Comprehensive Guide to mRNA and Amino Acids
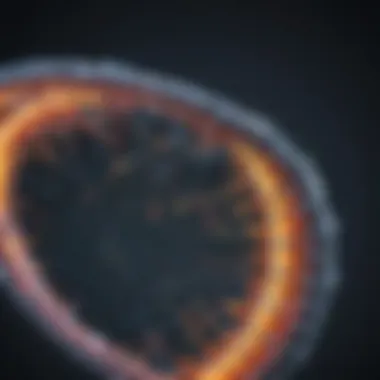
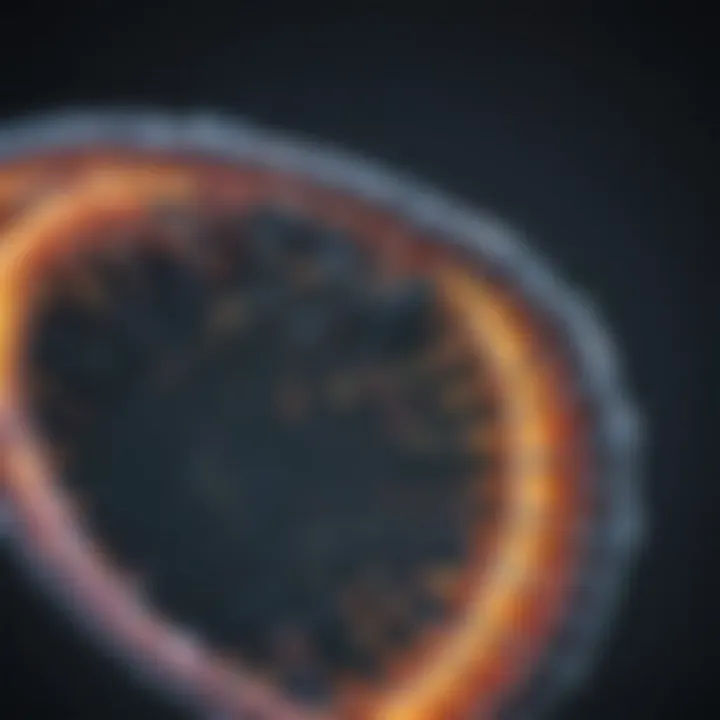
Intro
The study of genetics is a riveting journey through the very fabric of life itself. In this exploration, the role of messenger RNA (mRNA) takes center stage as it serves as the vital bridge between the intricacies of genetic code and the synthesis of proteins. Understanding how mRNA converts into amino acids is key in grasping how organisms function. This guide aims to simplify the complex nuances of mRNA to amino acid mapping, revealing not only its scientific underpinnings but also its broader implications in various fields.
Research Highlights
In the realm of molecular biology, mRNA acts as an intermediary between the genetic material in DNA and the actual building blocks of life—amino acids. Through a process called translation, ribosomes read the sequence of mRNA and produce proteins by linking together specific amino acids in the correct order.
Key Findings
- The genetic code comprises a series of three-nucleotide sequences called codons, each corresponding to a specific amino acid.
- Starting from the initiation codon (AUG), the ribosome translates mRNA into an amino acid chain, which then folds into functional proteins.
- The mRNA to amino acid mapping is not one-dimensional; it is influenced by various factors such as tRNA availability and ribosomal efficiency.
Implications and Applications
The implications of understanding mRNA and its conversion to amino acids extend beyond the laboratory walls. In the field of medicine, mRNA technology is a game changer, evident through its pivotal role in the development of vaccines. This inspires further research into genetic therapies where manipulating mRNA could potentially treat diseases caused by protein misfolding. Moreover, the agricultural sector could harness this knowledge for genetic modifications that lead to higher yields and pest resistance in crops.
Methodology Overview
To progressively unpack the concept of mRNA and its translation into amino acids, a structured approach is essential.
Research Design
A systematic literature review forms the backbone of our methodology, allowing us to map existing knowledge with recent advancements in mRNA research.
Experimental Procedures
In laboratory settings, researchers typically utilize techniques such as:
- In-vitro translation systems: To observe how mRNA translates into proteins without cellular interference.
- Sequencing technologies: These help identify the sequences of mRNA and the resulting amino acids to confirm accuracy.
As we dive deeper into understanding mRNA mapping, the next sections will further elucidate codon functions, the translation process, and the intricate relationship between genetic coding and protein synthesis.
