The Role of Physicist Engineers in Modern Innovation
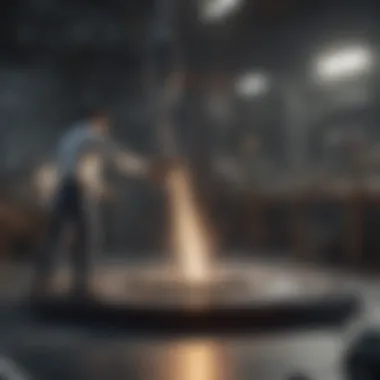
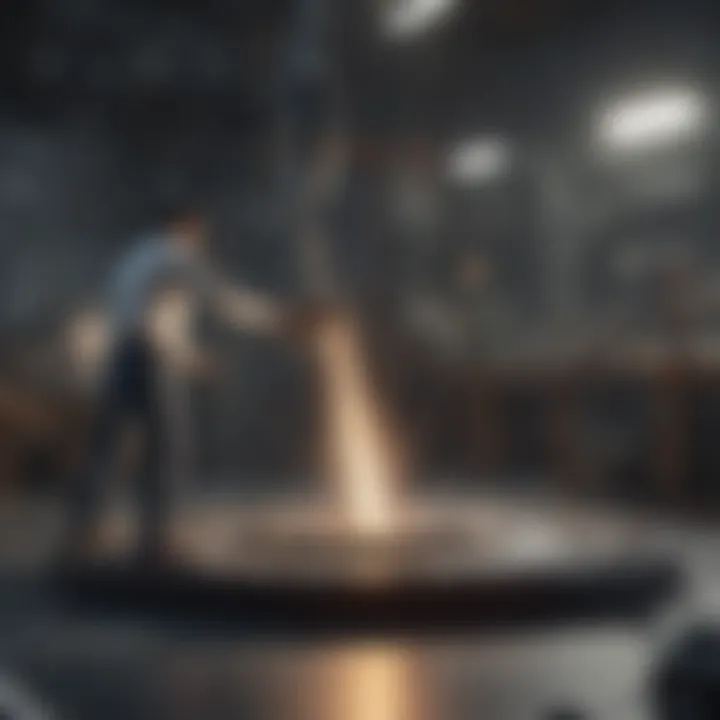
Intro
The integration of physics and engineering has long been essential in addressing complex problems and developing innovative solutions. The career of a physicist engineer exemplifies this merge, where one employs core principles of physics in practical engineering projects. This article seeks to explore the vital role of physicist engineers, laying bare the educational paths they typically traverse, the skills they acquire, and the challenges they confront in their professions.
By examining pioneering projects in the field, the article will elucidate how these professionals impact technological advancement and societal wellbeing. In doing so, it rallies against the often-overlooked significance of their contributions to the scientific world and everyday life.
Research Highlights
Key Findings
Physicist engineers possess a unique skill set, blending theoretical knowledge of physics with practical engineering expertise. They are involved in diverse areas such as renewable energy, materials science, and advanced manufacturing technologies. Their ability to think critically and apply scientific principles directly leads to groundbreaking innovations.
Implications and Applications
Understanding the role of physicist engineers provides insights into contemporary technological challenges. For instance, the growth of renewable energy sources, such as solar and wind power, relies heavily on expertise that physicist engineers provide. Their familiarity with the advanced principles of physics makes them critical in optimizing the efficiency of energy systems. Likewise, in fields like nanotechnology, physicist engineers serve as a bridge connecting theoretical developments with tangible applications in electronics and materials.
"The contributions of physicist engineers are essential for sustainable technological growth and solving today's pressing challenges."
Methodology Overview
Research Design
The study highlights the educational framework necessary for aspiring physicist engineers. Typically, this includes a rigorous combination of physics and engineering courses, fostering a multidisciplinary understanding needed for effective problem-solving in real-world contexts.
Experimental Procedures
Real-world projects featuring physicist engineers illustrate their role in innovation. Collaborative works in laboratories and industries embody the experimental spirit of this profession, leading to advancements across various engineering fields. Examples of successful projects can yield insights into effective practices and methodologies that other physicist engineers may adopt.
