Quantum Sensors: The Future of Precision Measurement
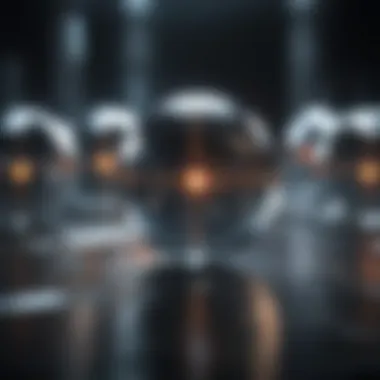
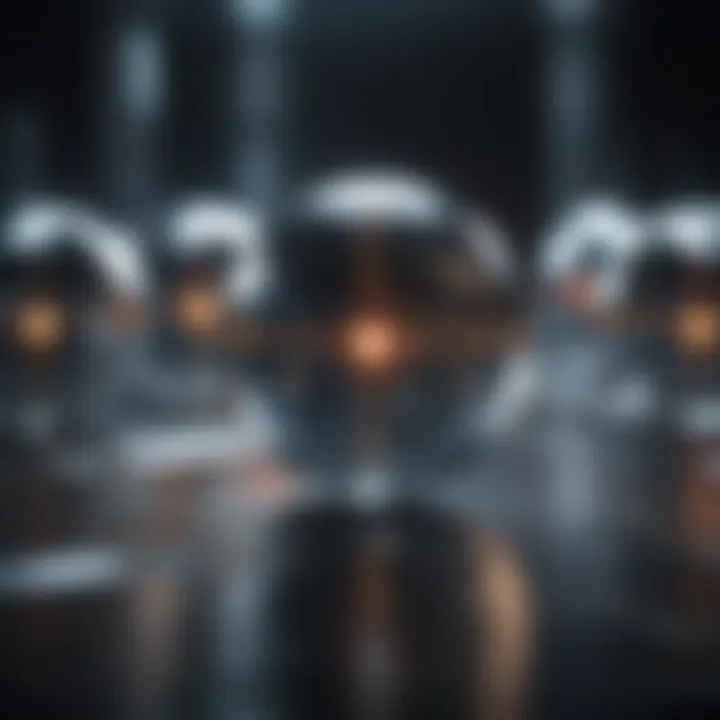
Intro
In the ever-evolving landscape of measurement technology, quantum sensors are starting to stand out like a lighthouse in a stormy sea. These instruments, rooted in the principles of quantum mechanics, are transforming how we gather data across various scientific fields. From detecting minute changes in gravitational forces to measuring the magnetic fields produced by biological systems, quantum sensors offer precision that conventional methods simply can't match.
At the heart of their effectiveness lies the unique behavior of matter at the quantum level. Unlike classical sensors, which are limited by noise and interference in their environment, quantum sensors leverage strange phenomena like superposition and entanglement to enhance their sensitivity. This exploration is not just a theoretical exercise; it has real-world implications that stretch far and wide—from the realm of physics to applications in medical diagnostics and environmental monitoring.
As we embark on the journey through the realm of quantum sensors, we will dissect the key findings that mark their significance and highlight their transformative potential. This examination will include an exploration of the foundational principles of quantum sensing, their various applications across disciplines, and the anticipated advancements that await us in this innovative field.
Prelude to Quantum Sensing
In the ever-evolving field of technology, quantum sensing emerges as a beacon of precision, revolutionizing how various measurements are conducted. The significance of quantum sensing lies in its ability to leverage the principles of quantum mechanics, drastically enhancing sensitivity and accuracy beyond conventional methods. Whether in environmental monitoring, medical diagnostics, or fundamental physics research, the applications are as diverse as they are groundbreaking. Understanding quantum sensing equips researchers and professionals with insights that could reshape industries and scientific paradigms.