Preface to mRNA
Messenger RNA, or mRNA, plays a crucial role in the complex web of biological systems. It's like the middleman between the instructions encoded in DNA and the actual production of proteins. Understanding this relationship is not just for your average biology student; it’s a topic that resonates throughout all fields of life sciences, biotechnology, and even medicine.
The article aims to provide a comprehensive look at various aspects of mRNA, including its definition, structure, and the operation of translating genetic code into functional proteins. This foundational knowledge is key for grasping how genes dictate the behavior of living organisms, the essence of life itself.
By detailing these aspects, we can appreciate mRNA's role in and significance to genetic coding. It helps bridge the gap between theoretical knowledge and practical applications. Knowing the ins and outs of mRNA equips researchers and practitioners to employ it effectively, be it in gene therapy, vaccine development, or other biotechnological innovations.
Definition and Function
At its core, mRNA serves as a messenger, carrying genetic information from DNA in the nucleus to the ribosomes in the cytoplasm, where proteins are synthesized. Essentially, it’s the transitory form of genetic information that directs the making of proteins, the workhorses of the cell.
The very act of producing proteins is vital for life. Without mRNA, your cells wouldn’t know how to assemble proteins, leading to a cascade of failures at a biological level. For example, the process starts when DNA is transcribed into mRNA. This mRNA then guides the synthesis of proteins by presenting a sequence of nucleotides that corresponds to specific amino acids. This interplay is what fuels cellular functions and impacts overall organismal health.
Structure of mRNA
Understanding the structure of mRNA can give one insight into how it performs its role. The architecture of mRNA is multi-layered, consisting of the primary, secondary, and tertiary structures.
Primary Structure
The primary structure of mRNA is its linear sequence of nucleotides. Each nucleotide consists of a nitrogenous base, a sugar, and a phosphate group. This sequence directly reflects the genetic information contained within DNA, dictating the amino acid sequence of the resulting protein.
An essential characteristic of the primary structure is that it dictates the genetic code and contributes to the way proteins are synthesized through codons. Each codon, which is a triplet of nucleotides, corresponds to a specific amino acid or a stop signal during translation, making this structural aspect particularly useful in understanding protein synthesis. Its simplicity is its strength; while it appears basic, the effects of mutations in this sequence can have profound consequences in terms of how proteins function, thus playing a pivotal role in cellular processes.
Secondary Structure
The secondary structure refers to the localized folding of the mRNA strand, stabilized by base pairing within the molecule itself. Hairpins, loops, and other formations can emerge, creating regions where the RNA folds back on itself.
The key feature of secondary structure is its potential to influence the stability and translation efficiency of mRNA. For instance, certain structural motifs are recognized by ribosomes, which can enhance translation. However, a complex secondary structure can also impede access to the ribosome, slowing down the translation process. This dual role enhances its importance in genetic expression and regulation.
Tertiary Structure
The tertiary structure is the overall three-dimensional shape of an mRNA molecule. This architecture is crucial; it can determine how the mRNA interacts with proteins and ribosomes during translation. This structure results from various interactions, including hydrogen bonds and hydrophobic effects.
A striking characteristic of the tertiary structure is how it can facilitate specific interactions with ribosomal components or regulatory proteins, enhancing the effectiveness of translation. Ultimately, a well-structured mRNA can lead to more efficient protein production, clarifying its significance in the translation process.
Comparative Analysis
To understand the role of mRNA fully, one must compare it with other nucleic acid types, particularly DNA and transfer RNA (tRNA).
mRNA vs. DNA
Comparing mRNA with DNA sheds light on their different functions. While DNA serves as a stable repository of genetic information, mRNA is more transient, quickly synthesized and degraded as needed. This impermanence allows for greater flexibility in gene expression. One significant feature is that DNA is double-stranded, offering stability, whereas mRNA is single-stranded, making it more adaptable for its role in protein synthesis.