By connecting academic knowledge to hands-on experience, these professionals push the boundaries of conventional engineering, providing a robust framework for future developments.
Prologue to Physicist Engineering
The fusion of physics and engineering takes center stage in understanding the role of the physicist engineer. This profession not only requires advanced knowledge of physical laws but also the ability to apply these principles in practical contexts. The significance of this intersection cannot be underestimated. Both fields complement each other remarkably, resulting in innovations that propel technology forward.
Physicist engineers bridge theoretical concepts with engineering applications. In the modern world, challenges such as climate change, energy sustainability, and healthcare innovation demand an integrated approach. By utilizing the analytical tools of physics, these professionals can design efficient systems, develop new materials, and create technologies that enhance quality of life.
Defining Physicist Engineering
Physicist engineering involves more than just the application of scientific principles; it requires a deep understanding of both physics and engineering methodologies. A physicist engineer is equipped with skills in analysis, design, and technical implementation, allowing them to address complex problems across various industries. The role often entails tasks such as modeling physical systems, conducting experiments, and collaborating with interdisciplinary teams.
The core of this discipline lies in its ability to translate abstract physical theories into tangible engineering designs. This is critical in areas such as semiconductor technology, renewable energy systems, and materials science. Therefore, a concise definition would be: physicist engineering is the practice of integrating physics concepts into engineering solutions.
Historical Context
Historically, the fields of physics and engineering evolved separately, though they have been interconnected from early scientific advancements. The Industrial Revolution marked a turning point, as engineers began to apply physical principles more rigorously to increasing productivity in manufacturing. Significant contributions from physicists like James Clerk Maxwell and Albert Einstein laid foundational theories that engineers would later employ in practical applications.
The 20th century saw further integration of these disciplines in response to technological advancements such as computers and telecommunications. Notably, physicist engineers played pivotal roles in the development of innovative technologies such as lasers and nuclear energy. The advancements in materials science can also be traced back to collaborative efforts between physicists and engineers.
Understanding the historical context of physicist engineering is crucial for recognizing its current relevance. As industries and society face new challenges, the historical collaborations serve as a model for future efforts in innovation and problem-solving.
Educational Pathways for Aspiring Physicist Engineers
The convergence of physics and engineering presents unique educational pathways for students who wish to become physicist engineers. This intersection is critical because it fosters the skill set necessary to address complex engineering problems while applying the principles of physics. Understanding these pathways can help aspiring professionals navigate their educational journeys effectively.
Typical Degree Programs
A solid foundation in both physics and engineering is essential. Most physicist engineers pursue a degree in either physics or engineering, often followed by a master's or doctoral level education. Common undergraduate programs include:
- Bachelor of Science in Physics: This program offers robust theoretical knowledge and experimental techniques in physics. It prepares students for advanced studies in both applied and theoretical fields.
- Bachelor of Science in Engineering Physics: This program combines engineering principles with advanced physics, providing a well-rounded education that is applicable in various industries.
- Bachelor of Engineering in Mechanical, Electrical, or Civil Engineering: These traditional engineering degrees allow students to specialize but also provide opportunities to focus on the physics aspects of their chosen field.
Advanced degrees often focus on specific areas such as materials science, quantum mechanics, or applied physics, which are highly relevant in contemporary engineering problems. A graduate degree can significantly enhance knowledge and improve job prospects in specialized fields.