Theoretical Foundations of Quantum Mechanics
The bedrock of quantum sensors is firmly anchored in quantum mechanics, a branch of physics that describes the behavior of matter and energy on the smallest scales. At its core, quantum mechanics challenges classical notions, introducing concepts such as superposition and entanglement. These principles enable devices to measure physical quantities with unparalleled finesse. For instance, a quantum sensor can detect incredibly weak signals that would be imperceptible to traditional sensors.
The idea of superposition allows particles to exist in multiple states simultaneously until observed; this characteristic can be harnessed in sensors. Concurrently, entanglement provides a unique correlation between particles, allowing for enhanced measurement capabilities. As such, these theoretical frameworks are not merely academic; they form the scaffolding upon which practical applications of quantum sensing are built.
What is a Quantum Sensor?
A quantum sensor is a device designed to utilize quantum mechanical properties to sense various physical phenomena, usually with heightened precision compared to classical sensors. To put it simply, while conventional sensors may struggle with minute variations, quantum sensors establish baselines that are influenced by the nuances of quantum behavior.
Applications include:
- Quantum Magnetometers: Measure magnetic fields at a sensitivity level unattainable by classical methods.
- Quantum Gravimeters: Gauge gravitational pulls with impressive accuracy, aiding in geological surveys and resource exploration.
- Quantum Imaging Devices: Enable high-resolution images by exploiting quantum phenomena, relevant in fields like medical imaging.
By placing themselves at the crossroads of cutting-edge research and practical implementation, quantum sensors stand as a testament to how modern science can redefine our understanding of measurement, driving forward both innovation and industry.
How Quantum Sensors Work
Understanding how quantum sensors work is pivotal to comprehending the broader implications of these technologies in our everyday lives and scientific advancements. Quantum sensors offer a stark departure from classical measurement tools, enhancing capabilities in terms of sensitivity and precision. Their operation is rooted in the principles of quantum mechanics and hinges on the behavior of subatomic particles. They unlock unique measurement possibilities in diverse fields, ranging from environmental monitoring to medical diagnostics.
Key Principles of Quantum Measurement
The core of quantum measurement lies in its ability to probe the fundamental properties of quantum states. These states behave distinctly, allowing quantum sensors to tap into phenomena that conventional sensors cannot.
- Superposition: Quantum particles can exist in multiple states simultaneously, enabling sensors to gather data from various conditions all at once. This characteristic greatly increases the efficiency of measurements.
- Entanglement: This principle allows particles to become interconnected such that the state of one instantly influences the state of another, regardless of distance. This feature is exploited in advanced sensors to achieve unparalleled precision and sensitivity.
- Non-locality: The implications of entanglement extend beyond mere connectivity. Measurements on one part of an entangled system can yield insights about another, all without direct interaction. This can enhance data gathering in distributed quantum sensing networks.
Ultimately, these principles grant quantum sensors their enhanced capabilities, making them a cutting-edge alternative in measurement technology.
Quantum States and Their Role in Sensing
The role of quantum states in sensing cannot be overstated. Each quantum sensor relies on specific types of quantum states to make measurements, which are manipulated in ways that classical sensors cannot achieve.
- Coherent States: Often linked with lasers, these states maintain a fixed phase relationship between particles, yielding precise measurement capabilities. They are vital in applications where phase stability is crucial, such as in optical sensing.
- Squeezed States: These states reduce uncertainty in one measurement at the cost of increasing uncertainty in another. This trade-off can lead to significant improvements in measurement precision, especially in gravitational wave detection, where noise is a persistent challenge.
- Fock States: Using discrete numbers of particles, Fock states can be utilized in quantum communication and cryptography, proving essential in secure data transmission practices.
By manipulating these states, researchers refine measurements, enabling sensors to provide superior results in applications that require fine-tuning.
Decoherence and Its Impact on Measurements
Decoherence describes the loss of quantum coherence, a critical phenomenon that affects measurement in quantum sensors. When a quantum system interacts with its environment, it can lose its unique quantum state, reverting to classical behaviors and thus diminishing its sensitivity. The impact of decoherence can manifest in several ways:
- Loss of Information: As coherence is lost, the quantum state can become entangled with environmental noise, leading to loss of critical information during measurement processes.