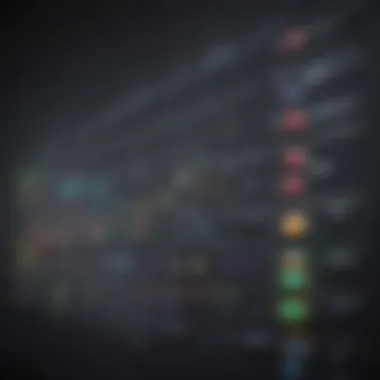
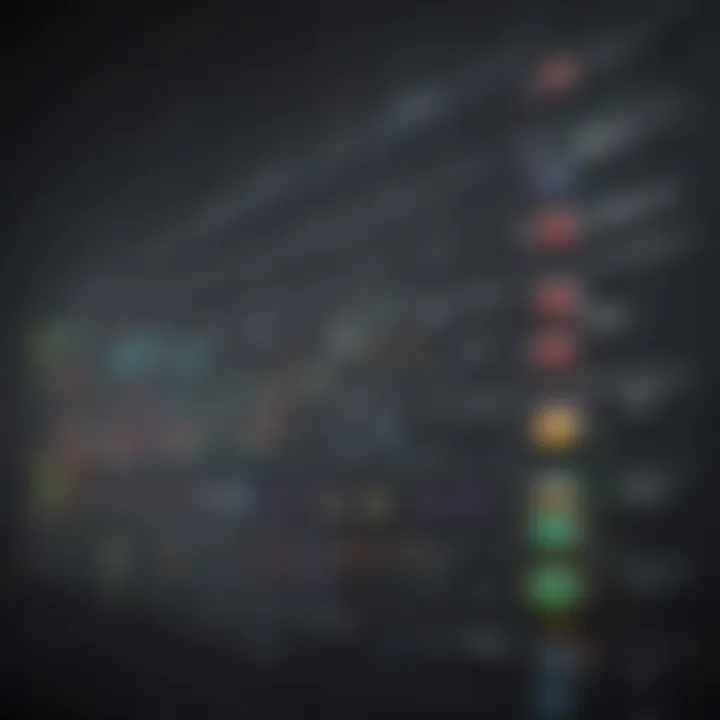
The advantage of mRNA's fleeting nature is that it can readily respond to cellular needs, facilitating rapid changes in protein production as required by environmental conditions.
mRNA vs. tRNA
Whereas mRNA is the template that carries genetic information, tRNA's job is to transport the correct amino acids to the ribosome during protein synthesis. Each tRNA molecule has an anticodon that matches a specific codon on the mRNA strand, ensuring the appropriate amino acids are added to the growing polypeptide chain.
The notable difference is in their functions: mRNA serves as the blueprint, while tRNA plays the role of the delivery service. This complementary relationship is integral to the protein synthesis machinery and showcases the necessity of both molecules in translating genetic information into functional proteins.
The Genetic Code
The genetic code is the cornerstone of molecular biology, describing how sequences of nucleotides in DNA and mRNA translate into amino acids, which are the building blocks of proteins. This code is essential for all forms of life, as it dictates the structure and function of proteins, ultimately influencing everything from metabolism to immune response. Understanding the genetic code opens the door to comprehending how genetic information is expressed and regulated within cells.
Understanding Codons
Definition of Codons
Codons are three-nucleotide sequences found within mRNA that specify which amino acid will be added next during protein synthesis. Each codon corresponds to a particular amino acid or a stop signal in the translation process. A key characteristic of codons is their triplet nature, allowing for a total of 64 possible combinations from the four nucleotides (adenine, cytosine, guanine, and uracil).
This triplet format serves a crucial function in protein coding—it enhances genetic diversity and allows for precise translation of genetic information. The unique feature here is that while there are 20 standard amino acids, the redundancy in codons means that multiple codons can specify the same amino acid, which is a significant benefit as it helps minimize potential errors in protein synthesis.
Types of Codons
In the realm of protein synthesis, codons can be categorized into three types: sense codons, nonsense codons, and initiator codons. Sense codons correspond to amino acids, while nonsense codons signal termination of protein synthesis. Initiator codons specifically flag the start of the translation process.
The presence of various types of codons emphasizes the complexity of gene expression and highlights the importance of codon recognition in the ribosome during translation. This classification aids in understanding mutational effects, as changes in codons can lead to altered amino acid sequences that may affect protein function.
The Codon Table
Standard Codon Table
The standard codon table is a vital reference tool in the field of genetics. It lists out all the codons and their corresponding amino acids, encapsulating the relationship between mRNA sequences and protein output. This table is immensely beneficial because it provides a clear, organized framework that aids researchers when interpreting genetic sequences.
One unique feature is its use in various molecular biology applications, from gene cloning to vaccine design. By referring to this table, scientists can quickly ascertain how changes in DNA sequence might affect protein synthesis, which is essential for designing experiments in genetic research.