Interdisciplinary Studies

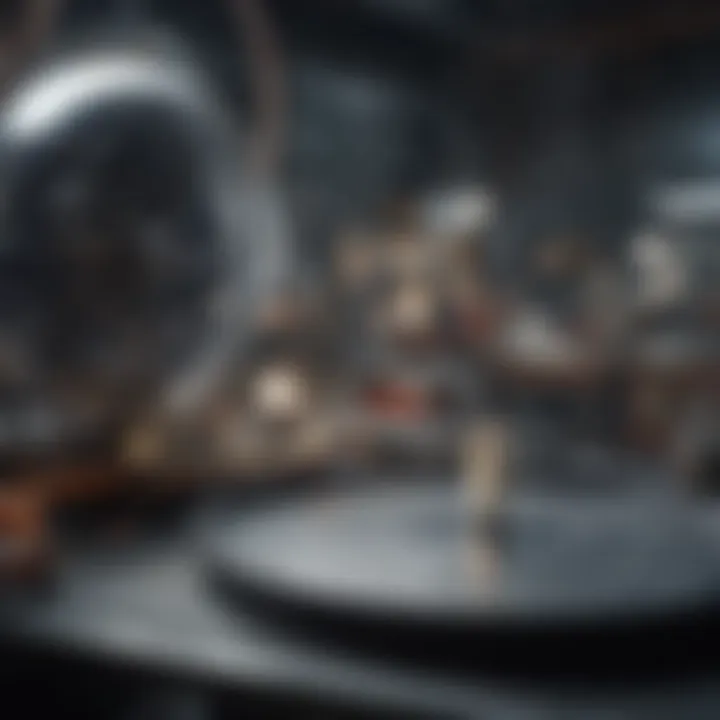
Interdisciplinary studies are vital for physicist engineers, as they engage with concepts from various disciplines. Collaboration with computer science, mathematics, and environmental science can deepen understanding and broaden skills. For instance, integrating software engineering with traditional physics knowledge allows for greater innovation in the development of simulations and modeling.
Students benefit by gaining:
- A diverse skill set that is attractive to employers.
- The ability to tackle complex problems in innovative ways.
- Enhanced teamwork capabilities through collaboration on multidisciplinary projects.
Programs that encourage co-op experiences and interdisciplinary projects create valuable contexts for students to apply their learning in real-world scenarios.
Importance of Research Experience
Research experience is a cornerstone of education for physicist engineers. Engaging in research activities allows students to apply theoretical knowledge in practical ways. It promotes critical thinking, innovation, and problem-solving skills. Participating in research also helps students build relationships with faculty and industry professionals, which can be crucial for future job opportunities.
Students should seek:
- Internships or co-op positions in relevant fields that demonstrate research capabilities.
- Opportunities to assist in faculty-led projects or seek independent research initiatives.
- Participation in conferences to present findings and network with professionals in the field.
Research not only enriches a student's perspective but also lays a strong foundation for future work in industries ranging from aerospace to renewable energy.
"The role of the physicist engineer is not just solving problems but also innovating new solutions that can change entire industries."
This holistic approach to education presents a thorough preparation for aspiring physicist engineers, equipping them with a multifaceted understanding essential for success in both the engineering and physics realms.
Core Competencies of Physicist Engineers
In the specialized field of physicist engineering, core competencies are essential for effective practice. These competencies provide a framework for the skills and knowledge necessary to integrate principles of physics with engineering solutions. Understanding these core competencies equips developing physicist engineers to tackle complex engineering challenges and contribute to innovative projects. Moreover, it emphasizes the necessity of a multidisciplinary approach in contemporary technological environments.
Analytical Thinking
Analytical thinking is at the heart of a physicist engineer's toolkit. This skill encompasses the ability to dissect complex problems into manageable parts, enabling a structured approach to finding solutions. Physicist engineers utilize this skill to formulate hypotheses, analyze data, and evaluate outcomes critically. Through analytical thinking, they can understand the underlying physics involved in engineering challenges, which leads to more effective and efficient designs.
The ability to analyze encompasses various dimensions, including:
- Data Interpretation: Physicist engineers must be adept in interpreting experimental results and simulations. This goes beyond basic calculations; it involves understanding the implications of data and how they fit within broader experimental contexts.
- Modeling: Creating and refining mathematical models that represent physical phenomena is a critical part of their work. Analytical thinking aids in testing these models against real-world data to ensure accuracy.
- Decision Making: By analyzing all available information, physicist engineers can make informed choices that balance theoretical principles with practical realities.