- Reduced Precision: Measurement precision can decrease significantly as uncertainty in quantum states grows due to environmental factors.
- Shortened Lifetimes: The effectiveness of quantum sensors that rely on sustained states decreases as decoherence times shorten, creating challenges in maintaining useful readings in real-world applications.
"Decoherence represents a fundamental barrier that must be overcome for quantum sensors to reach their full potential in practical environments."
To surmount these challenges, a range of techniques is supplied, including error correction codes and cooling mechanisms. Understanding and mitigating decoherence is essential for maintaining the fidelity of quantum sensor measurements.
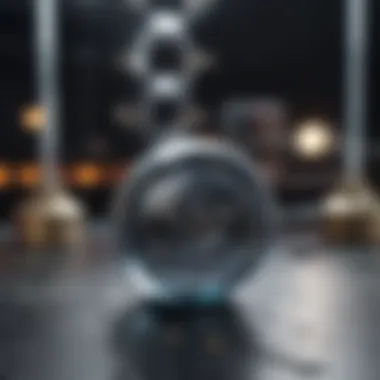
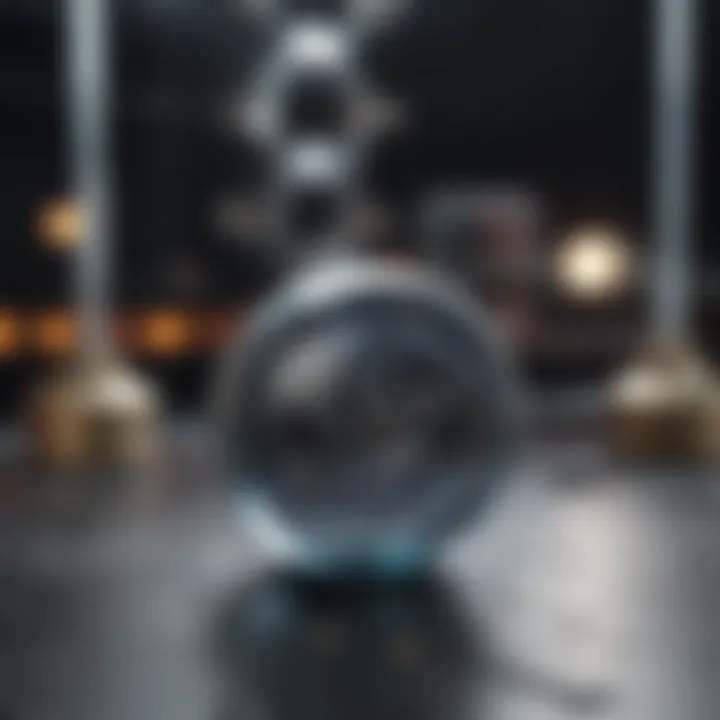
Types of Quantum Sensors
Quantum sensors represent a frontier in measurement technology, offering a range of devices that harness unique properties of quantum mechanics. They push the boundaries of sensitivity and precision, leading to advancements in multiple fields. Understanding the various types of quantum sensors is crucial as they each have distinctive capabilities and applications. This section explores the different categories, emphasizing their significance and real-world implications.
Atom Interferometers
Atom interferometers are cutting-edge instruments that leverage the wave-like nature of atoms to measure tiny changes in motion or gravitational fields. These sensors operate by splitting a beam of atoms into two separate paths, then recombining the beams to observe interference patterns. The precision of atom interferometers exceeds that of conventional sensors.
The benefits of atom interferometers include:
- Ultra-high sensitivity: Capable of detecting minute changes, these instruments could monitor gravitational waves or detect shifts in Earth’s gravity with remarkable accuracy.
- Versatility: Applications are vast, from geophysical surveying to fundamental physics research.