Alternative Codon Tables
Not all organisms follow the standard codon table. Alternative codon tables exist and they reflect variations found in some species, particularly among protozoa and mitochondria. The existence of these alternative tables sheds light on the evolutionary adaptations of different life forms and the potential for translational machinery variability.
While these tables provide insights into the flexibility of the genetic code, they can complicate genetic studies. Researchers must be aware of these differences when investigating gene expression and mutation effects, as the implications could lead to varying protein structures and functions across different organisms.
Redundancy of the Genetic Code
The redundancy of the genetic code refers to the phenomenon where multiple codons encode for the same amino acid. This characteristic enhances biological robustness, as it provides a buffer against mutations. Notably, if a single nucleotide is altered, the redundancy might prevent a resulting change in protein function.
Moreover, this redundancy plays an essential role in evolution, allowing organisms to adapt to environmental pressures while maintaining their essential protein synthesis capabilities. A thorough understanding of how redundancy works is crucial for scientists working in genetics and biomedicine, as it affects everything from evolutionary biology to therapeutic strategies in genetic disorders.
"The genetic code is not merely a cellular instruction manual; it's a rich tapestry that reflects the complexity and adaptability of life itself."
Translation Process
The translation process is a pivotal set of mechanisms in cellular biology where messenger RNA (mRNA) is deciphered to synthesize polypeptides, ultimately folding into functional proteins. This section dissects the various stages of translation: initiation, elongation, and termination. Not only is this process a fundamental aspect of gene expression, but it also serves as a backdrop for understanding many medical and biotechnological advancements. Given its relevance, this section combines intricate details with practical implications, ensuring a comprehensive understanding of how mRNA maps to amino acids during protein synthesis.
Initiation
Initiation marks the commencement of translation, pulling together mRNA, ribosomal subunits, and the initial transfer RNA (tRNA) that carries the amino acid. This phase is vital as it sets the stage for the entire translation process, ensuring accurate protein synthesis from the starting point.
Role of Ribosomes
Ribosomes are the factories of protein synthesis and play a critical role in decoding mRNA. They consist of two subunits: a large and a small one, both made of ribosomal RNA (rRNA) and proteins. One remarkable aspect is their ability to facilitate the alignment of mRNA with tRNA, ensuring codons are accurately read. This characteristic makes ribosomes essential in translating genetic information into functional proteins.
- Key Point: Ribosomes move along the mRNA, translating it into a polypeptide chain, allowing for rapid protein assembly.
- Unique Feature: Their dual subunit structure enhances efficiency, as one subunit binds the mRNA, while the other helps position the tRNAs correctly.
While ribosomes are generally a robust solution for translation, potential complications may arise in their functioning under stressful cellular conditions, possibly leading to misfolded proteins.
Initiator tRNA
Initiator tRNA plays a specialized role at the beginning of the translation process. It uniquely carries the amino acid methionine, which always marks the start of protein synthesis. This unique property differentiates initiator tRNA from other tRNAs, which covey other amino acids.
- Key Characteristic: The ability of initiator tRNA to recognize the start codon (AUG) on the mRNA allows for seamless alignment.
- Unique Feature: It interacts directly with the ribosome to position the start of the amino acid chain properly.
One of the advantages of initiator tRNA is that it accelerates the efficiency of the translation process, as it starts the polypeptide synthesis swiftly. Yet, a downside might be that its limited availability could create bottlenecks in specific cellular conditions.
Elongation
Elongation is where the core activity of peptide bond formation occurs, expanding the amino acid chain sequentially according to the mRNA template.