Problem Solving Skills
Problem solving is another indispensable competency in physicist engineering. This skill requires not only the identification of problems but also the creativity to propose viable solutions. The physicist engineer often finds themselves in situations where conventional methods may not suffice, thus necessitating innovative thinking and adaptability.
Key facets of problem solving include:
- Creative Solutions: Physicist engineers must think outside the box, often developing new technologies or modifying existing ones to meet specific requirements.
- Collaborative Efforts: Many engineering challenges require teamwork. Effective collaboration ensures diverse perspectives are considered, which often leads to more robust solutions.
- Iterative Process: Problem solving is rarely linear. It often involves cycles of trial, error, and refinement. Physicist engineers must be comfortable revisiting problems and adjusting their strategies as needed.
"Effective problem-solving is crucial in physicist engineering, where real-world applications often defy simple theoretical solutions."
Technical Proficiency
Technical proficiency is fundamental in achieving success in physicist engineering. This encompasses a blend of both theoretical knowledge and practical skills. It demands a thorough understanding of physics principles, as well as the tools and technologies commonly used in the field.
Components of technical proficiency include:
- Software and Simulation Tools: Proficiency in software like MATLAB or COMSOL Multiphysics is crucial for modeling and simulating physical systems. This allows physicist engineers to predict outcomes and refine their designs before implementing them in practice.
- Hands-On Skills: Experience with laboratory equipment, prototyping tools, and manufacturing processes is essential. This hands-on experience ensures that physicist engineers can bridge the gap between concepts and actual devices or systems.
- Staying Updated: The technical landscape is ever-evolving. Physicist engineers must engage in continuous learning to stay abreast of emerging technologies and methodologies.
In summary, the core competencies of analytical thinking, problem solving, and technical proficiency create a solid foundation for physicist engineers. Together, they empower these professionals to navigate the complexities of modern engineering challenges effectively.
Current Trends in Physicist Engineering
The domain of physicist engineering is experiencing rapid evolution due to various advancements in technology and shifts in societal needs. This has turned the focus towards integrating innovative practices for sustainable solutions. Thus, understanding current trends becomes crucial for stakeholders such as students, educators, and professionals. It serves as a compass for aspiring physicist engineers, helping them navigate their career choices while addressing global challenges effectively.
Innovation and Technology Integration
Innovation in physicist engineering presents a unique opportunity for the amalgamation of theoretical physics and practical application. Innovations often arise from the need to solve complex problems in real-world contexts. For instance, integrating quantum computing with traditional engineering practices is becoming a focal point. Quantum technologies enhance computational abilities, leading to advanced solutions in various fields, including materials science and cryptography.
Moreover, machine learning and artificial intelligence are significantly influencing engineering practices. The application of these technologies allows physicist engineers to optimize existing systems and develop predictive models. This can lead to improved efficiency in sectors like energy, aerospace, and telecommunications. As engineers embrace these sophisticated technologies, they must remain well-versed in the underlying physics principles to leverage their full potential.
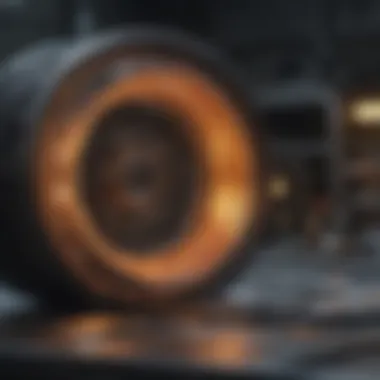
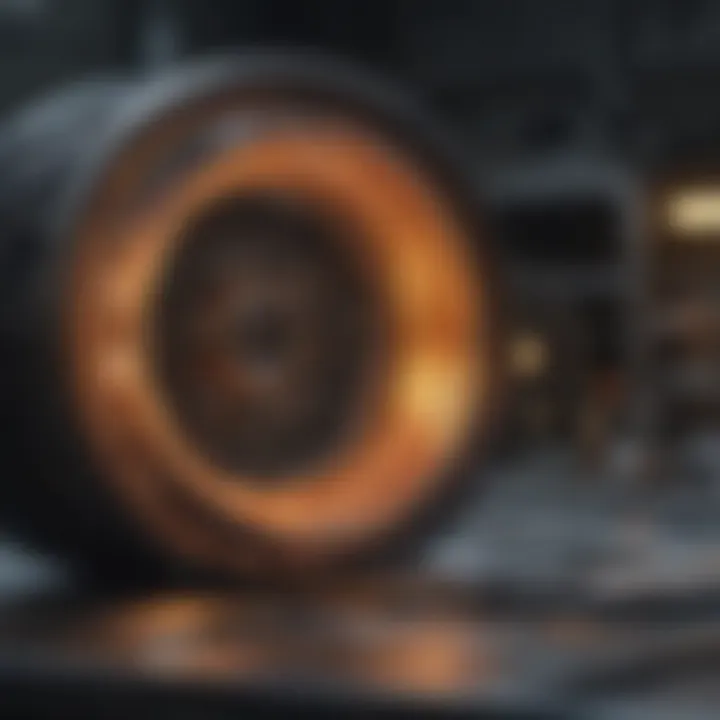
"Innovation is the key to sustainable growth and efficiency. Physicist engineers must be at the forefront of this change."