"Atom interferometry has opened new avenues in measurement, providing insights that traditional methods simply can't achieve."
These sensors are not just theoretical constructs; they’re actively being employed in ongoing research. For instance, they can be instrumental in searching for dark matter by detecting gravitational anomalies.
Quantum Magnetometers
Quantum magnetometers capitalize on quantum entanglement to achieve exceptional sensitivity to magnetic fields. Utilizing atoms or ions, these sensors can measure extremely weak magnetic signals that would otherwise be undetectable. A celebrated example is the use of optically pumped magnetometers in medical applications.
Their significance lies in:
- Improved imaging techniques: These devices enhance the resolution of magnetic resonance imaging (MRI).
- Environmental monitoring: Quantum magnetometers are valuable in assessing geological formations, enabling the identification of mineral deposits.
The operational principle centers around the concept of superposition, allowing for measurements that are far superior to classical counterparts. By manipulating quantum states, they provide insights into a range of phenomena, including biomagnetic fields produced by the human heart and brain.
Quantum Gravimeters
Quantum gravimeters represent another state-of-the-art technology by measuring gravitational acceleration with unmatched accuracy. These devices typically utilize matter-wave interferometry, allowing them to sense gravity variations caused by changes in mass distribution within the Earth.
Some key attributes include:
- High sensitivity: Capable of detecting variations at the level of microgals, making them essential for Geosciences.
- Portable designs: Recent advancements have enabled the miniaturization of quantum gravimeters, making them feasible for field use in diverse settings.
The applications are extensive, from natural disaster assessment to military operations, allowing for the monitoring of underground structures like tunnels or caves. The implications of their use in climate change studies are also profound, as they contribute vital information about ice cap melting and sea level rise.
Optomechanical Sensors
Optomechanical sensors exploit the interaction between light and mechanical vibrations. By analyzing the displacement of mirrors and other optical elements, these sensors can measure forces, pressure variations, and displacements at unprecedented levels of precision.
Such sensors boast the following benefits:
- Precision engineering: They are valuable in detecting gravitational waves, playing a pivotal role in the field of astrophysics.
- Advanced applications: Used in telecommunications and fiber optics, they improve the performance of optical networks.
The fundamental operating principle is grounded in the quantum behavior of light and motion, where information about tiny changes in the environment can be inferred from optical measurements. As these technologies evolve, they bring promising advancements in both industrial and research applications.
Applications of Quantum Sensors
Quantum sensors represent a significant leap in measurement technology, impacting various sectors in profound ways. With their ability to measure physical quantities with exceptional precision, quantum sensors are making waves in fields ranging from environmental monitoring to medical diagnostics. The applications of these sensors underscore not only their versatility but also their integral role in advancing science and technology.
Environmental Monitoring
One of the standout uses of quantum sensors lies in environmental monitoring. These devices can track minute changes in environmental conditions, such as fluctuations in temperature, pressure, and even electromagnetic fields. For instance, quantum magnetometers can detect subtle variations in magnetic fields that may indicate geological changes or the presence of pollutants.
This capability is crucial for understanding climate change effects and implementing effective conservation strategies. By enabling real-time monitoring of environmental conditions, these sensors can assist in early detection of natural disasters or serve as a part of ecosystem health assessments. The data gathered can lead to better decision-making processes at both local and global levels.