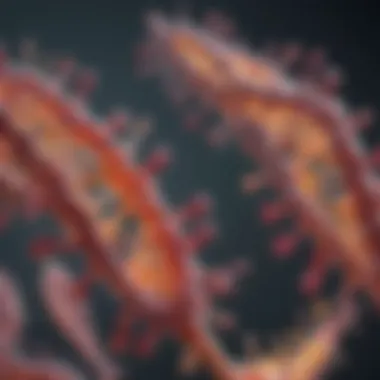
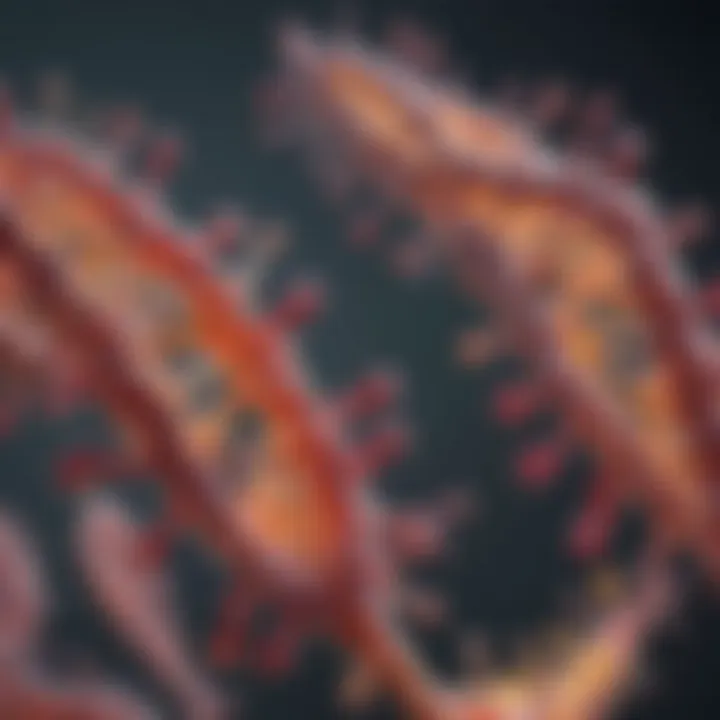
Peptide Bond Formation
During elongation, peptide bonds are formed between adjacent amino acids, establishing a polypeptide chain. This process occurs in the ribosome's A-site (aminoacyl site), where a new tRNA molecule brings its amino acid.
- Key Characteristic: The catalytic activity involved is facilitated by ribosomal RNA, showcasing a remarkable level of RNA functionality in biology.
- Unique Feature: The formation of peptide bonds is highly efficient, and the ribosome ensures only correct base-pairing occurs, reducing errors in protein synthesis.
The efficiency of peptide bond formation enhances the versatility of biological proteins, but potential misalignments during elongation can result in nonfunctional proteins, demonstrating a vulnerability in this robust mechanism.
Amino Acid Sequence Construction
Amino acid sequence construction involves sequentially adding amino acids per the codons on the mRNA strand. This translation of nucleotide sequences into functional structure is what creates the unique characteristics of every protein.
- Key Point: The order of amino acids determines the protein's structure and function, making this stage crucial.
- Unique Feature: The efficiency of this process contributes to diverse protein synthesis, catering to cellular demands.
However, this stage could also face challenges, such as the competition of incorrect tRNAs reaching the ribosome, which may disrupt the fidelity of protein construction, resulting in dysfunctional proteins.
Termination
Termination is the concluding phase of translation, ensuring the synthesis of full proteins and release of the newly formed polypeptide.
Release Factors
Release factors are proteins that play an essential role at the termination stage. They recognize the stop codon on the mRNA, which does not correspond to any tRNA, signaling the end of the translation.
- Key Characteristic: Their ability to bind to the ribosome and facilitate the release of the completed polypeptide chain is critical in the translation process.
- Unique Feature: They effectively disassemble the translation complex, ensuring the ribosomal subunits can be reused in subsequent translation events.
The timely involvement of release factors enhances translation efficiency, but any dysfunction in these factors could lead to premature termination or failure to terminate, potentially obstructing protein synthesis entirely.
Disassembly of the Translation Complex
Once the polypeptide is released, disassembly of the translation complex occurs, which involves separating the ribosomal subunits and releasing the mRNA. This aspect of termination is crucial for preparing the ribosome for the next round of translation.
- Key Point: The rapid disassembly allows the cell to reuse the ribosomal components for new mRNA strands, making the process resource efficient.
- Unique Feature: Efficient disassembly is paramount in maintaining cellular homeostasis, enabling timely response to varying cellular environments.
However, in conditions where disassembly is inefficient, accumulation of translation complexes can lead to cellular stress, impacting overall protein homeostasis.
In summary, the translation process serves not just as a mechanism for protein synthesis, but rather as a cornerstone for understanding how genetic information manifests into life's rich tapestry. Each stage—initiation, elongation, termination—holds its intricacies and significance, underlining the sophistication of cellular machinery.
The mRNA to Amino Acid Chart
The mRNA to amino acid chart serves as a foundational tool in molecular biology. It acts as a guide to translate the sequence of nucleotides in mRNA into the sequence of amino acids that form proteins. Understanding this mapping is crucial for anyone delving into genetics, biotechnology, or medicine. There are various aspects to consider when discussing this chart, particularly its structure, uses, and the implications of the genetic code it embodies.
