity, illustrating the collaboration between biochemistry and molecular biology.
- Metabolic Pathways: These pathways are a network of biochemical reactions. From glycolysis to the Krebs cycle, understanding these processes helps in revealing how cells produce energy and react to their environments.
Crossing Boundaries with Bioinformatics
Bioinformatics is the bridge that links molecular biology with computational science. It provides tools and techniques to analyze biological data on a grand scale, a necessity in today's genomic era. With vast amount of data generated daily, manual analysis becomes impractical, which is where bioinformatics thrives.
- Data Analysis: Bioinformatics techniques enable the interpretation of complex datasets, such as those from DNA sequencing projects. Advanced algorithms allow researchers to identify patterns and correlations that would be impossible to discern by traditional means.
- Modeling Biological Processes: Through simulations and computational models, scientists can examine how different factors might influence cellular behavior. For instance, predicting how genetic mutations may alter protein functions can have huge impacts in drug development and personalized medicine.
Combining Molecular Biology with Genetics, Biochemistry, and Bioinformatics not only advances our knowledge but also propels us into the future of scientific innovation.
Future Directions in Molecular Biology
The field of molecular biology is ever-evolving, a truth that cannot be ignored. As we step into this new age, the future holds a treasure trove of advancements that will continuously reshape our understanding of life at the molecular level. Insights gained from molecular biology are not just academic; they influence various domains, from healthcare to agriculture, and impact our everyday lives in profound ways.
Emerging Technologies
One of the pivotal elements steering the future of molecular biology is emerging technology. These breakthrough tools and methodologies are pushing the boundaries of what we thought possible.
- CRISPR-Cas9: This gene-editing technology has sparked a revolution. It allows for precise modifications to DNA with unprecedented accuracy. Researchers can potentially eradicate genetic disorders, enhance crop resilience, or even modify organisms in ways previously thought impossible. The implications are boundless.
- Single-cell sequencing: Understanding cellular diversity is key. This technology permits the analysis of individual cells, uncovering variations previously obscured in bulk analyses. It has significant relevance in cancer research, as different tumor cells may respond differently to treatments.
- Proteomics and Metabolomics: These fields are gaining traction, as analyzing all proteins and metabolites in a cell can provide insight into the function and regulation within biological systems. Integrating these disciplines with genomic data can lead to a more holistic view of biology.
- Artificial Intelligence (AI): The role of AI in molecular biology cannot be overlooked. It has the capability to analyze vast amounts of data, identify patterns, and predict outcomes more efficiently than traditional methods.
These technologies are not just tools; they are gateways to new discoveries that could change our understanding of biology, offering solutions to complex problems and enhancing our approach to disease treatment, agricultural strategies, and beyond.
Potential Ethical Considerations
As we embrace these advancements, it's essential to tread carefully through the moral landscape they create. The convergence of biology and technology raises questions that demand thorough scrutiny. Issues include:
- Gene Editing Ethics: With tools like CRISPR, the ability to alter human genes leads to debates about designer babies, genetic enhancements, and the potential for unintended consequences. What guidelines should govern such powerful technologies?
- Privacy and Data Security: With vast datasets being generated, particularly in genomics, safeguarding personal genetic information becomes imperative. How do we protect individuals' rights without stifling scientific progress?
- Biodiversity and Ecosystem Impact: The manipulation of organisms can have far-reaching impacts on natural ecosystems. Introducing modified organisms into the wild could disrupt existing balances, leading to unforeseen consequences.
- Accessibility and Inequality: As advancements surge, who gets access to these breakthroughs? Ensuring that all sectors of society benefit from new technologies remains a pressing issue.
"The advancement of molecular biology should not just be about scientific achievement, but also about responsible stewardship of the tools we create."
In closing, the future directions in molecular biology are filled with promise and complexity. Emerging technologies stand to revolutionize various fields, but ethical considerations must be at the forefront of discussions. Balancing innovation with responsibility is crucial as we navigate these exciting frontiers.