Medical Diagnostics
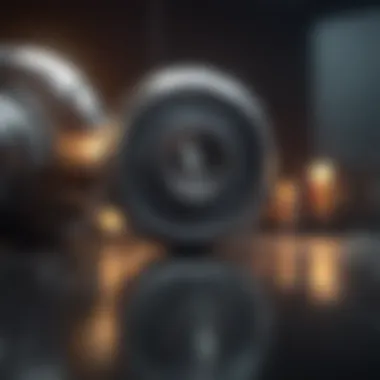
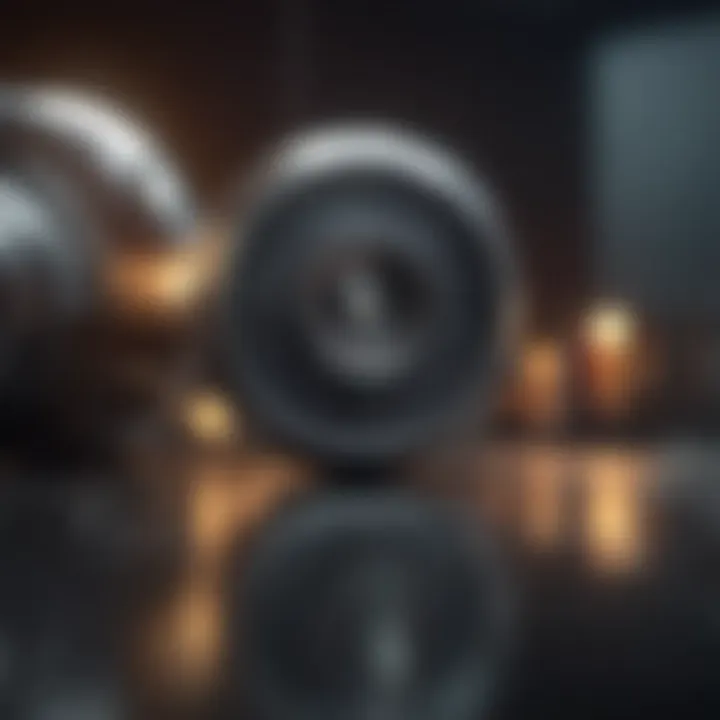
The healthcare sector is also benefitting immensely from the applications of quantum sensors. In medical diagnostics, these sensors can detect biological markers with unprecedented sensitivity. For example, using quantum-enhanced imaging techniques, clinicians can identify tumors much earlier than conventional methods allow.
Moreover, quantum sensors improve imaging technologies like MRI by enhancing image resolution and reducing scan times. The implications are far-reaching, as they not only facilitate earlier diagnoses but also pave the way for personalized medicine practices.
Fundamental Physics Research
In the realm of fundamental physics, quantum sensors are pivotal. They provide insights into basic laws of nature and facilitate experimentation at scales previously thought impossible. For example, quantum gravimeters can measure gravitational forces with extraordinary precision, opening pathways to deeper understanding within cosmology and general relativity.
These tools not only challenge existing theories but also encourage new approaches to understanding the universe's behavior. As researchers continue to explore how matter interacts at quantum levels, sensors will be indispensable in uncovering nature’s intricacies.
Navigation and Earth Observation
Lastly, quantum sensors enhance navigation and earth observation technologies significantly. Devices like atom interferometers are used for high-precision inertial navigation systems. Unlike traditional GPS, which can falter in signal loss scenarios, quantum sensors provide stable references based on quantum properties that remain effective regardless of location.
In earth observation, these sensors can map terrain and analyze changes on a micro-scale. This information is vital in various applications, including urban planning, agricultural monitoring, and resource management.
"The application of quantum sensors in navigation represents a game-changer, providing reliability where other systems might fail."
Advantages of Quantum Sensors
Quantum sensors are a breakthrough in the measurement landscape, providing unique advantages over classical sensor technologies. As we dive into the benefits of quantum sensing, it's clear that the implications reach across a variety of fields, shaping how we perceive and interpret the natural world. The importance of these sensors stems from their ability to combine theoretical frameworks from quantum mechanics with practical applications, leading to transformative impacts on technology and research.
Enhanced Sensitivity
One of the cornerstone features of quantum sensors is their enhanced sensitivity. Traditional sensors, while effective, often struggle to detect subtle changes in their environment. Quantum sensors, however, leverage quantum superposition, allowing them to pick up even the faintest signals that other devices might miss. For instance, quantum magnetometers can detect minute magnetic fields, which could be crucial for applications ranging from geological exploration to medical diagnostics.