Key benefits of innovation and technology integration include:
- Enhanced problem-solving capabilities.
- Increased efficiency in processes.
- Improved accuracy in predictions and outcomes.
Sustainable Engineering Practices
As global awareness of environmental issues rises, sustainable engineering practices have become a priority for physicist engineers. This trend emphasizes developing technologies that minimize ecological footprints while advancing industrial objectives. Sustainable practices involve assessing the entire lifecycle of a product or system, promoting the responsible use of resources.
Material science is a critical area where sustainability is gaining ground. The development of new materials, such as biodegradable plastics and energy-efficient composites, highlights the intersection of innovation and sustainability. Additionally, physicist engineers are exploring renewable energy solutions, such as solar photovoltaics and wind turbines, encouraging the shift towards greener alternatives in energy production.
Incorporating sustainability into engineering decisions not only supports environmental goals but also meets growing demands from consumers and regulators for eco-friendly solutions.
Considerations for implementing sustainable practices include:
- Evaluating the lifecycle impacts of materials.
- Focusing on energy efficiency in design.
- Encouraging recycling and reusability in engineering solutions.
Thus, keeping abreast of current trends in innovation and sustainability can empower physicist engineers to excel in their careers and contribute meaningfully to broader societal goals.
Real-World Applications of Physicist Engineering
Physicist engineering serves as a nexus between the theoretical constructs of physics and practical implementations in various industries. The application of physicist engineering can be observed in numerous fields, bridging the gap between abstract ideas and tangible technology. This section highlights critical areas where the role of physicist engineers manifests prominently, illustrating their contributions and the significance of their expertise.
Industrial Applications
Physicist engineers play an essential role in industrial applications, particularly in areas such as manufacturing, energy production, and materials development. These professionals use principles of physics to optimize processes and create efficiencies. For example, in the manufacturing sector,
- Material science: Physicist engineers contribute to designing materials that are strong, lightweight, and cost-effective. This knowledge advances industries ranging from automotive to aerospace.
- Process optimization: They analyze systems to improve production yield while reducing waste. Techniques like Six Sigma often incorporate their insights, leading to significant cost savings for companies.
- Energy: Applications in renewable energy systems, such as solar panels and wind turbines, involve complex physical principles. Physicist engineers design, test, and implement solutions that enhance energy efficiency and sustainability.
Research and Development
The role of physicist engineers in research and development is pivotal for technological advancement. This field requires a blend of creativity and analytical skills, making it a suitable area for physicist engineers. Their work often includes:
- Innovative projects: These professionals direct research efforts on advanced materials and technologies. This can involve simulations and modeling of physical systems to predict behaviors under varied conditions.
- Interdisciplinary collaboration: They frequently collaborate with engineers from other disciplines, leveraging physics to address challenges in new product development. Such collaborations allow for innovative solutions to emerge that might not be possible within a single discipline.