Chart Overview
The chart simplifies the complex relationship between mRNA codons and amino acids. Essentially, each codon in the mRNA corresponds to a specific amino acid or a stop signal in protein synthesis. Generally, this chart is presented as a table, allowing researchers and students to quickly identify which codon codes for which amino acid. This visual representation is an essential part of biological study, enabling clarity in understanding how genes express proteins.
Symbol Representation
Single-Letter Codes
The single-letter code is a concise way to represent amino acids, using just one letter for each. This system provides a quick reference that is especially popular in protein sequences. A key characteristic of single-letter codes is their efficiency in summarizing large sequences of proteins without losing essential information. For instance, the amino acid Methionine is represented by the letter 'M.'
One of the unique features of single-letter codes is their widespread acceptance in bioinformatics and protein databases, making them a preferred choice among scientists. However, while they are practical, it can sometimes lead to confusion for those new to the field, as it may require memorization of significant associations.
Three-Letter Codes
On the other hand, the three-letter code is a more descriptive format, providing a clearer view of the amino acid names, like 'Met' for Methionine. This representation is particularly useful for educational purposes as it offers a more intuitive understanding and minimizes ambiguity. A notable benefit of the three-letter system is its ease of use when teaching foundational concepts; students can link the code directly to the amino acid name, which aids retention.
However, the three-letter codes can take up more space, which may not be as efficient for displaying large protein sequences. Each system has its strengths, and knowing when to use each is part of becoming proficient in this field.
Practical Applications
Biotechnology
In biotechnology, the mRNA to amino acid chart has immense relevance, particularly in genetically engineering organisms to produce desired proteins. The ability to accurately predict and manipulate protein synthesis allows scientists to innovate in areas like gene editing and synthetic biology. The chart acts as a reference point, ensuring that modifications lead to the intended outcomes at the protein level.
A distinctive feature of biotechnology applications is the ability to use the chart to design synthetic genes that yield proteins for therapeutic use. While this is a groundbreaking advancement, it also comes with complexities regarding off-target effects and unpredicted results during protein folding, thus necessitating precise mapping against the chart.
Pharmaceutical Development
When it comes to pharmaceutical development, understanding the mapping from mRNA to amino acids is crucial for drug design. This knowledge enables researchers to develop medications that can selectively target specific proteins or enzymes implicated in diseases. For example, using mRNA-based therapeutics, researchers have begun exploring how these mappings can inform the development of vaccines.
Moreover, this mapping provides insights into how modifications to amino acids can enhance drug efficacy or stability. However, the developing phase can face challenges, particularly in ensuring safety and efficacy, establishing a rigorous testing process to validate the implications presented by the chart.
"The mRNA to amino acid chart is more than just a table; it's a roadmap guiding us through the complex landscape of genetic expression and protein synthesis."
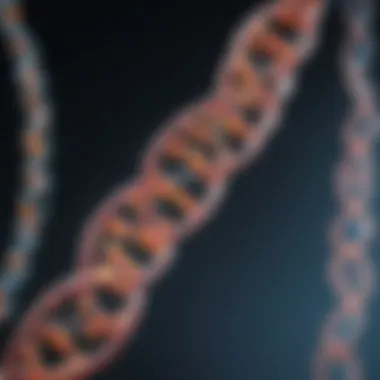
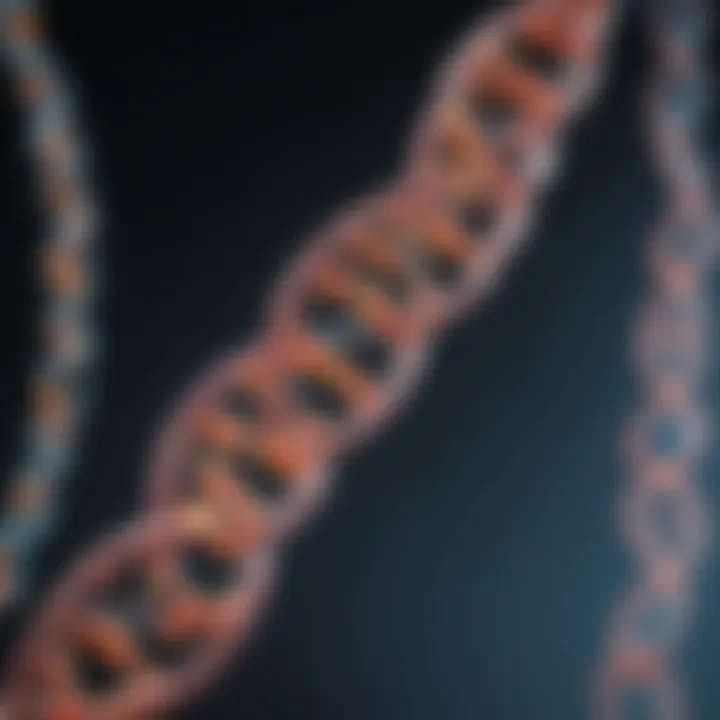
The significance of the mRNA to amino acid chart goes beyond mere academic interest. It’s a pivotal element in numerous scientific fields, linking the understanding of genetic information to practical applications that can affect our world.