"In the world of quantum sensing, sensitivity is not just a number; it's a paradigm shift in measurement."
This increased sensitivity opens doors to a multitude of uses. In medical diagnostics, for example, early-stage cancer detection significantly relies on advanced sensitivity levels. If sensors can identify biomagnetic signals related to tumor growth, they could be invaluable for developing non-invasive diagnostic techniques.
Increased Precision
Next up is precision, another significant advantage that cannot be understated. Quantum sensors are not only sensitive but also boast a level of precision that surpasses that of classical apparatus. This is possible thanks to the unique characteristics of quantum states, which allow for remarkably accurate measurements of physical quantities. An example can be found in quantum gravimeters, used for measuring minute variations in gravity, which can inform everything from underground resource exploration to the monitoring of tectonic activity.
This precision enables scientists to conduct experiments and gather data that were previously thought to be unattainable. In fundamental physics research, for instance, this capability helps unravel the mysteries of gravitation and potentially opens paths to discovering new physics beyond the Standard Model.
Miniaturization Potential
Finally, let's talk about miniaturization potential. Quantum sensors have a remarkable ability to condense complex sensing mechanisms into small, portable devices. This is a game-changer, particularly in the context of field applications. Smaller sensors mean that vital data can be collected in places previously deemed impractical, such as remote locations or even outer space.
The design of a quantum optical sensor can be reduced significantly while maintaining its performance levels. Such advancements allow researchers and professionals to deploy quantum sensors in practical and diverse settings. For example, a compact quantum device could monitor environmental parameters in real-time, aiding conservation efforts around the world.
In summary, the advantages of quantum sensors - enhanced sensitivity, increased precision, and miniaturization potential - represent a substantial leap forward in measurement technology. These benefits not only offer new avenues for research but also pave the way for groundbreaking applications across various disciplines, including medical science, environmental monitoring, and fundamental physics.
Challenges in Quantum Sensing
As we venture into the sophisticated realm of quantum sensors, it becomes essential to acknowledge the hurdles facing this burgeoning field. Understanding the impediments is critical, not just for researchers and engineers, but for anyone invested in the future of measurement technology. This section examines three primary challenges: technical limitations, scalability, and cost considerations. Each aspect holds considerable weight in shaping the advancement and widespread adoption of quantum sensing technologies.
Technical Limitations
One cannot help but notice the inherent technical limitations that quantum sensors grapple with. While these devices boast extraordinary sensitivity, they are not without quirks that can hinder performance. For example, the phenomenon of decoherence can obliterate the fragile quantum states that sensors rely on. This lack of stability might lead to inaccuracies in readings – essentially making the sensors less reliable than one would hope.
Additionally, many quantum sensors operate at extreme conditions, such as ultra-low temperatures, which pose challenges in practicality and integration into existing systems. This creates a barrier where technology may shine in a research setting but falls flat in real-world applications. The difficulty in maintaining these specialized environments complicates matters for commercial entities wishing to harness quantum sensors for everyday use.
Scalability Issues
Scalability stands out as another formidable challenge when it comes to quantum sensors. Presently, many of these sensors are effectively bespoke devices, fine-tuned for specific applications rather than mass-produced for general use. The delicate nature and intricate calibration of quantum sensors make it a tough nut to crack if one tries to ramp up manufacturing processes.