- Funding and grants: They are pivotal in securing financial resources for high-impact research initiatives. Their understanding of scientific principles allows them to articulate project importance to potential funders effectively.
Contributions to Healthcare Technology
In healthcare technology, physicist engineers significantly contribute to diagnostic tools and treatment methodologies. Their contributions include:
- Medical imaging: Imaging techniques like MRI and CT scans rely heavily on principles of physics for their design and operation. Physicist engineers ensure these technologies yield high-quality images while optimizing safety and efficiency.
- Radiation therapy: They utilize their understanding of physics to improve radiation therapies for cancer treatment, helping to minimize exposure to healthy tissues while maximizing the impact on tumors.
- Device innovation: Developing new biomedical devices requires insights from physics. Physicist engineers work on the design of implantable devices and diagnostic instruments, ensuring they function effectively in a biological environment.
"The blending of physics with engineering not only advances technology but also enhances the quality of life through innovative healthcare solutions."
Challenges Faced by Physicist Engineers
The role of physicist engineers encompasses a wide array of responsibilities that bridge the gap between theoretical physics and practical engineering. However, this integration comes with its own set of challenges. In this section, we will explore these obstacles, focusing on two primary areas: interdisciplinary barriers and technological limitations. Understanding these challenges is crucial for physicist engineers to navigate the complexities of their work and contribute toward innovative solutions.
Interdisciplinary Barriers
Physicist engineers operate at the confluence of two distinct fields—physics and engineering. While this position can be advantageous, it also presents interdisciplinary barriers that can hinder collaboration and project success. These barriers often stem from differences in culture, language, and methodology between the two areas.
Key points regarding interdisciplinary barriers include:
- Communication Gaps: Engineers and physicists may use the same terms to describe different concepts. This can create misunderstandings that delay progress on projects.
- Diverging Objectives: Engineers typically focus on practical applications, while physicists may prioritize theoretical outcomes. Aligning these different objectives requires negotiating priorities, which can be time-consuming.
- Educational Backgrounds: Training programs emphasize different aspects of each discipline. This can lead to discrepancies in knowledge and skills, further complicating teamwork.
Physicist engineers need to develop strong communication skills and foster collaboration between disciplines to overcome these barriers. Encouraging an environment of shared learning can also enhance mutual understanding and efficiency.
Technological Limitations
Another critical challenge faced by physicist engineers is navigating technological limitations. Despite advancements in technology, certain constraints can impede the effective application of physics in engineering contexts. Understanding these limitations is imperative for fostering innovation and finding solutions.
Main factors contributing to technological limitations include:
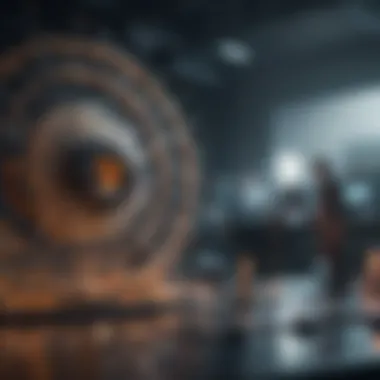
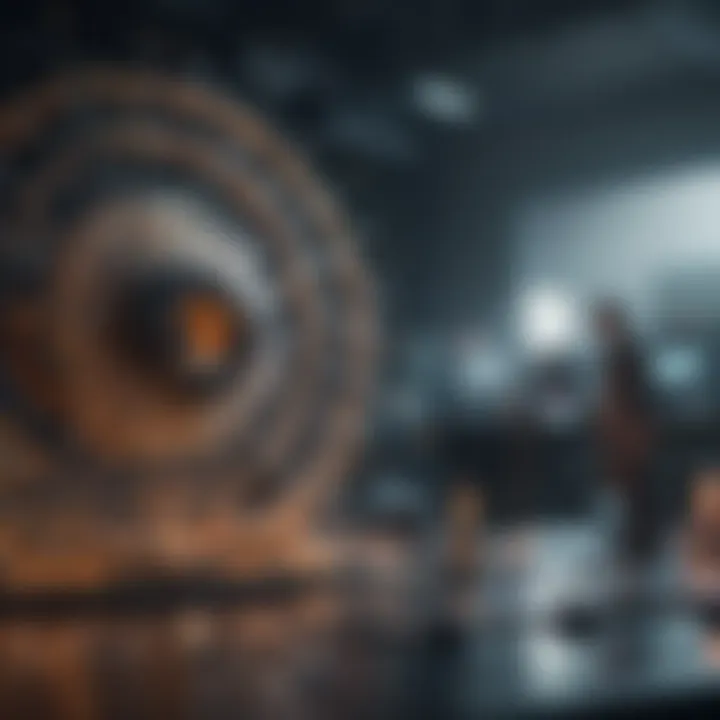
- Resource Availability: Access to advanced materials or technologies can influence project outcomes. Restrictions in material science can limit experimental options for physicist engineers.