Significance of the mRNA to Amino Acid Chart in Science
The mRNA to amino acid chart is not just a simple chart; it holds monumental significance in our understanding of life at a molecular level. This mapping of genetic information directs how proteins are synthesized, which, in turn, are essential for every biological process. By analyzing this chart, scientists gain insights into protein structures, functions, and the very coding language that underpins life.
Impact on Understanding Biology
When we consider the enormous complexity of biological systems, the mRNA to amino acid chart serves as a foundational element in molecular biology. Each codon, a sequence of three nucleotides in mRNA, corresponds to a specific amino acid. This relationship is fundamental for translating genetic information into functional proteins. Understanding this mapping not only clarifies how genes dictate biological traits but also enhances our grasp of how mutations can lead to diseases.
In essence, this chart is a bridge. It connects the abstract realm of genetic code with the tangible world of proteins, which carry out the cellular tasks needed for life. By comprehensively analyzing the mRNA to amino acid chart, students and researchers can address deeper questions about genetic regulation and evolution.
Role in Genetic Engineering
In the ever-evolving landscape of genetic engineering, the mRNA to amino acid chart is invaluable. As scientists explore gene editing techniques like CRISPR, understanding how mRNA sequences translate into proteins allows for precise modifications of genetic material. For instance, by altering a codon, scientists can produce variants of a protein with enhanced properties or reduced side effects.
Moreover, this chart helps in designing synthetic biology applications. Researchers can predict how changes in the mRNA sequence will affect the resulting amino acid sequence and, ultimately, the function of the resultant protein. This predictive power is a game-changer in fields such as biomanufacturing and personalized medicine, enhancing our capacity to develop tailored therapies for patients.
Applications in Medicine
Gene Therapy
Gene therapy represents a novel approach to treating diseases at the genetic level. Central to this process is the mRNA to amino acid mapping. By delivering functional copies of genes into patients, or modifying existing ones, this technique aims to correct genetic disorders at their source. A key characteristic of gene therapy is its targeted approach; instead of merely addressing symptoms, it confronts the root cause of diseases.
One unique feature of gene therapy is its versatility. It can be used for a range of conditions, from inherited disorders to certain types of cancer. The advantages are significant: the potential for long-term cures and less reliance on traditional medications. However, challenges such as immune responses or unintended mutations remain concerns that researchers are diligently working to overcome. The mRNA to amino acid chart remains a cornerstone in refining these therapeutic strategies.
Vaccination Technologies
Vaccination technologies have been revolutionized by the advent of mRNA vaccine platforms. These vaccines instruct cells to produce a protein that resembles part of a virus, prompting an immune response. The direct translation of mRNA sequences into functional proteins exemplifies the critical role of the mRNA to amino acid chart in modern medicine.
A pivotal aspect of vaccination technologies is their rapid adaptability. If a new viral variant emerges, the mRNA can be quickly adjusted to address the new threat. This adaptability is crucial for public health responses, especially during pandemics. The distinctive feature of mRNA vaccines is their ability to provoke strong immune reactions without using live pathogens, making them a safe choice.
However, challenges such as cold-chain storage and public hesitance remain barriers to widespread acceptance and application. Understanding the mRNA to amino acid chart not only aids in the design of these vaccines but also in educating the public about their safety and efficacy.
In summary, the mRNA to amino acid chart is not just a reference; it is a key to unlocking the mysteries of biology, advancing genetic engineering, and transforming medicine.
Future Prospects of mRNA Research
The sphere of mRNA research is evolving at a remarkable pace, holding great potential for a multitude of therapeutic applications. As scientists delve deeper into understanding the mechanisms underlying mRNA interactions and functions, several key trends and challenges have emerged, shaping the future landscape of biotechnology.