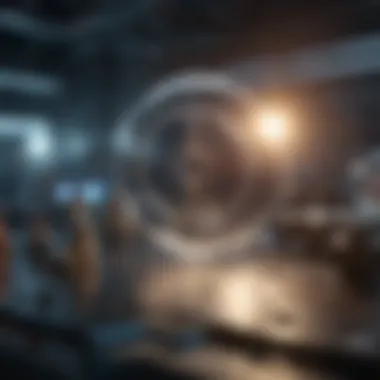
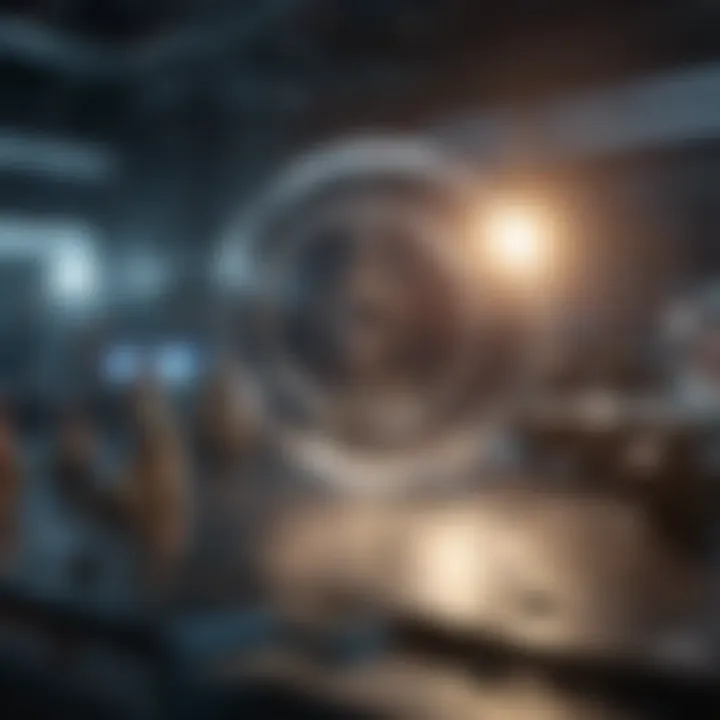
Moreover, as we consider potential applications in fields like environmental monitoring or medical diagnostics, the ability to scale these technologies is crucial. There lies a critical need for adaptable devices that can meet varying demands without losing too much in performance. As researchers explore innovative designs and materials, the path towards scalable quantum sensors remains elusive yet vital.
Cost Considerations
Ultimately, cost plays a pivotal role in the future of quantum sensors. The investment required to develop and produce these advanced devices is substantial. Whether for research institutions or companies, the financial outlay to bring a quantum sensor from concept to market can be daunting.
Some factors affecting the costs include:
- Research and Development Expenses: Ongoing R&D costs can easily balloon as scientists seek to enhance the technology’s viability.
- Material Costs: The specialized materials and components needed for quantum sensors aren't your run-of-the-mill supplies.
- Technical Expertise: Employing leading experts in quantum mechanics can drive up labor costs.
Given these challenges, it becomes clear that to realize the full potential of quantum sensing, there must be a concerted effort to address these blockers. Advances in material science, engineering, and financial models are essential to pushing this technology into a new era.
"Understanding these challenges isn't just about being cautious; it's about setting a roadmap for the future of quantum measurement technology."
Future Perspectives of Quantum Sensing
The field of quantum sensing is on a remarkable trajectory, with advancements that promise to redefine measurement technology. As scientists and engineers explore this uncharted territory, the implications of quantum sensors become increasingly multifaceted. They extend beyond mere novelty, influencing sectors such as healthcare, environmental sustainability, and navigational systems. Here, we will delve into some critical aspects of the future of quantum sensing.
Innovative Research Directions
The landscape of quantum sensing is poised for growth, with various research streams emerging. One promising direction is the development of novel materials that enhance sensor performance. For instance, researchers are investigating the utility of topological insulators, which could display unique electromagnetic properties that fundamentally change how we measure events at quantum levels.
Another intriguing area is the advancement of quantum metrology, where scientists work to improve measurement precision. This shift could lead to breakthroughs in timekeeping, positioning systems, and even communications technology. The integration of new quantum technologies, such as superconducting qubits, could also offer unprecedented calibration methods, leading to further enhanced reliability in measurements.
"Quantum sensing isn't just about improving sensitivity; it's about revolutionizing how we perceive information at a fundamental level."