- Rapid Technological Change: The fast pace of technological innovation means that skills and knowledge can quickly become outdated, necessitating continuous education. Physicist engineers must stay abreast of new developments to remain effective.
- Integration with Existing Systems: Implementing new technologies often requires harmonizing with established systems, which can be logistically challenging and costly.
To counteract these limitations, physicist engineers should engage in ongoing professional development and actively participate in collaborative projects. Fostering partnerships with technologists and researchers can also provide access to cutting-edge tools and methodologies.
In summary, the challenges faced by physicist engineers demand a proactive approach to foster collaboration and innovation. Overcoming interdisciplinary barriers and technological limitations is essential for the continued advancement of the field.
Pioneers in Physicist Engineering
The field of physicist engineering owes a great deal of its progress to the individuals who were instrumental in shaping its foundations. These pioneers not only advanced theoretical knowledge but also successfully translated complex scientific principles into practical engineering solutions. Their contributions have spanned various domains, from energy systems to medical devices, illustrating the vital intersection of physics and engineering. By highlighting their achievements, we can better understand the significance of their roles and the ongoing impact they have on both scientific and engineering disciplines.
Notable Figures
Several key figures stand out in the annals of physicist engineering. Names like Nikola Tesla, Robert Oppenheimer, and Marie Curie resonate due to their groundbreaking work that has paved the way for modern engineering practices.
- Nikola Tesla: His innovations in electrical engineering and electromagnetism served as the basis for modern power systems. Tesla’s vision for alternating current transformed how energy is transmitted.
- Robert Oppenheimer: Known for his leadership during the Manhattan Project, Oppenheimer's work emphasized the importance of combining physics with engineering to achieve monumental feats within tight timelines.
- Marie Curie: Her pioneering research on radioactivity has been instrumental in the development of nuclear engineering and its applications in medical technology.
These figures are representative of a broader group of individuals who have made essential contributions to the domain. Their stories often reflect the perseverance and dedication needed to overcome challenges in interdisciplinary fields.
Impact on the Field
The influence of these pioneers is multifaceted. By linking theoretical physics with practical engineering applications, they have opened pathways for future innovation and collaboration. Key impacts include:
- Technological Advancements: The groundwork laid by these pioneers has led to significant technological breakthroughs. For example, Tesla's work on wireless transmission continues to affect telecommunications profoundly.
- Interdisciplinary Collaboration: Their achievements demonstrate the necessity of cross-disciplinary approaches. Engineers and physicists often need to collaborate to solve complex problems, resulting in more comprehensive solutions that neither discipline could achieve independently.
- Educational Foundations: The legacy of these pioneers is evident in educational curricula. Many universities emphasize a combined approach to physics and engineering, inspired by the successes of these figures. This encourages new generations of scientists and engineers to think broadly and integratively.
"The greatest technological innovations are often born at the intersection of fields, where collaboration breeds creativity and problem-solving."
In summary, the pioneers of physicist engineering heralded a new era of understanding and innovation. Their contributions laid a robust framework within which future engineers and scientists can operate. Acknowledging their impact not only honors their legacies but also inspires ongoing exploration in the field.