Trends in Therapeutic Development
One of the most telling trends in mRNA research is its upward trajectory in therapeutic development. Just look at how mRNA vaccines for COVID-19 have changed the game. This innovative approach has not only underscored the feasibility of mRNA as a platform but also highlighted its speed in enabling rapid responses to emerging infectious diseases.
Additionally, researchers are increasingly exploring the use of mRNA in cancers, where it could instruct the body to produce proteins that stimulate a robust immune response against tumors. Another area of interest is in rare genetic disorders, where customized mRNA can be used to deliver the correct proteins that the body lacks. This personalized medicine approach illustrates how mRNA materials could lead to tailor-made treatments. The promise of mRNA therapies lies not only in their ability to treat existing conditions but also in their preventive potential, such as developing vaccines targeting a myriad of pathogens.
Challenges Facing mRNA Technology
While there is significant excitement surrounding mRNA technology, challenges loom large on the horizon.
Stability Issues
Stability issues refer to the fragility of mRNA molecules outside of the controlled environment of a laboratory. These molecules can be quite sensitive to heat and degradation, which brings complications for storage and transportation. The key characteristic of this instability lies in the inherent nature of RNA, which can easily be broken down by nucleases—enzymes that cleave RNA strands.
This aspect is crucial in discussions about mRNA vaccines, as it affects their shelf life and usability. A beneficial choice for the future could include advancements in encapsulation techniques, which have shown promise in improving the stability of mRNA, allowing for greater accessibility and wider use. Unique compounds and modifications like lipid nanoparticles can enhance mRNA’s resistance to degradation, thus providing necessary support for its application in therapeutic settings.
Delivery Mechanisms
Delivery mechanisms play a pivotal role in ensuring that mRNA reaches its target within the body's cells without being prematurely degraded. The key characteristic of these systems lies in their ability to protect mRNA while facilitating its entry into cells. This is particularly important, considering the fact that mRNA has difficulty crossing cellular membranes due to its size and negative charge.
Innovative delivery methods, such as lipid nanoparticles, are gaining traction. These have demonstrated effectiveness in transporting mRNA into human cells, safeguarding the mRNA during transit. However, achieving effective delivery poses unique challenges. The delivery vehicles must not only be efficient but also biocompatible, minimizing toxicity while maximizing biodistribution. As research continues, finding the sweet spot between delivery efficiency and safety will remain a key focus area.
Ethical Considerations
As mRNA technology progresses, ethical considerations become increasingly relevant. Questions around genetic privacy, informed consent, and potential misuse of mRNA technologies in genetic modification must be addressed. Transparency in the research and development phases will be critical in fostering public trust and ensuring ethical compliance in mRNA applications, especially as they cross into therapeutic realms with implications for gene editing and personalized medicine.
"The ongoing dialogue about ethics must keep pace with technological advancements to avoid pitfalls and ensure responsible use of mRNA technologies."
In summary, while the future of mRNA research appears promising, navigating through various challenges and ethical landscapes is essential for unlocking its full potential. As we forge ahead, a balanced approach that emphasizes innovation alongside ethical responsibility will dictate the trajectory of mRNA in health sciences.
Closure
The study of the relationship between mRNA and amino acids holds great importance in the contemporary scientific landscape. This article emphasizes how understanding mRNA to amino acid mapping can illuminate our grasp of fundamental biological processes. By unpacking complex terminologies and mapping out relevant concepts, readers can connect theoretical knowledge with practical applications.
Summary of Key Points
In reviewing the content presented in this article, several key points emerge:
- mRNA Structure: The intricate architecture of messenger RNA underpins its function in carrying genetic information.
- Genetic Code: Codons are the backbone of protein synthesis, translating nucleotides into amino acids efficiently.
- Translation Process: The stages of initiation, elongation, and termination outline the journey of mRNA as it transforms into a protein.
- mRNA to Amino Acid Chart: This chart serves as a crucial reference, simplifying the conversion process.
- Future Prospects: Ongoing research into mRNA technology underlines its potential in healthcare and biotechnology.
Final Thoughts on mRNA Significance
As we reflect on the significance of mRNA, we recognize its role as a cornerstone in genetic expression. The applications of mRNA research touch on numerous fields such as genetics, medicine, and biotechnology. mRNA serves not only as a messenger but also as a vital player in developing therapeutics and vaccines, proving its relevance in modern science.