Moreover, experimenting with entangled particles for sensing purposes has garnered attention. Entanglement can amplify measurement signals, allowing for the detection of phenomena that traditional sensors might overlook. This could truly extend our capabilities in areas like astrophysics, where understanding subatomic forces is crucial.
Interdisciplinary Collaborations
The future of quantum sensing is intrinsically linked to collaboration among various scientific disciplines. Physicists, engineers, biologists, and data scientists are increasingly recognizing that their collective expertise can lead to more innovative solutions.
For example, the intersection of biology and quantum technology can foster breakthroughs in medical diagnostics. By combining quantum sensors with biological analyses, researchers may detect diseases earlier and more accurately than current methods allow. Such interdisciplinary approaches pave the way for more integrative and holistic methodologies in research.
Universities and research institutions are establishing collaborative networks, encouraging experts from different fields to contribute their perspectives and knowledge. This blend of skills can stimulate creativity and foster environments where unconventional ideas thrive.
- Quantum sensors used in medical imaging can vastly improve accuracy.
- Collaborations might yield new environmental sensing devices to monitor pollution.
Integrating Quantum Sensors with AI
Artificial Intelligence stands at the forefront of technology’s evolution, and its integration with quantum sensors is a natural progression. The power of AI lies in its ability to process vast amounts of data quickly, identifying patterns that may not be apparent to human researchers.
By combining the precision of quantum sensors with AI algorithms, we can achieve enhanced data interpretation. Imagine a scenario where quantum sensors collect environmental data in real time, while an AI analyzes the trends and anomalies, alerting us to potential ecological crises before they escalate. This synergy could lead to timely interventions, significantly impacting areas like climate change and public health.
Furthermore, optimizing quantum sensor operations using AI can introduce efficiencies, like reducing noise in measurements and predicting system behaviors under different conditions. This will not only enhance existing sensor technologies but enable the creation of entirely new systems.
End
The journey through the world of quantum sensors reveals a landscape filled with unparalleled potential and innovation. These devices, built on the intricate foundations of quantum mechanics, hold the key to unlocking measurements with an accuracy that traditional technology could only dream of. By examining their operational principles and innovative applications, we can appreciate their value across various scientific fields.
Summary of Key Insights
Several insights stand out, emphasizing the vital role of quantum sensors:
- Precision Redefined: The sensitivity of quantum sensors often surpasses what we can achieve with conventional methods. Whether it's mapping gravitational variations for earth sciences or detecting minute magnetic fields in biological samples, quantum sensors enable more detailed explorations.
- Diverse Applications: The versatility of these sensors extends from environmental monitoring to medical diagnostics. Each field of application highlights the transformative potential that quantum technologies can bring to various domains.
- Future Potential: As research progresses and technology advances, the integration of quantum sensors with artificial intelligence offers new pathways for innovation. This interdisciplinary approach could provide solutions to problems previously considered unsolvable.
Overall, the significance of quantum sensors isn't just in the technology itself, but also in how it reshapes our understanding and interaction with the world.
The Expanding Role of Quantum Sensors
As we look ahead, the influence of quantum sensors is bound to grow exponentially. Their role continues to expand in ways that extend beyond traditional measurement capabilities.
- Driving Scientific Research: Quantum sensors are increasingly becoming pivotal in fundamental physics, helping researchers probe the mysteries of the universe at a level of detail never before attainable.
- Enhancing Everyday Technology: Beyond laboratories, the integration of these sensors into everyday tech could change the landscape of consumer electronics or smart devices, making them not only more effective but also more responsive to user needs.
- Global Challenges: In tackling issues like climate change, quantum sensors could play an instrumental role in environmental monitoring and resource management, enabling data collection that informs policy decisions and public awareness.
Consequently, as the landscape of technology evolves, quantum sensors stand at the frontier, promising to redefine what is possible in measurement and observation. The implications for science, health, and industry demand continued focus and investment, ensuring their contributions resonate across generations.