Future Prospects for Physicist Engineers
The field of physicist engineering, as it evolves, holds substantial promise in shaping future technologies. As reliance on scientific principles for engineering solutions increases, the integration of physics will be vital in addressing complex challenges. This importance stems from the interdisciplinary nature of physicist engineering, allowing professionals to bridge the gap between pure science and practical applications.
Evolving Technologies
Evolving technologies represent a significant dimension of future prospects for physicist engineers. Innovations in renewable energy, quantum computing, and nanotechnology showcase how physicist engineers are at the forefront of design and implementation. For instance, renewable energy systems require a firm understanding of physical laws governing energy transfer and efficiency. By harnessing these principles, physicist engineers can create more sustainable solutions to combat climate change. Furthermore, advancements in quantum technology demand engineers who understand not just the theoretical aspects, but can also create devices that utilize these principles effectively.
The shift towards smart materials and functionality in engineering design points to a need for professionals who can manipulate properties at a microscopic level. This trend will likely expand, offering physicist engineers opportunities to innovate in robotics and smart systems integration. Their role will grow, demanding not only technical skills but also creativity in applying physics to problem-solving across various sectors.
Career Opportunities
The career landscape for physicist engineers is diverse and expanding. They can find roles in different industries, ranging from aerospace engineering to semiconductor manufacturing. Often, organizations are seeking such interdisciplinary talent to enhance their innovation pipelines. For example, companies like IBM are actively recruiting physicist engineers to lead research in quantum computing and semiconductor technologies.
Job titles may include:
- Research Scientist: Engaging in cutting-edge research and development.
- Systems Engineer: Integrating complex systems and technologies.
- Product Development Engineer: Designing innovative products based on scientific principles.
- Energy Analyst: Specializing in sustainable systems.
- Biomedical Engineer: Applying physics concepts to healthcare technologies.
Moreover, physicist engineers increasingly pursue entrepreneurial routes, creating startups focused on emerging technologies. With their unique skill set, these engineers often contribute significantly to innovation in sectors like healthcare and environmental technologies, positioning themselves as essential players in future marketplaces.
"Physicist engineers are not just builders; they are creators of tomorrow's solutions, driven by the pursuit of knowledge and its practical applications."
Overall, the future for physicist engineers is robust, characterized by technological advancement and growing career avenues. Their ability to adapt and innovate will be crucial to addressing both existing and future challenges.* This interdisciplinary role places them at the heart of progress, making physicist engineers indispensable in shaping the future.
The End
The role of physicist engineers has grown increasingly significant in today's world. As the domains of physics and engineering converge, professionals in this field bring unique insights and skills that facilitate innovation and address complex challenges. This article emphasizes the intersection between these two disciplines, revealing the profound impact physicist engineers have on technology and society at large.
Summary of Key Insights
In reviewing the crucial components of physicist engineering, several key insights emerge:
- Interdisciplinary Expertise: Physicist engineers leverage a deep understanding of physical principles and engineering practices. This dual expertise allows them to navigate complex technical challenges effectively.
- Real-World Applications: Their work finds relevance across various sectors, including healthcare, manufacturing, and sustainable energy solutions. These professionals contribute to significant advancements that shape everyday life.
- Recognition of Challenges: The article addresses the obstacles faced by physicist engineers. These include interdisciplinary barriers and limitations in technology development. Overcoming these issues is essential for maximizing their potential impact.
"The integration of physicist engineering is vital for advancing technological frontiers and promoting innovative solutions."
The Importance of Physicist Engineers
Physicist engineers play an essential role in the promotion of technological progress. They combine the foundational principles of physics with engineering design to create efficient and sustainable products. Their contributions are particularly important in areas such as:
- Advancing Nanotechnology: This cutting-edge field benefits from the insightful application of physics in engineering processes, leading to novel materials and applications.
- Enhancing Renewable Energy Solutions: Knowledge in thermodynamics and electromagnetism directly applies to the improvement of solar panels and wind turbines.
- Healthcare Innovations: Physicist engineers work alongside medical professionals to develop advanced imaging technologies, such as MRI and CT scans, ensuring better diagnostics and treatment.